Abstract
Background:
Arginine metabolism is an important factor involved in tumorigenesis, progression, and survival of tumor cells. Besides, other metabolites produced in the arginine metabolism process, such as polyamines, nitric oxide, argininosuccinate, and agmatine, play key roles in different stages of tumor development. On the other hand, herbal metabolites are widely used to treat cancer. One of these herbal flavonoids is quercetin.Methods:
In this study, according to MTT assay data, two concentrations of quercetin flavonoid were selected (57.5 and 115 µM) to treat human embryonic kidney 293 (HEK293) cells. Then RNA was extracted from the cells and used as a template for cDNA synthesis. Using real-time PCR, the expression of key enzymes involved in arginine metabolism was evaluated, including arginase 2 (Arg2), ornithine carbamoyl transferase (OTC), agmatinase (AGMAT), arginase 1 (Arg1), nitric oxide synthase 1 (nNOS), arginine decarboxylase (ADC), ornithine decarboxylase 1 (ODC), ornithine carbamoyl transferase (OCT), spermidine synthase (SRM), spermine synthase (SMS), argininosuccinate synthase 1 (ASS1), and argininosuccinate lyase (ASL). The Student t-test was used to analyze the data considering a P value of < 0.05 as the significance level.Results:
Our results indicated significant changes in the expression of arginine metabolism enzymes after quercetin exposure, confirming a role for quercetin plant flavonoid in regulating arginine metabolism in HEK293 cells.Conclusions:
Quercetin could alter the gene expression of the key enzymes involved in arginine metabolism. This was the first study investigating the effects of quercetin on arginine metabolism in HEK293 cells.Keywords
1. Background
Quercetin (3, 3, 4, 5, 7-pentahydroxy flavone) is a plant flavonoid that is found in the daily diet of millions of people around the world (1, 2). Tea, vegetables, and red wine are rich in quercetin (3, 4). Quercetin is a free-radical scavenger and can temporarily bind to metal ions (5). Also, it has the ability to cross the blood-brain-barrier (6). Several studies have shown that quercetin has beneficial effects on many diseases such as respiratory and cardiac disorders and viral infections (7-9). In addition, it has been shown that quercetin can inhibit the growth of cancer cells (10, 11), as shown in clinical trials (12). Millions of people are suffering and dying of cancer every year (13). Although quercetin exhibits prooxidant activity at lower concentrations, its activity is reversed to antioxidant at higher concentrations (14).
Many unnecessary amino acids are essential for the growth of rapidly dividing tumor cells and to support and regulate the synthesis of nucleotides, macromolecule biosynthesis, cellular antioxidant systems, epigenetic changes, post-translational modifications, and many other cellular functions (15-18). Arginine is one of the conditionally essential amino acids for humans, and it has been shown that cancer cells are deficient in arginine (19). Also, it has been shown that tumor cells are sensitive to the absence of arginine, which provides a therapeutic strategy for eradicating cancer cells (20, 21). Arginine metabolism is interrelated with urea metabolism, synthesis of polyamines (putrescin, spermine and spermidine), as well as pyrimidine and nitric oxide biosynthesis. Besides, arginine metabolism has been associated with the development of many tumors (22-24). Changes in arginine metabolism might increase the mutation load of cells and alter cellular proliferation (25).
Human embryonic kidney 293 (HEK293) is a human cell line derived from embryonic kidney cells (26). It is used as a cellular model to study the effects of different substances on cancer cells (27). This cell line may also be extracted from the immature neonatal neurons of human kidney cells (28).
2. Objectives
In this study, we aimed to investigate the gene expression of a number of key enzymes involved in arginine metabolism in the HEK293 cells treated with quercetin.
3. Methods
3.1. Cell Culture and Quercetin Treatment
Human embryonic kidney 293 cells were acquired from the Iranian Biological Research Center, Karaj, Iran, and stored at 37°C under 5% CO2 and appropriate humidity. These cells were preserved in a medium containing 89% high-glucose DMEM (Gibco, USA), 10% fetal bovine serum (FBS) (Gibco, USA), and 1% penicillin 100 μg/mL and streptomycin 100 U/mL and cultured in 6-well cell culture plates. After reaching 70% confluence, the cells were treated with 57.5 and 115 µM quercetin (Sigma, USA) for 24 hours. Also, a set of untreated cells was used as control.
3.2. Cell Viability Assay
Human embryonic kidney 293 cells were cultured in a 96-well plate and incubated for 24, 48, and 72 h in a CO2 incubator before being exposed to different concentrations of quercetin (320, 160, 80, 40, 20, 10, 5, 2.5 and 1.25 µM). Then the cells were kept in the CO2 incubator for 24 h. After that, MTT reagent (4 - 5 mg/mL in sterile PBS) (Sigma, USA) was added to the wells, and incubation continued for another 4 h. Next, dimethyl sulfoxide (DMSO, Sigma, USA) was added to the wells which were then shaken. Optical density was read at 580 nm (29).
