Abstract
Background:
The global threat of antimicrobial resistance is undoubtedly on the increase and most researches in this area have concentrated on effects of drug exposure and usage on resistance. Little attention has been given to natural environments apparently devoid of direct anthropogenic impact as possible reservoirs of drug resistance traits.Objectives:
This research was undertaken to determine possible similarities between drug resistance traits in species of Klebsiella and Citrobacter obtained from wild animals and 2 human groups.Methods:
Human samples were collected from Humans free from antibiotics (HN) and Human on antibiotics (HA) while samples from wildlife (WL) were taken from rats (Rattus spp), grasscutters (Thryonomys swinderianus), squirrels (Xerus erythropus), antelopes (Tragelephus scriptus), rabbits (Oryctolagus cuniculus), and farm lizards (Agama spp). Samples were analyzed using basic microbiological methods. The Disc diffusion technique was used for drug sensitivity testing while plasmid analysis was based on gel electrophoresis.Results:
Members of the genus Klebsiella isolated from HA exhibited more resistance to ampicillin (AMP) and augmentin (AUG) than those isolated from HN. However, Klebsiella isolated from HN displayed higher resistance to ceftriaxone (CTN), clarithromycin (CMN), ofloxacin (OFL), and pefloxacin (PEF) than those from HA. WL isolates were mostly resistant to AMP, CMN, and AUG, and less resistant to PEF, CTN, and ciprofloxacin. Correlation analysis of the antimicrobial resistance pattern on a 2 tailed test P ≤ 0.01 and P ≤ 0.05, revealed a high correlation (ranging from 0.715 to 0.917) among all the microorganisms from both sources. Gel electrophoretic analysis of plasmid DNA extracted from the isolates revealed the presence of a 23.1 kb plasmid DNA in 6 strains of Citrobacter and 3 strains of Klebsiella.Conclusions:
These results indicate that wild life may be important reservoirs of drug resistance genes and pathogens that have public health relevance.Keywords
Humans Wild Life Drug Resistance Plasmids Klebsiella Citrobacter
1. Background
The increasing resistance of bacteria to antibacterial agents is a continuing global public health threat (1). Recently, the world health organization released a list of drug resistant bacteria, among them, members of the Enterobacteriacae, which are considered the greatest threat to human health (2). The factors driving antimicrobial drug resistance among infectious agents remain vague (3), with many studies on the risk factors often non-conclusive (4). However, the development of resistance inevitably follows the introduction of a new antibiotic. Usage of antibiotics is therefore a risk factor in the development of antibiotic resistance (5).
The rate at which antibiotic resistance develops is related to the total consumption of antibiotics, regardless of whether appropriately used or not. The startling period of antibiotic resistance emergence began after the introduction of industrially produced antibiotics. This created a correlation between antibiotic pressure and emergence of resistance. In addition to this, however, antibiotic-resistant bacteria have been found in hosts and environments apparently free from any antibiotic pressure imposed by the activities of man (6, 7). Most researches on antibiotic resistance dissemination have focused on human and veterinary medicine, ignoring the need to understand how bacterial resistance is disseminated within reservoirs in natural environments devoid of anthropogenic impact (8).
2. Objectives
Although controversy continues to surround the impact of natural reservoirs (9), many serious and life threatening human diseases seem to have been the result of increasingly close and frequent contacts with a new array of zoonotic potential pathogens. It is therefore important to study the similarities between drug resistance traits in some microorganisms inhabiting diverse human and wild life sources. This is the central theme of this work with the following objectives:
- To compare the resistance profile of Enterobacteriaceae isolates from human and animal sources.
- To compare the resistance profile of isolates from humans on antibiotics and those not on antibiotics vis a vis isolates from wild life.
- To isolate and compare the resistance plasmids of isolates from the above mentioned sources.
3. Methods
3.1. Ethics Statement
All samples were collected using aseptic methods and in conformity with the ethical guidelines of the 1975 declaration of Helsinki.
