Abstract
Background:
Considering the increased rate of microbial resistance to antibiotics and chemical side effects of antibiotics, there is a need for an alternative antimicrobial agent with fewer complications. Medicinal plants are rich resources of phytochemical compounds with antibacterial activity that could fight off this problem.Objectives:
The aim of this research was to investigate the chemical composition, antimicrobial, and antibiofilm properties of Malva sylvestris on some pathogenic bacteria.Methods:
Antibacterial effect of the extract was assessed by the well diffusion and broth microdilution methods against Staphylococcus aureus, Enterococcus faecalis, Pseudomonas aeruginosa, Klebsiella pneumoniae, and Escherichia coli. The anti-biofilm property of the extract was also examined using the crystal violet assay. Finally, the chemical constituents and phenolic compounds of the extract were determined by gas chromatography-mass spectrometry (GC-MS) and high-performance liquid chromatography (HPLC), respectively.Results:
The methanolic extract of M. sylvestris showed antimicrobial activity against all tested Gram-negative and Gram-positive strains by the agar well diffusion method. The minimum inhibitory concentration (MIC) of the extract ranged from 21.9 ± 0.1 to 51.9 ± 0.5 mg/mL against the tested microorganisms. In addition, the minimum bactericidal concentration (MBC) spanned from 43.7 ± 0.1 to 85.8 ± 0.3 mg/mL. The biofilm inhibitory concentration (BIC50) of the extract was found to be 40 - 87 mg/mL against the tested bacteria. Analysis of the extract by GC-MS indicated that the most abundant compounds were 1-heptacosanol (38.41%), 17-Pentatriacontene (19.78%), and 6,9,12,15-docosatetraenoic acid, methyl ester (8.08%). High-performance liquid chromatography confirmed the presence of apigenin (6.84 ppm) and salicylic acid (1.5 ppm) as phenolic compounds in M. sylvestris methanolic extract.Conclusions:
The results of this study represent the high potency of M. sylvestris extract as a source of biologically-active compounds for the development of future phytotherapeutic products with antibacterial and antibiofilm activity.Keywords
Antibacterial Agent GC-MS, HPLC Medicinal Plants Malva sylvestris Apigenin Salicylic Acid
1. Background
A biofilm is a microbial community characterized by adhesion to biotic or abiotic surfaces, which is embedded by extracellular polymeric substances. This matrix gives sanctuary to internal cells and helps them to absorb nutrients and adhere to the associated surfaces (1).
Biofilms play a significant role in the survival of bacterial cells under adverse environmental conditions. Moreover, bacteria in biofilms are more resistant to biocides and immune system than their planktonic counterparts (2). It is estimated that about 65% of all bacterial infections are related to bacterial biofilms. Numerous human and veterinary pathogens, including Pseudomonas aeruginosa, Escherichia coli, Enterococcus faecalis, Staphylococcus aureus, Klebsiella pneumoniae, Acinetobacter baumannii, and Salmonella spp., cause infections which are difficult to treat due to their potency to produce sessile biofilms (3). Bacterial cells in biofilms can be released and cause a discontinuous secondary contamination of various products during the production process (4). In addition, in the food industry, equipment and surfaces are often contaminated by biofilm-producing microorganisms. In most cases, this issue becomes a major problem because these biofilms can act as a source of cross-contamination in foods (1).
Antimicrobial agents are not effective against biofilm form, and there are few novel compounds under development. In recent years, interest in natural antimicrobial products to overcome the biofilm form of bacteria has increased (5).
Medicinal plants are non-toxic or less toxic, cost-effective, easily accessible, and safe resources for drugs. Herbs contain phytochemical compounds, such as alkaloids, terpenoids, tannins, and flavonoids, which have antimicrobial activity against planktonic (6) and biofilm forms of bacteria (5, 7).
Malva sylvestris belongs to the Malvaceae family. Previous studies revealed the presence of sugars, fatty acids, amino acids, vitamins, mucilages, coumarins, and some sterols and triterpenes in this plant. Furthermore, it has been reported that M. sylvestris possesses significant quantities of phenols and total flavonoids (8, 9).
Malva sylvestris commonly grows in Europe, North Africa, and various parts of Asia. In Iran, M. sylvestris is found around Tehran, Gilan, and the central and southern parts of Iran, such as Bandar Abbas and Qeshm (10). In Iran, M. sylvestris (named as Panirak) is commonly used as a vegetable and for medicinal purposes (10). The flowers of this plant are used for the treatment of bronchitis, eczema, digestive problems, inflammations, cut wound, and dermal infected wounds (11, 12).