3.3. RNA Extraction and cDNA Synthesis
After 24 hours of treatment with 57.5 and 115 µM quercetin, RNA was extracted (blood/cultured cell total RNA mini kit, Favorgen Biotech) from HEK293 cells. The quality of extracted RNA was evaluated by a nanodrop (Nabi, Korea). The extracted RNA was then exposed to DNase I enzyme (Sinaclon, DNase I. RNase-free), and cDNA was synthesized (Roje technology, nRT-ROSET Kit).
3.4. Real-time PCR
Complementary DNA was used as a template for gene expression analysis. Using Oligo 7, IDT (integrated DNA technologies) and NCBI primer blast software, primers were designed for the genes of the enzymes involved in arginine metabolic pathways (Table 1), and the specificity of the designed primers was also evaluated (Table 1). Glyceraldehyde 3-phosphate dehydrogenase (GAPDH) and 18s rRNA genes were considered as internal controls. Quantitative expression of the genes was evaluated by the SYBR Green method [real QPCR 2x mix (Green), amplicon] (first cycle: 95°C for 15 minutes followed by 40 cycles: 25 seconds at 95°C and 60 seconds at 60°C). Gene expression was analyzed using a real-time PCR instrument (LightCycler® 96 Instrument, Roche).
The Primers Used in This Study for Assessing the Gene Expression of Arginine Metabolic Enzymes
Genes | Forward Primer | Reverse Primer |
---|---|---|
Glyceraldehyde 3-phosphate dehydrogenase (NM-001256799.3) (30) | 5’-CCTGCACCACCAACTGCTTA-3’ | 5’-CATGAGTCCTTCCACGATACCA-3’ |
18s rRNA (31) | 5’-GTAACCCGTTGAACCCCATT-3’ | 5’-CCATCCAATCGGTAGTAGCG-3’ |
Peptidylprolyl isomerase A (NM-001300981.2) | 5’-GGGTTTATGTGTCAGGGTGGT-3’ | 5’-ATGGACAAGATGCCAGGACC-3’ |
Arginase 1 (NM-000045.4) | 5’-CTGAGGGTTGACTGACTGGA-3’ | 5’-TCTCAAGCAGACCAGCCTTTC-3’ |
Arginase 2 (NM-001172.4) | 5’-TGATAGGAGCCCCGTTCTCAC-3’ | 5’-ACTGGAGAGCCTTTTCATCAAGC-3’ |
Agmatinase (NM-024758.5) | 5’-CCTTCCAGTCCCTCATGGTTG-3’ | 5’-GTGATCTCCACCCAAGGTCAG-3’ |
Arginine decarboxylase (NM-001293562.2) | 5’-AGCCTTGGACCTGTACTT-3’ | 5’-TCTAGCAGAACCTCCTTCTT-3’ |
Ornithine carbamoyltransferase (NM-000531.6) | 5’-GGCTGTCAGATTTGTACCATCC-3’ | 5’-TTGTTCCCATCCCCGATCCAG-3’ |
Spermidine synthase (NM-003132.3) | 5’-ATCCTCGTCTTCCGCAGTAA-3’ | 5’-TTGGCGATCATCTCCTGGTA-3’ |
Spermin synthase (NM-001258423.1) | 5’-CCTCACTATGGCAGCAGCA-3’ | 5’-GCTCCTGGAAAATGGACTGGA-3’ |
Nitric oxide synthase 1 (NM-000620.5) | 5’-GCAACACCCCTCTCTTGGAC-3’ | 5’-CAAAGTTTCTGCTGCGTGCTC-3’ |
Argininosuccinate lyase (NM-000048.4) | 5’-CCACTGGCGTCATCTCTACG-3’ | 5’-GAATGGCATCCCTTTGCGG-3’ |
Argininosuccinate synthase 1 (NM-000050.4) | 5’-AGCAGGCACCATCCTTTACC-3’ | 5’-CACTTTCCCTTCCACTCGCT-3’ |
Ornithine decarboxylase 1 (NM-001287188.2) | 5’-TTGCGGATTGCCACTGATGAT-3’ | 5’-TCAGAGATTGCCTGCACGAAG-3’ |
3.5. Statistical Analysis
Statistical quantitative gene expression analysis of arginine metabolic enzymes was performed using REST 2009 (Corbett research) and GraphPad Prism 8 software packages. The Student t-test was used for statistical analysis, and a P-value of < 0.05 was considered as the significance level. Regression analysis was used to evaluate the primers’ efficiency scores.