3.2. Sample Collection and Bacterial Identification
This study was conducted on human population between the ages of 10-45 and above, and Wild animals: rats (Rattus spp), grasscutters (Thryonomys swinderianus), squirrels (Xerus erythropus) antelopes (Tragelephus scriptus), rabbits (Oryctolagus cuniculus), and farm lizards (Agama spp). Stool samples were taken from humans while the test animals were necrospied. The human clinical samples were collected from Bishop Shanahan Hospital, Nsukka, Nigeria after informed consents were obtained from both the hospital administration and the patients. Wild life (WL) samples were collected from Okutu, Okpuje, Ede-Oballa, Allor uno, Opi, Opiagu, and Obollo communities in old Nsukka Division of Enugu state, Nigeria. Human samples were obtained from 2 human groups, designated as Human not on antibiotics (HN) and Human on antibiotics (HA). HN represented groups who had not used antibiotics for 3 months prior to sample collection while HA represented groups that had been on antibiotics therapy within the 3 months.
Samples were inoculated onto nutrient and MacConkey agar plates and incubated for 24 hours at 37°C. Colonies obtained were isolated and purified. Colonies obtained from MacConkey agar were designated as either lactose fermenters or non-lactose fermenters based on the pigmentation. All isolates were Gram stained and examined microscopically. Biochemical tests were done based on the Gram reactions and in accordance with the manual for general bacteriology of the American society of microbiology (1981) (10). Among the tests carried out were sugar fermentation (glucose, lactose, sucrose, and mannitol), Voges-Proscauer (VP), catalase, indole, oxidase, hydrogen sulfide (H2S) production, methyl red, and motility tests. CHROMagar ™ (orientation) and API 20E (bioMerieux, France) were used to confirm the identity of the isolates.
3.3. Susceptibility Testing
Resistance to commonly used antibiotics was determined using the Kirby-Bauer disc diffusion test (11). Antibiotics tested include ampicillin, augmentin, chloramphenicol, clarithomycin, cetriaxone, nitrofurantoin, gentamycin, ofloxacin, ciprofloxacin, and peflacine.
3.4. Plasmid Isolation and Electrophoresis
Strains selected based on antibiotic resistance and provenance were subjected to plasmid isolation and agarose gel electrophoresis according to the methods of Kado and Liu (1981) (12) recently used by Akter et al. (2011) (13). Each selected colony was transferred into a conical flask containing nutrient broth and incubated at 37°C overnight with shaking (180 x g). An aliquot (1 mL) of the culture was taken and centrifuged (16,000 x g) for 30 seconds at 4oC. The supernatant was removed and the pellet was re-suspended in 150 µL of Tris-EDTA buffer [10 mM Tris chloride (pH 8), 1 Mm EDTA (pH 8)] solution by vigorous vortexing. Subsequently, 200 µL of NaOH-SDS (0.2M NaOH, 1% SDS) solution and 150 µL of 3M potassium acetate (pH 4.8) were added and the mixture vortexed for 10 seconds. The content was centrifuged (16,000 x g) for 5 minutes at 4°C. The supernatant was precipitated with 600 µL of ice cold 100% ethanol. A portion (15 µL) of plasmid DNA was loaded onto a 0.8% agarose gel containing 0.5 µg mL-1 ethidium bromide and electrophoresed in TBE (Tris-Boric acid –EDTA) buffer, all in Sigma-Aldrich Horizontal Electrophoresis Unit (Mini Z33,879-6). Lambda- Hind ΠΙ digested DNA ladder (Ambion) was used as standard size marker. The plasmid DNAs were visualized and photographed by placing the gel on a UV (300 nm) trans illuminator. Protective goggles were used.
3.5. Statistical Analysis
Bacterial isolates were grouped according to human and wildlife provenance. Analysis of Variance and Pearson Correlation tests were carried out using the Statistical Package for Social Sciences (SPSS 16.0) Inc (444N Michigan USA).
4. Results
Citrobacter and Klebsiella spp. were isolated from the sources considered in the study with Citrobacter spp being the more frequently isolated organisms in the WL samples. Members of the genus Klebsiella isolated from HA exhibited more resistance to ampicillin and augmentin than those isolated from HN. However, Klebsiella isolated from HN showed a greater resistance to cetriaxone, clarithomycin, ofloxacin, and pefloxacin. This is shown in Figure 1.