4. Results
Herbal remedies, medicinal plants, and their ingredients are among the agents employed to fight cancer. Today, numerous researchers around the world are investigating the effects of these plant ingredients on cancer cells (32, 33). One of these plant ingredients is quercetin which is a flavonoid with proven anticancer properties (34, 35). Arginine is a conditionally essential amino acid for humans; however, many cancer cells are deficient in arginine. Therefore, this amino acid becomes essential to these cells (36). In this research, MTT assay showed that the concentrations of 2.5 μM to 1.2 mM of quercetin did not exert any toxicity against HEK293 cells. Therefore, we exposed HEK293 cells to 57.5 and 115 µM quercetin concentrations and observed altered gene expression of some of the studied arginine metabolic enzymes. These cells were treated with these concentrations of quercetin for 24 hours.
In arginine metabolism, ornithine carbamoyltransferase converts L-ornithine and carbamoylphosphate into L-citrulline in mitochondria. This enzyme had no expression in neither untreated HEK293 cells nor the cells treated with 57.5 and 115 µM quercetin. Arginase I and II convert L-arginine to L-ornithine. Arginase 1 enzyme is located in cytoplasm while arginase 2 is found in mitochondria. Arginase 1 showed no expression in the HEK293 cells treated with 57.5 and 115 µM quercetin compared with the control group. On the other hand, while arginase 2 expression did not significantly change in 115 µM quercetin concentration compared with the control, at 57.5 µM quercetin concentration, the gene expression of this enzyme showed a 2.07-fold increase. Arginine decarboxylase enzyme converts L-arginine into agmatine in mitochondria. Although the gene expression of this enzyme in the HEK293 cells treated with 57.5 µM quercetin was not significantly different compared with the control group, at 115 µM concentration, the gene expression of this enzyme showed a 0.85-fold increase. The agmatinase enzyme, which is expressed in mitochondria, converts agmatine into putrescine. The HEK293 cells exposed to 57.5 µM quercetin showed a 1.92-fold increase in the gene expression of this enzyme while at the 115 µM quercetin concentration, a 0.74-fold decrease was seen in agmatinase gene expression compared to the control group. Nitric oxide synthase 1 catalyzes the conversion of L-arginine into nitric oxide and L-citrulline in cytoplasm. In quercetin-treated HEK-293 cells, while the 57.5 µM concentration did not significantly change the gene expression of this gene in comparison with the control group, the HEK293 cells treated with 115 µM quercetin showed a 0.76-fold decrease compared with the control group. Argininosuccinate synthase 1, a cytoplasmic enzyme, converts L-citrulline into argininosuccinate. In the HEK293 cells exposed to the 57.5 µM quercetin concentration, the expression of this enzyme showed a 0.554-fold decrease; nonetheless, at the 115 µM concentration, there was no significant difference between treated and untreated groups. Argininosuccinate lyase, which is also located in cytoplasm, converts argininosuccinate into L-arginine. Although the gene expression of this enzyme did not show significant changes in treated and untreated cells at the 57.5 µM quercetin concentration, the expression of this enzyme at the 115 µM quercetin concentration showed a 33.513-fold increase. The conversion of L-ornithine to putrescin is mediated by ornithine decarboxylase in cytoplasm. At 57.5 and 115 µM quercetin concentrations, the gene expression of this enzyme increased 1.31- and 1.225-fold, respectively. In another enzymatic reaction in cytoplasm, spermidine synthase converts putrescin to spermidine. In the HEK293 cells treated with 57.5 and 115 µM quercetin, the expression of this enzyme increased by 2.7- and 2.05-fold, respectively, compared to the control group. Spermine synthase also catalyzes the conversion of spermidine to spermine in cytoplasm. In the HEK293 cells treated with 57.5 µM quercetin, the expression of this enzyme showed a 0.885-fold decrease. However, at the 115 µM concentration, the expression of this enzyme was not significantly different between quercetin-treated and untreated cells (Figure 1).
Changes in the relative gene expression of arginine metabolic enzymes in the HEK 293 cells treated with 57.5 and 115 µM quercetin for 24 hours. The data was analyzed with the Student t-test considering a P-value of < 0.05 as the significance level. All differences were significant.
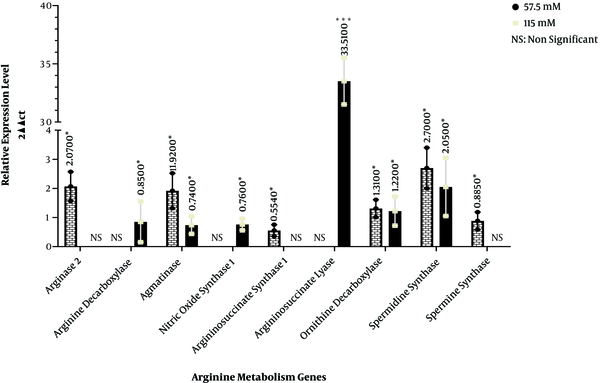
5. Discussion
Cancer is one of the leading causes of death worldwide, that affects the lives of millions of people every year (13). A lot of research is underway to fight with this disease (37-39). Arginine is one of conditionally essential amino acids for normal human cells and is involved in the synthesis of many tumor-inducing metabolites in cancer cells, influencing their proliferation, growth, and metastasis (40, 41). Various types of cancer cells may be either arginine dependent or independent (42). Arginine metabolism involves the conversion of L-arginine to L-ornithine by arginase I and II enzymes. Arginase I is a cytosolic enzyme in liver cells. Arginase II; on the other hand, is a mitochondrial enzyme mostly expressed in non-liver cells (43). Although arginase I was not expressed in HEK293 cells, the expression of arginase II significantly increased in the HEK293 cells treated with quercetin at the 57.5 µM concentration. Arginine metabolism is involved in the production of putrescin, spermine, and spermidine polyamines (44). Three enzymes including ornithine decarboxylase 1, spermine synthase, and spermidine synthase catalyze the enzymatic reactions leading to the production of these polyamines. Oncogenes and free polyamine content play a significant role in determining the amount of ornithine decarboxylase 1, spermine synthase, and spermidine synthase proteins (45, 46). In this study, the gene expression of these enzymes significantly decreased in the HEK293 cells exposed to the 115 µM quercetin concentration compared to the cells treated with the 57.5 µM concentration of this flavonoid. This indicates the anti-tumor effects of quercetin by reducing the synthesis of the catalytic enzymes involved in arginine metabolism. On the other hand, arginine decarboxylase that catalyzes the synthesis of agmatine from L-arginine can reduce the production of polyamines by inhibiting ornithine decarboxylase 1 (47). The gene expression of this enzyme showed a significant increase in the HEK293 cells treated with 115 µM quercetin. So, the decreased expression of polyamines observed in this study could be attributed to the action of an ornithine decarboxylase 1-independent pathway. Previous studies on various cell lines have shown that quercetin reduces ornithine decarboxylase (ODC) expression. However, this study showed that the expression of this enzyme increased in quercetin-treated HEK 293 cells (48). Also, nitric oxide synthase 1 plays an important role in arginine metabolism. In human cells, the three enzymes of inducible nitric oxide synthase (iNOS), neuronal nitric oxide synthase (nNOS), and endothelial nitric oxide synthase (eNOS) are involved in the conversion of L-arginine to nitric oxide and L-citrulline, and each of these enzymes has its own characteristics (49). In this study, nNOS, which is expressed in neurons, was upregulated in the HEK 293 cells treated with 115 µM quercetin. This enzyme can be involved in carcinogenesis as well (49-51). In another study on rat models of arterial erectile dysfunction, it was shown that quercetin treatment had no effect on nNOS expression (52).
Argininosuccinate synthase 1 and argininosuccinate lyase are two enzymes converting citrulline to arginine. Argininosuccinate lyase plays a vital role in the growth of various tumor cells and has been suggested as a therapeutic target for treating cancers (53-55). This enzyme significantly increased in the HEK 293 cells treated with 115 µM quercetin. The argininosuccinate synthase 1 enzyme exhibits variable expression in different cancers (42). In this study, argininosuccinate synthase 1 showed a significant increase in the HEK 293 cells exposed to 57.5 µM quercetin. Consistent with our findings, the hyperammonemic rats fed with 50 mg/kg body weight quercetin showed overexpressed ASS1, OTC, and ARG (56).
5.1. Conclusion
To the best of our knowledge, this is the first study investigating arginine metabolic enzymes in the HEK293 cells treated with quercetin. It was shown that quercetin could change the gene expression of the enzymes involved in arginine metabolism. The findings of this study suggest investigating the role of plant flavonoids in future cancer therapy research.
Acknowledgements
References
-
1.
Babaei F, Mirzababaei M, Nassiri-Asl M. Quercetin in food: Possible mechanisms of its effect on memory. J Food Sci. 2018;83(9):2280-7. [PubMed ID: 30103275]. https://doi.org/10.1111/1750-3841.14317.
-
2.
Kawabata K, Mukai R, Ishisaka A. Quercetin and related polyphenols: New insights and implications for their bioactivity and bioavailability. Food Funct. 2015;6(5):1399-417. [PubMed ID: 25761771]. https://doi.org/10.1039/c4fo01178c.