Antibiotic Resistance Pattern of Klebsiella spp Isolated from the Two Human Groups
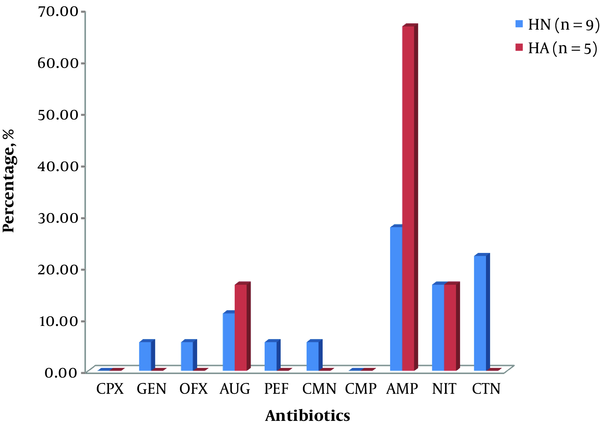
Similarly, Citrobacter spp. isolated from HA showed appreciable resistance to ampicillin (39.29%), augmentin (28.57%), chloramphenicol (10.71%), and clarithomycin (10.71%). Furthermore, members of the genus from HN showed resistance to ampicillin (25%), augmentin (18.75%), cetriaxone (18.75), and nitrofurantoin (12.50) in that order. This is shown in Figure 2.
Antibiotic Resistance Pattern of Citrobacter Isolated from the Two Human Groups
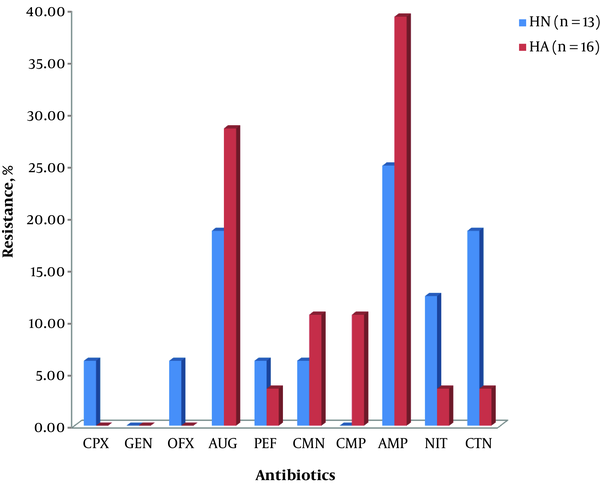
The antimicrobial resistance pattern exhibited by the test organisms was further analyzed using the Pearson correlation. The correlates were examined using a 2-tailed test (P ≤ 0.01; and P ≤ 0.05). From the analyses, there was a strong direct relationship between the resistance patterns of the Klebsiella spp (0.715) isolated from the 2 human sources. Similarly, Citrobacter from HN showed a strong positive correlation with Klebsiella from HN source (0.917). The lowest correlates (0.653) existed between Citrobacter from HA and Citrobacter from HN. Overall, there exists a high correlation of resistance pattern among all the microorganisms isolated from both sources. This information is presented in Table 1.
Correlation Matrix of Antibiotics Resistance Between Citrobacter and Klebsiella Isolated from the Two Human Sources (HA and HN)
The resistance pattern exhibited by isolates from WL against the test antibiotics is summarized in Table 2. The microorganisms were most resistant to AMP, CMN, and AUG, and least resistant to PEF, GEN, OFX, CTN, and CPX. Species of Klebsiella isolated from wild life exhibited varying degrees of susceptibility to the test antibiotics, least of all against AMP to which 81.82% of the organisms were resistant. However, those isolated from human sources showed a lower percentage resistance to AMP (47.22%) but a higher percentage to NIT (16.67%), AUG (13.89%), and CTN (11.11%). This information is presented in Figure 3.
Percentage Distribution of Antibiotic Resistance Among Citrobacter and Klebsiella spp from Wild Life
S/N | Antibiotics | Klebsiella spp., % | Citrobacter spp., % |
---|---|---|---|
WL (n = 11) | WL (n = 31) | ||
1 | CPX | 0.00 | 0.00 |
2 | GEN | 0.00 | 0.00 |
3 | OFX | 0.00 | 0.00 |
4 | AUG | 9.09 | 9.30 |
5 | PEF | 0.00 | 0.00 |
6 | CMN | 9.09 | 18.60 |
7 | CMP | 0.00 | 18.60 |
8 | AMP | 81.82 | 51.16 |
9 | NIT | 0.00 | 2.33 |
10 | CTN | 0.00 | 0.00 |
Antibiotic Resistance Pattern of Klebsiella spp Isolated from Human Sources and Wildlife
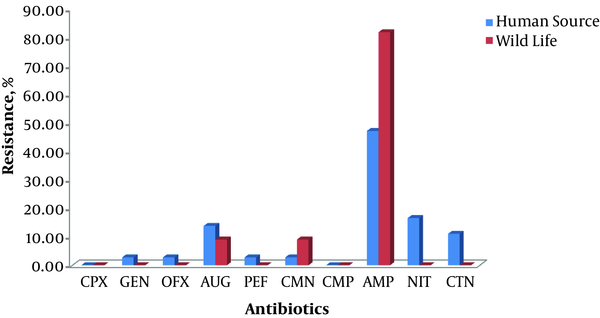
Wildlife isolates of Citrobacter generally demonstrated resistance to AMP (51.16%), CMP (18.60%), CMN (18.60%), AUG (9.30%) and NIT (2.33).Similarly, those isolated from human sources were resistant to the antibiotics except GEN (0.00%). The percentage resistance of Citrobacter isolates from the general population is shown in Figure 4.