-
3.
Nishimuro H, Ohnishi H, Sato M, Ohnishi-Kameyama M, Matsunaga I, Naito S, et al. Estimated daily intake and seasonal food sources of quercetin in Japan. Nutrients. 2015;7(4):2345-58. [PubMed ID: 25849945]. [PubMed Central ID: PMC4425148]. https://doi.org/10.3390/nu7042345.
-
4.
Kumar R, Vijayalakshmi S, Nadanasabapathi S. Health benefits of quercetin. Def Life Sci J. 2017;2(2):142-51. https://doi.org/10.14429/dlsj.2.11359.
-
5.
Ezzati Nazhad Dolatabadi J, Mokhtarzadeh A, Ghareghoran SM, Dehghan G. Synthesis, characterization and antioxidant property of quercetin-Tb(III) complex. Adv Pharm Bull. 2014;4(2):101-4. [PubMed ID: 24511472]. [PubMed Central ID: PMC3915808]. https://doi.org/10.5681/apb.2014.016.
-
6.
Faria A, Pestana D, Teixeira D, Azevedo J, De Freitas V, Mateus N, et al. Flavonoid transport across RBE4 cells: A blood-brain barrier model. Cell Mol Biol Lett. 2010;15(2):234-41. [PubMed ID: 20140760]. [PubMed Central ID: PMC6275689]. https://doi.org/10.2478/s11658-010-0006-4.
-
7.
Pei B, Yang M, Qi X, Shen X, Chen X, Zhang F. Quercetin ameliorates ischemia/reperfusion-induced cognitive deficits by inhibiting ASK1/JNK3/caspase-3 by enhancing the Akt signaling pathway. Biochem Biophys Res Commun. 2016;478(1):199-205. [PubMed ID: 27450812]. https://doi.org/10.1016/j.bbrc.2016.07.068.
-
8.
Heinz SA, Henson DA, Austin MD, Jin F, Nieman DC. Quercetin supplementation and upper respiratory tract infection: A randomized community clinical trial. Pharmacol Res. 2010;62(3):237-42. [PubMed ID: 20478383]. [PubMed Central ID: PMC7128946]. https://doi.org/10.1016/j.phrs.2010.05.001.
-
9.
Wong G, He S, Siragam V, Bi Y, Mbikay M, Chretien M, et al. Antiviral activity of quercetin-3-beta-O-D-glucoside against Zika virus infection. Virol Sin. 2017;32(6):545-7. [PubMed ID: 28884445]. [PubMed Central ID: PMC6598929]. https://doi.org/10.1007/s12250-017-4057-9.
-
10.
Khan F, Niaz K, Maqbool F, Ismail Hassan F, Abdollahi M, Nagulapalli Venkata KC, et al. Molecular targets underlying the anticancer effects of quercetin: An update. Nutrients. 2016;8(9). [PubMed ID: 27589790]. [PubMed Central ID: PMC5037516]. https://doi.org/10.3390/nu8090529.
-
11.
Russo GL, Russo M, Spagnuolo C, Tedesco I, Bilotto S, Iannitti R, et al. Quercetin: A pleiotropic kinase inhibitor against cancer. Cancer Treat Res. 2014;159:185-205. [PubMed ID: 24114481]. https://doi.org/10.1007/978-3-642-38007-5_11.
-
12.
Kooshyar MM, Mozafari PM, Amirchaghmaghi M, Pakfetrat A, Karoos P, Mohasel MR, et al. A randomized placebo- controlled double blind clinical trial of quercetin in the prevention and treatment of chemotherapy-induced oral mucositis. J Clin Diagn Res. 2017;11(3):ZC46-50. [PubMed ID: 28511508]. [PubMed Central ID: PMC5427434]. https://doi.org/10.7860/JCDR/2017/23975.9571.
-
13.
McGuire S. World cancer report 2014. Geneva, Switzerland: World Health Organization, International Agency for Research on Cancer, WHO Press, 2015. Adv Nutr. 2016;7(2):418-9. [PubMed ID: 26980827]. [PubMed Central ID: PMC4785485]. https://doi.org/10.3945/an.116.012211.
-
14.
Min K, Ebeler SE. Quercetin inhibits hydrogen peroxide-induced DNA damage and enhances DNA repair in Caco-2 cells. Food Chem Toxicol. 2009;47(11):2716-22. [PubMed ID: 19651184]. https://doi.org/10.1016/j.fct.2009.07.033.
-
15.
Meng M, Chen S, Lao T, Liang D, Sang N. Nitrogen anabolism underlies the importance of glutaminolysis in proliferating cells. Cell Cycle. 2010;9(19):3921-32. [PubMed ID: 20935507]. [PubMed Central ID: PMC3047752]. https://doi.org/10.4161/cc.9.19.13139.
-
16.
Ma Z, Vosseller K. O-GlcNAc in cancer biology. Amino Acids. 2013;45(4):719-33. [PubMed ID: 23836420]. https://doi.org/10.1007/s00726-013-1543-8.
-
17.
Choi BH, Coloff JL. The diverse functions of non-essential amino acids in cancer. Cancers. 2019;11(5). [PubMed ID: 31096630]. [PubMed Central ID: PMC6562791]. https://doi.org/10.3390/cancers11050675.
-
18.
Zhang J, Pavlova NN, Thompson CB. Cancer cell metabolism: The essential role of the nonessential amino acid, glutamine. EMBO J. 2017;36(10):1302-15. [PubMed ID: 28420743]. [PubMed Central ID: PMC5430235]. https://doi.org/10.15252/embj.201696151.
-
19.
Sullivan MR, Danai LV, Lewis CA, Chan SH, Gui DY, Kunchok T, et al. Quantification of microenvironmental metabolites in murine cancers reveals determinants of tumor nutrient availability. Elife. 2019;8. [PubMed ID: 30990168]. [PubMed Central ID: PMC6510537]. https://doi.org/10.7554/eLife.44235.
-
20.
Dang CV. Essentiality of non-essential amino acids for tumour cells and tumorigenesis. Nat Metab. 2019;1(9):847-8. [PubMed ID: 32694747]. https://doi.org/10.1038/s42255-019-0113-0.
-
21.
Al-Koussa H, El Mais N, Maalouf H, Abi-Habib R, El-Sibai M. Arginine deprivation: A potential therapeutic for cancer cell metastasis? A review. Cancer Cell Int. 2020;20:150. [PubMed ID: 32390765]. [PubMed Central ID: PMC7201942]. https://doi.org/10.1186/s12935-020-01232-9.
-
22.
Eyler CE, Wu Q, Yan K, MacSwords JM, Chandler-Militello D, Misuraca KL, et al. Glioma stem cell proliferation and tumor growth are promoted by nitric oxide synthase-2. Cell. 2011;146(1):53-66. [PubMed ID: 21729780]. [PubMed Central ID: PMC3144745]. https://doi.org/10.1016/j.cell.2011.06.006.
-
23.
Keshet R, Szlosarek P, Carracedo A, Erez A. Rewiring urea cycle metabolism in cancer to support anabolism. Nat Rev Cancer. 2018;18(10):634-45. [PubMed ID: 30194362]. https://doi.org/10.1038/s41568-018-0054-z.
-
24.
Gerner EW, Bruckheimer E, Cohen A. Cancer pharmacoprevention: Targeting polyamine metabolism to manage risk factors for colon cancer. J Biol Chem. 2018;293(48):18770-8. [PubMed ID: 30355737]. [PubMed Central ID: PMC6290143]. https://doi.org/10.1074/jbc.TM118.003343.
-
25.
Lee JS, Adler L, Karathia H, Carmel N, Rabinovich S, Auslander N, et al. Urea cycle dysregulation generates clinically relevant genomic and biochemical signatures. Cell. 2018;174(6):1559-1570 e22. [PubMed ID: 30100185]. [PubMed Central ID: PMC6225773]. https://doi.org/10.1016/j.cell.2018.07.019.
-
26.
Graham FL, Smiley J, Russell WC, Nairn R. Characteristics of a human cell line transformed by DNA from human adenovirus type 5. J Gen Virol. 1977;36(1):59-74. [PubMed ID: 886304]. https://doi.org/10.1099/0022-1317-36-1-59.
-
27.
Stepanenko AA, Dmitrenko VV. HEK293 in cell biology and cancer research: Phenotype, karyotype, tumorigenicity, and stress-induced genome-phenotype evolution. Gene. 2015;569(2):182-90. [PubMed ID: 26026906]. https://doi.org/10.1016/j.gene.2015.05.065.
-
28.
Shaw G, Morse S, Ararat M, Graham FL. Preferential transformation of human neuronal cells by human adenoviruses and the origin of HEK 293 cells. FASEB J. 2002;16(8):869-71. [PubMed ID: 11967234]. https://doi.org/10.1096/fj.01-0995fje.
-
29.
Teicher BA, Lazo JS, Sartorelli AC. Classification of antineoplastic agents by their selective toxicities toward oxygenated and hypoxic tumor cells. Cancer Res. 1981;41(1):73-81. [PubMed ID: 7448778].