Antibiotic Resistance Pattern of Citrobacter Isolated from Human and Wildlife Sources
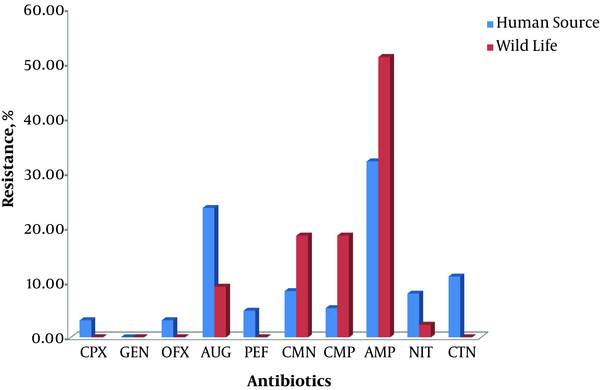
In order to determine the relationship among the enterics in respect of their antimicrobial resistance pattern, a correlation analysis was done. The correlates analyzed the relationship between isolates from wildlife (WL) and humans on antibiotics (HA), as well as those from wild life (WL) and humans not on antibiotics (HN). The correlation matrices were determined at P ≤ 0.05 and presented in Tables 3 and 4 below. High correlation coefficient was observed among the different genera of bacteria isolated from one source to those from different sources. For example, the correlation between HA and WL revealed a correlate of 0.945 between Klebsiella (HA) and Klebsiella (WL). Similarly, a correlation coefficient of 0.942 existed between Citrobacter (WL) and Klebsiella (WL) (Table 3). However, a weak direct linear correlation of 0.499 was observed between Citrobacter (HN) and Citrobacter (WL) (Table 4).
Correlation Matrix of Antibiotics Resistance among Enterics Isolated from HA and WL
Correlation Matrix of Antibiotics Resistance among Enterics Isolated from HN and WL
4.1. Plasmid DNA Profiling and Distribution among the Isolated Organisms
The electrophoretic separation technique showed the presence of resistance plasmid DNAs in some of the isolates, both drug resistant and susceptible ones. The molecular weight of the plasmids revolved around 23.1 kb. For instance plasmid DNA was isolated from 6 strains of Citrobacter and 3 strains of Klebsiella. The distribution of the plasmids is shown in Table 5 and Figures 5 and 6.
Distribution of Plasmid DNA from Test Isolates
Bacteria Isolate | Number of Isolates Tested | Number Isolates with Plasmid | Antibiotic Profile | Mol wt. of Plasmid, kb |
---|---|---|---|---|
Citrobacter | 13 | 6 | MDR | 23.1 |
Klebsiella | 10 | 3 | MDR & MDS | 23.1 |
AGE of Plasmid DNA from MDR and MDS Citrobacter
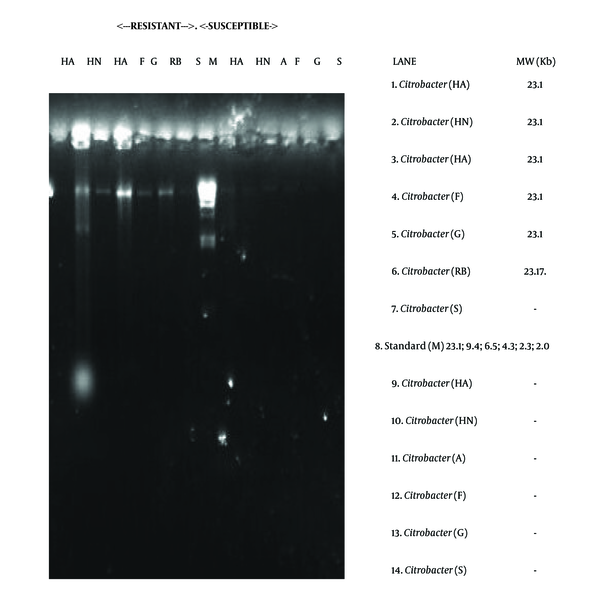
AGE of Plasmid DNA from MDR and MDS Klebsiella spp
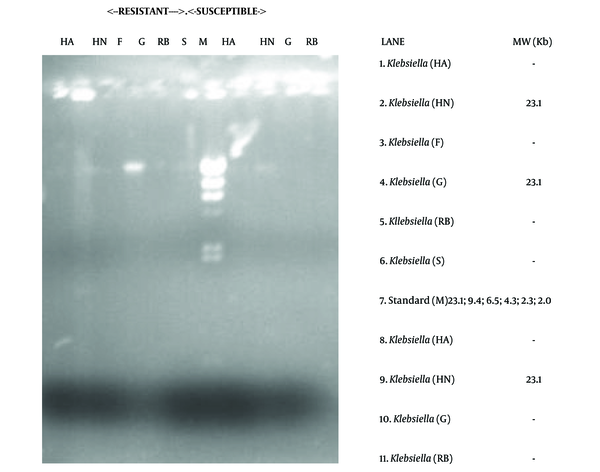
5. Discussion
The isolation of Klebsiella and Citrobacter, members of the Enterobacteriaceae, from the sample sources attests to their ubiquity and makes their response to antibacterial agents relevant in public health. These organisms have been isolated from different animate (14) and inanimate sources including sewage, refuse, sheds, and animal skins (15-17). In addition, they have been isolated from different parts of the human body especially the gastrointestinal tracts (18). In some cases, they may be found in the blood system (where they cause blood sepsis), urinary, and genital tracts causing varying degrees of urinogenital disorders (19, 20). As members of the Enterobacteriaceae, they are considered major public health challenges (2, 21).
The antibiotic resistance pattern of Citrobacter and Klebsiella spp. observed in this study revealed no significant difference between organisms isolated from HA and HN. This suggests first, the possibility of organism spillover between these 2 human groups and secondly, the independence of antibiotic resistance on the organisms’ immediate anthropogenic environment. This is in line with the concern raised by Rose et al. (2009) (22) and Levy (2010) (23) where drug resistant microorganisms move among people and animals, from one country to another without notice. The import of this is that the persistence and spread of antibiotic resistant bacteria in the community increases the pool of drug resistance traits. This is the condition previously known only in the traditional antibiotic-resistance hot spots of hospitals and nursing homes where close physical contact and the presence of susceptible hosts are believed to contribute to the spread of resistant bacteria.
It was observed that most of the isolated organisms were susceptible to fluroquinolone antibiotics. This is in line with reports by Malik et al. (2006) (24) who demonstrated that fluoroquinolones have improved pharmacokinetic and pharmacodynamic properties, and are therefore more efficacious than other antibiotics. A further comparison of the resistance pattern of isolates from humans on antibiotic therapy and humans not on therapy showed that resistance to certain drugs may not necessarily be a consequence of previous exposure to the antibiotics. High correlates of > 0.5 signifies that other factors such as the discharge of antibiotic contaminated wastewater effluents (25, 26) among others may be responsible for the resistance exhibited against the antibiotics (Table 1). This is in line with previous researches that have adduced the presence of resistance to several factors including, but not limited to, environmental factors (19, 27, 28), selective pressure of antibiotics (29), genetic compatibility of microorganisms (30), etc. Reportedly, whether or not microorganisms were previously exposed to antibiotics, their intrinsic ability to pick up genetic material from their environment (16) is an indication that resistance could be conferred on an organism through various means (31).
The statistical analysis of experimental findings revealed that antimicrobial resistance pattern of the organisms obtained from human and wild life correlate significantly (Tables 3 and 4), although isolates from both sources displayed marked differences in resistance pattern against commonly used antibiotics (Figures 3 to 6). This finding is consistent with the reports of Van den Bogaard and Stobberingh (2000) (32), Eze (2012) (16) and Costa et al. (2013) (31) showing that microorganisms, regardless of their source, could be resistant to antibiotics. More importantly, it further shows systematic (rather than random) variation in the mode and mechanism of resistance (32).