-
30.
Rodriguez-Jimenez FJ, Krause A, Schulz S, Forssmann WG, Conejo-Garcia JR, Schreeb R, et al. Distribution of new human beta-defensin genes clustered on chromosome 20 in functionally different segments of epididymis. Genomics. 2003;81(2):175-83. [PubMed ID: 12620395]. https://doi.org/10.1016/s0888-7543(02)00034-4.
-
31.
Turner N, Lim XY, Toop HD, Osborne B, Brandon AE, Taylor EN, et al. A selective inhibitor of ceramide synthase 1 reveals a novel role in fat metabolism. Nat Commun. 2018;9(1):3165. [PubMed ID: 30131496]. [PubMed Central ID: PMC6104039]. https://doi.org/10.1038/s41467-018-05613-7.
-
32.
Chen M, May BH, Zhou IW, Xue CC, Zhang AL. Meta-analysis of oxaliplatin-based chemotherapy combined with traditional medicines for colorectal cancer: Contributions of specific plants to tumor response. Integr Cancer Ther. 2016;15(1):40-59. [PubMed ID: 26254190]. [PubMed Central ID: PMC5736077]. https://doi.org/10.1177/1534735415596424.
-
33.
Jaradat NA, Shawahna R, Eid AM, Al-Ramahi R, Asma MK, Zaid AN. Herbal remedies use by breast cancer patients in the West Bank of Palestine. J Ethnopharmacol. 2016;178:1-8. [PubMed ID: 26656536]. https://doi.org/10.1016/j.jep.2015.11.050.
-
34.
Jeong JH, An JY, Kwon YT, Rhee JG, Lee YJ. Effects of low dose quercetin: Cancer cell-specific inhibition of cell cycle progression. J Cell Biochem. 2009;106(1):73-82. [PubMed ID: 19009557]. [PubMed Central ID: PMC2736626]. https://doi.org/10.1002/jcb.21977.
-
35.
Vidya Priyadarsini R, Senthil Murugan R, Maitreyi S, Ramalingam K, Karunagaran D, Nagini S. The flavonoid quercetin induces cell cycle arrest and mitochondria-mediated apoptosis in human cervical cancer (HeLa) cells through p53 induction and NF-kappaB inhibition. Eur J Pharmacol. 2010;649(1-3):84-91. [PubMed ID: 20858478]. https://doi.org/10.1016/j.ejphar.2010.09.020.
-
36.
Delage B, Fennell DA, Nicholson L, McNeish I, Lemoine NR, Crook T, et al. Arginine deprivation and argininosuccinate synthetase expression in the treatment of cancer. Int J Cancer. 2010;126(12):2762-72. [PubMed ID: 20104527]. https://doi.org/10.1002/ijc.25202.
-
37.
Hientz K, Mohr A, Bhakta-Guha D, Efferth T. The role of p53 in cancer drug resistance and targeted chemotherapy. Oncotarget. 2017;8(5):8921-46. [PubMed ID: 27888811]. [PubMed Central ID: PMC5352454]. https://doi.org/10.18632/oncotarget.13475.
-
38.
Jose A, Labala S, Venuganti VV. Co-delivery of curcumin and STAT3 siRNA using deformable cationic liposomes to treat skin cancer. J Drug Target. 2017;25(4):330-41. [PubMed ID: 27819148]. https://doi.org/10.1080/1061186X.2016.1258567.
-
39.
Galanis E, Atherton PJ, Maurer MJ, Knutson KL, Dowdy SC, Cliby WA, et al. Oncolytic measles virus expressing the sodium iodide symporter to treat drug-resistant ovarian cancer. Cancer Res. 2015;75(1):22-30. [PubMed ID: 25398436]. [PubMed Central ID: PMC4377302]. https://doi.org/10.1158/0008-5472.CAN-14-2533.
-
40.
Albaugh VL, Pinzon-Guzman C, Barbul A. Arginine-Dual roles as an onconutrient and immunonutrient. J Surg Oncol. 2017;115(3):273-80. [PubMed ID: 27861915]. [PubMed Central ID: PMC6486789]. https://doi.org/10.1002/jso.24490.
-
41.
Patil MD, Bhaumik J, Babykutty S, Banerjee UC, Fukumura D. Arginine dependence of tumor cells: Targeting a chink in cancer's armor. Oncogene. 2016;35(38):4957-72. [PubMed ID: 27109103]. [PubMed Central ID: PMC5457742]. https://doi.org/10.1038/onc.2016.37.
-
42.
Qiu F, Huang J, Sui M. Targeting arginine metabolism pathway to treat arginine-dependent cancers. Cancer Lett. 2015;364(1):1-7. [PubMed ID: 25917076]. https://doi.org/10.1016/j.canlet.2015.04.020.