The study further revealed that direct contact with antibiotics may not be the only factor contributing to and sustaining resistance. Although this stance appears debatable, some researchers have earlier shown that organisms are isolated from wildlife where there is remote chance of contact with antibiotics exhibit resistance to some test antibiotics. Multidrug-resistant bacteria have been discovered in wild birds (gulls, birds of prey) and mammals (wolves, foxes, rabbits, deer, otters) with no apparent exposure to antimicrobials (33, 34), thus, suggesting that resistance may develop among organisms in drug pressure free environments and that once developed, resistance may not be confined to the ecological niche where it primarily originated. Since none of the ‘wildlife’ used in this research had presumably received antibiotics, it can be inferred once again that plasmid bearing and drug resistant microorganisms can be found in areas where there is no sustainable pressure for their maintenance. According to Costa et al. (2013) (31), 2 hypothesis may justify the presence of these MDR strains of bacteria in wildlife: (i) colonization of the wildlife’s gut with resistant strains directly from the environment or harboured by their prey or, (ii) sharing of transferable genetic elements that code for resistance between “ingested” strains and the native enteric flora of wild animals. The latter takes place preferably between bacteria with the highest phylogenetic proximity, however, it may also occur between different genera and species (31).
The possibility of antibiotic resistance mediation by genetic element was examined using plasmid profiling and electrophoretic separation of genetic components. The study revealed the occurrence of 23.1 kb plasmid DNAs in Citrobacter and Klebsiella (Table 5; Figures 5 and 6). This occurrence of 23.1 kb plasmid in these organisms is in agreement with the research reports of Dillon and Yeung (1989) (35), Fortin et al. (1993) (36), Van den Bogaard and Stobberingh (2000) (37), Sharif and Astal (2004) (38), as well as Eze (2012) (16). This 23.1 kb plasmid has been reported to be perhaps, one of the most frequently occurring plasmids in microbes known for mediating ESBL occurrence and multiple drug resistance (38).
6. Conclusion
The foregoing shows that wildlife may act as important environmental bio-indicators, reservoirs of medically important pathogens, and resistance genes such as those found among bacteria from humans on antibiotic therapy as well as those outside the hospital environment. They may be counted among the potential melting pots for the development and sustenance of resistance traits.
References
-
1.
Schechner V, Temkin E, Harbarth S, Carmeli Y, Schwaber MJ. Epidemiological interpretation of studies examining the effect of antibiotic usage on resistance. Clin Microbiol Rev. 2013;26(2):289-307. [PubMed ID: 23554418]. https://doi.org/10.1128/CMR.00001-13.
-
2.
Willyard C. The drug-resistant bacteria that pose the greatest health threats. Nature. 2017;543(7643):15. [PubMed ID: 28252092]. https://doi.org/10.1038/nature.2017.21550.
-
3.
Afema JA, Byarugaba DK, Shah DH, Atukwase E, Nambi M, Sischo WM. Potential Sources and Transmission of Salmonella and Antimicrobial Resistance in Kampala, Uganda. PLoS One. 2016;11(3). e0152130. [PubMed ID: 26999788]. https://doi.org/10.1371/journal.pone.0152130.
-
4.
Castillo-Tokumori F, Irey-Salgado C, Malaga G. Worrisome high frequency of extended-spectrum beta-lactamase-producing Escherichia coli in community-acquired urinary tract infections: a case-control study. Int J Infect Dis. 2017;55:16-9. [PubMed ID: 27979787]. https://doi.org/10.1016/j.ijid.2016.12.007.
-
5.
Livermore DM. beta-Lactamases in laboratory and clinical resistance. Clin Microbiol Rev. 1995;8(4):557-84. [PubMed ID: 8665470].
-
6.
Gilliver MA, Bennett M, Begon M, Hazel SM, Hart CA. Antibiotic resistance found in wild rodents. Nature. 1999;401(6750):233-4. [PubMed ID: 10499578]. https://doi.org/10.1038/45724.
-
7.
Souza V, Rocha M, Valera A, Eguiarte LE. Genetic structure of natural populations of Escherichia coli in wild hosts on different continents. Appl Environ Microbiol. 1999;65(8):3373-85. [PubMed ID: 10427022].
-
8.
Donato JJ, Moe LA, Converse BJ, Smart KD, Berklein FC, McManus PS, et al. Metagenomic analysis of apple orchard soil reveals antibiotic resistance genes encoding predicted bifunctional proteins. Appl Environ Microbiol. 2010;76(13):4396-401. [PubMed ID: 20453147]. https://doi.org/10.1128/AEM.01763-09.
-
9.
Pearce-Duvet JM. The origin of human pathogens: evaluating the role of agriculture and domestic animals in the evolution of human disease. Biol Rev Camb Philos Soc. 2006;81(3):369-82. [PubMed ID: 16672105]. https://doi.org/10.1017/S1464793106007020.
-
10.
American Society of Microbiology. Manual for General Bacteriology. 1981. Available from: http://ourfood.com/general-_bacteriology.html.
-
11.
Balouiri M, Sadiki M, Ibnsouda SK. Methods for in vitro evaluating antimicrobial activity: A review. J Pharm Analysis. 2016;6(2):71-9. https://doi.org/10.1016/j.jpha.2015.11.005.
-
12.
Kado CI, Liu ST. Rapid procedure for detection and isolation of large and small plasmids. J Bacteriol. 1981;145(3):1365-73. [PubMed ID: 7009583].
-
13.
Akter S, Rafiq-Un N, Rupa FA, Bari MDL, Hossain MAC. Antibiotic resistance and plasmid profiles in bacteria isolated from market-fresh vegetables. Agric Food Anal Bacteriol. 2011;1(2):140-9.
-
14.
Akinyemi KO, Oyefolu AOB, Salu OB, Adewale OA, Fasure AK. Bacterial Pathogens Associated with Tap and Well Waters in Lagos, Nigeria. East Central Afr J Surg. 2006;11(1):110-7.
-
15.
Akinyemi AA, Ajisafe MO. Occurrence of bacteria in the buccal cavity, gill and skin of Chrysichthys nigrodigitatus, Sardinella maderensis, and Mugil cephalus from Lagos lagoon Nigeria. Int. J. Biol. Chem. Sci. 2011 4;5(2):471-8.
-
16.
Eze EA. Systematic variations in drug resistance among some enteric gram-negative bacilli isolated from humans and sewage. J Microbiol Antimicrob. 2012;4(1):6-15. https://doi.org/10.5897/JMA11.031.
-
17.
Reuben CR, Gyar SD, Ashefo D, Tanimu H. Antimicrobial Resistance of Enterobacteria to Some Commonly used Antibiotics in General Hospital Akwanga, Nasarawa State, Nigeria. Int J Sci Res. 2013;2(2):227-81.
-
18.
Iroha IR, Adikwu MU, Esimone CO, Aibinu I, Amadi ES. Extended spectrum beta lactamase (ESBL) in E. coli isolated from a tertiary hospital in Enugu State, Nigeria. Pak J Med Sci. 2009;25(2):279-82.
-
19.
Aibinu I, Aednipekun E, Odugbemi T. Emergence of Quinolone Resistance amongst Escherichia coli strains isolated from Clinical infections in some Lagos State Hospitals, in Nigeria. Nigerian J Health Biomed Sci. 2004;3(2):73-8.
-
20.
Avgustin J. Animal production systems as a selective environment for antibiotic resistance genes. Acta Agriculturae Slovenica. 2012;1(1):7-17.
-
21.
Ny S, Lofmark S, Borjesson S, Englund S, Ringman M, Bergstrom J, et al. Community carriage of ESBL-producing Escherichia coli is associated with strains of low pathogenicity: a Swedish nationwide study. J Antimicrob Chemother. 2017;72(2):582-8. [PubMed ID: 27798205]. https://doi.org/10.1093/jac/dkw419.
-
22.
Rose JM, Gast RJ, Bogomolni A, Ellis JC, Lentell BJ, Touhey K, et al. Occurrence and patterns of antibiotic resistance in vertebrates off the Northeastern United States coast. FEMS Microbiol Ecol. 2009;67(3):421-31. [PubMed ID: 19187217]. https://doi.org/10.1111/j.1574-6941.2009.00648.x.
-
23.
Levy SB. Preface. In: Sosa AJ, Byarugaba DK, Amabile-Cuevas CF, Hsueh P, Kariuki S, Okeke IN, editors. Antimicrobial Resistance in Developing Countries. London: Springer; 2010.
-
24.
Malik S, Coombs GW, O'Brien FG, Peng H, Barton MD. Molecular typing of methicillin-resistant staphylococci isolated from cats and dogs. J Antimicrob Chemother. 2006;58(2):428-31. [PubMed ID: 16782740]. https://doi.org/10.1093/jac/dkl253.
-
25.