-
43.
Caldwell RB, Toque HA, Narayanan SP, Caldwell RW. Arginase: An old enzyme with new tricks. Trends Pharmacol Sci. 2015;36(6):395-405. [PubMed ID: 25930708]. [PubMed Central ID: PMC4461463]. https://doi.org/10.1016/j.tips.2015.03.006.
-
44.
Morris SMJ. Arginine metabolism revisited. J Nutr. 2016;146(12):2579S-86S. [PubMed ID: 27934648]. https://doi.org/10.3945/jn.115.226621.
-
45.
Casero RAJ, Murray Stewart T, Pegg AE. Polyamine metabolism and cancer: Treatments, challenges and opportunities. Nat Rev Cancer. 2018;18(11):681-95. [PubMed ID: 30181570]. [PubMed Central ID: PMC6487480]. https://doi.org/10.1038/s41568-018-0050-3.
-
46.
O'Brien TG, Simsiman RC, Boutwell RK. Induction of the polyamine-biosynthetic enzymes in mouse epidermis by tumor-promoting agents. Cancer Res. 1975;35(7):1662-70. [PubMed ID: 48421].
-
47.
Wolf C, Bruss M, Hanisch B, Gothert M, von Kugelgen I, Molderings GJ. Molecular basis for the antiproliferative effect of agmatine in tumor cells of colonic, hepatic, and neuronal origin. Mol Pharmacol. 2007;71(1):276-83. [PubMed ID: 17047095]. https://doi.org/10.1124/mol.106.028449.
-
48.
Saunders FR, Wallace HM. On the natural chemoprevention of cancer. Plant Physiol Biochem. 2010;48(7):621-6. [PubMed ID: 20347597]. https://doi.org/10.1016/j.plaphy.2010.03.001.
-
49.
Fukumura D, Kashiwagi S, Jain RK. The role of nitric oxide in tumour progression. Nat Rev Cancer. 2006;6(7):521-34. [PubMed ID: 16794635]. https://doi.org/10.1038/nrc1910.
-
50.
Bonavida B, Garban H. Nitric oxide-mediated sensitization of resistant tumor cells to apoptosis by chemo-immunotherapeutics. Redox Biol. 2015;6:486-94. [PubMed ID: 26432660]. [PubMed Central ID: PMC4596920]. https://doi.org/10.1016/j.redox.2015.08.013.
-
51.
Napoli C, Paolisso G, Casamassimi A, Al-Omran M, Barbieri M, Sommese L, et al. Effects of nitric oxide on cell proliferation: Novel insights. J Am Coll Cardiol. 2013;62(2):89-95. [PubMed ID: 23665095]. https://doi.org/10.1016/j.jacc.2013.03.070.
-
52.
Zhang Y, Huang C, Liu S, Bai J, Fan X, Guo J, et al. Effects of quercetin on intracavernous pressure and expression of nitrogen synthase isoforms in arterial erectile dysfunction rat model. Int J Clin Exp Med. 2015;8(5):7599-605. [PubMed ID: 26221305]. [PubMed Central ID: PMC4509250].
-
53.
Husson A, Brasse-Lagnel C, Fairand A, Renouf S, Lavoinne A. Argininosuccinate synthetase from the urea cycle to the citrulline-NO cycle. Eur J Biochem. 2003;270(9):1887-99. [PubMed ID: 12709047]. https://doi.org/10.1046/j.1432-1033.2003.03559.x.
-
54.
Hung YH, Huang HL, Chen WC, Yen MC, Cho CY, Weng TY, et al. Argininosuccinate lyase interacts with cyclin A2 in cytoplasm and modulates growth of liver tumor cells. Oncol Rep. 2017;37(2):969-78. [PubMed ID: 28035420]. [PubMed Central ID: PMC5355748]. https://doi.org/10.3892/or.2016.5334.
-
55.
Huang HL, Chen WC, Hsu HP, Cho CY, Hung YH, Wang CY, et al. Argininosuccinate lyase is a potential therapeutic target in breast cancer. Oncol Rep. 2015;34(6):3131-9. [PubMed ID: 26397737]. https://doi.org/10.3892/or.2015.4280.
-
56.
Kanimozhi S, Subramanian P, Shanmugapriya S, Sathishkumar S. Role of bioflavonoid quercetin on expression of urea cycle enzymes, astrocytic and inflammatory markers in hyperammonemic rats. Indian J Clin Biochem. 2017;32(1):68-73. [PubMed ID: 28149015]. [PubMed Central ID: PMC5247373]. https://doi.org/10.1007/s12291-016-0575-8.