Iweriebor BC, Gaqavu S, Obi LC, Nwodo UU, Okoh AI. Antibiotic susceptibilities of enterococcus species isolated from hospital and domestic wastewater effluents in alice, eastern cape province of South Africa. Int J Environ Res Public Health. 2015;12(4):4231-46. [PubMed ID: 25893999]. https://doi.org/10.3390/ijerph120404231.
-
26.
Blaak H, Lynch G, Italiaander R, Hamidjaja RA, Schets FM, de Roda Husman AM. Multidrug-Resistant and Extended Spectrum Beta-Lactamase-Producing Escherichia coli in Dutch Surface Water and Wastewater. PLoS One. 2015;10(6). e0127752. [PubMed ID: 26030904]. https://doi.org/10.1371/journal.pone.0127752.
-
27.
Usui M, Tagaki C, Fukuda A, Okubo T, Boonla C, Suzuki S, et al. Use of Aeromonas spp. as General Indicators of Antimicrobial Susceptibility among Bacteria in Aquatic Environments in Thailand. Front Microbiol. 2016;7:710. [PubMed ID: 27433156]. https://doi.org/10.3389/fmicb.2016.00710.
-
28.
Wyrsch ER, Roy Chowdhury P, Chapman TA, Charles IG, Hammond JM, Djordjevic SP. Genomic Microbial Epidemiology Is Needed to Comprehend the Global Problem of Antibiotic Resistance and to Improve Pathogen Diagnosis. Front Microbiol. 2016;7:843. [PubMed ID: 27379026]. https://doi.org/10.3389/fmicb.2016.00843.
-
29.
Akinyemi KO, Bamiro BS, Coker AO. Salmonellosis in Lagos, Nigeria: incidence of Plasmodium falciparum-associated co-infection, patterns of antimicrobial resistance, and emergence of reduced susceptibility to fluoroquinolones. J Health Popul Nutr. 2007;25(3):351-8. [PubMed ID: 18330069].
-
30.
Byarugaba DK. Mechanisms of Antimicrobial Resistance. In: Sosa AJ, Byarugba DK, Amabile C, Hsueh PR, Karuiki S, Okeke IN, editors. Antimicrobial Resistance in Developing Countries. USA: Springer Science and Business Media; 2010. p. 15-26.
-
31.
da Costa PM, Loureiro L, Matos AJ. Transfer of multidrug-resistant bacteria between intermingled ecological niches: the interface between humans, animals and the environment. Int J Environ Res Public Health. 2013;10(1):278-94. [PubMed ID: 23343983]. https://doi.org/10.3390/ijerph10010278.
-
32.
Mach PA, Grimes DJ. R-plasmid transfer in a wastewater treatment plant. Appl Environ Microbiol. 1982;44(6):1395-403. [PubMed ID: 6760813].
-
33.
da Costa PM, Vaz-Pires P, Bernardo F. Antimicrobial resistance in Escherichia coli isolated in wastewater and sludge from poultry slaughterhouse wastewater plants. J Environ Health. 2008;70(7):40-5-53. [PubMed ID: 18348391].
-
34.
Sjolund M, Bonnedahl J, Hernandez J, Bengtsson S, Cederbrant G, Pinhassi J, et al. Dissemination of multidrug-resistant bacteria into the Arctic. Emerg Infect Dis. 2008;14(1):70-2. [PubMed ID: 18258081]. https://doi.org/10.3201/eid1401.070704.
-
35.
Dillon JA, Yeung KH. Beta-lactamase plasmids and chromosomally mediated antibiotic resistance in pathogenic Neisseria species. Clin Microbiol Rev. 1989;2 Suppl:S125-33. [PubMed ID: 2655882].
-
36.
Fortin C, Marquis C, Nester EW, Dion P. Dynamic structure of Agrobacterium tumefaciens Ti plasmids. J Bacteriol. 1993;175(15):4790-9. [PubMed ID: 8335635].
-
37.
Stobberingh E, van den Bogaard A, London N, Driessen C, Top J, Willems R. Enterococci with glycopeptide resistance in turkeys, turkey farmers, turkey slaughterers, and (sub)urban residents in the south of The Netherlands: evidence for transmission of vancomycin resistance from animals to humans? Antimicrob Agents Chemother. 1999;43(9):2215-21. [PubMed ID: 10471567].
-
38.
Sharif FA, Astal ZY. Role of plasmids in mediating antibiotic resistance and extended–spectrum beta–lactamase production in escherichia coli. IUG J Nat Stud. 2015;12(2).