The antimicrobial activity of M. sylvestris extract against some pathogenic bacteria has been confirmed previously. Razavi et al. (11) showed that the flowers extract of M. sylvestris had high antibacterial effects against some human pathogenic bacteria, such as Enterococcus faecalis, Streptococcus agalactiae, and Staphylococcus aureus. In a study conducted by Shadid et al. (13), the acetonic extract of M. sylvestris exhibited strong antibacterial activity against Proteus vulgaris and S. aureus with the MIC values of 0.078 and 0.125 mg/mL, respectively. Other studies have also shown the cytotoxic properties of M. sylvestris extract against Salmonella enterica serovar Typhimurium (14), Listeria monocytogenes (15), Bacillus cereus (15), methicillin-resistant S. aureus (MRSA) (11), Aggregatibacter actinomycetemcomitans (10), and Candida species (16). The limitation of previous studies is that in all these studies, only the antimicrobial properties of the extract were investigated, and its anti-biofilm properties on pathogenic bacteria were not evaluated.
2. Objectives
Accordingly, the aim of the present study was to investigate the antibacterial and antibiofilm activities of M. sylvestris methanolic extract against P. aeruginosa, E. coli, K. pneumoniae, S. aureus, and E. faecalis standard strains. The major bioactive components of the extract were also determined to identify the relationship between the observed effects and the compounds.
3. Methods
3.1. Bacterial Strains
The bacterial strains used in this work were P. aeruginosa ATCC 9027, E. coli ATCC 10536, K. pneumoniae ATCC 10031, S. aureus ATCC 29737, and E. faecalis ATCC 29212. These strains were obtained from the Iranian Research Organization for Science and Technology and cultured aerobically in Nutrient Broth (NB) (Merck, Germany).
3.2. Plant Collection and Extraction
Malva sylvestris was obtained from the Botanical Center of Peykan Shahr, west of Tehran, Iran. The plant was authenticated in the Medicinal Plants and Drug Research Institute Herbarium (MPH) of Shahid Beheshti University (Tehran, Iran). A voucher herbarium specimen of Malva sylvestris L. (no.: 2767) was deposited in MPH. Aerial parts (flower, leaves, and stems) of the plant were thoroughly washed in distilled water. Thereafter, the plants were air-dried at room temperature under shade, pulverized in a hand mill, and saved for future analysis. Plant extraction was performed by the maceration method as described previously (17). In detail, 200 g of powdered M. sylvestris leaves was dissolved in 150 mL of methanol. Then, the samples were placed in a shaker incubator (Memmert, Germany) at 30°C for three days. Afterwards, suspensions were filtrated using a filter paper (Whatman no.1, USA) for four times, and the insoluble parts of suspension were taken. Rotary vacuum (Hidolph, Germany) was used to remove the solvent from the extract. The concentrations of 700 mg/mL were prepared with water plus 1% DMSO (Sigma, Aldrich). Then, the extract was sterilized using a Millipore filter (0.22 µm) and kept in a dark container at 4°C for further analysis.
3.3. Well Diffusion Method
The plant extract was tested for antimicrobial activity using the well diffusion method as described previously (18). The bacterial suspension was provided in Mueller Hinton Broth (MHB) (Merck, Germany), and cell density was adjusted to the 0.5 McFarland standard. Then, 10 µL of the mentioned suspension was delivered onto Mueller Hinton agar (MHA) (Merck, Germany) plates and spread over the surface of agar. Then, holes of 6 mm in diameter were dug with a sterile cork borer, and 100 µl of 50, 100, and 200 mg/mL extract solutions was introduced into the wells. After incubating the plates at 37°C for 24 h, the inhibition zones around the holes were measured for each of the tested bacteria. In this study, standard positive control (penicillin [10 µg] and streptomycin [10 µg]) (Mast, UK) and negative control (DMSO 1%) were included.
3.4. Determination of Minimum Inhibitory Concentration and Minimum Bactericidal Concentration Values
The minimum inhibitory concentration (MIC) and minimum bactericidal concentration (MBC) of the extract were assessed by the broth microdilution method as described by Novy et al. (19) with minor modifications. In detail, serial dilutions of the extract ranging from 1.56 to 200 mg/mL were provided in the microplate. A bacterial suspension at the concentration of 108 CFU/mL was also made and diluted to 1:100. Then, 10 µL of the diluted suspension was added to each microplate well and incubated at 37°C for 24 h. Minimum inhibitory concentration was determined as the lowest concentration that inhibited bacterial growth. To assess the MBC values, 100 µL from each well with no visible growth was subcultured on MHA plates, and the highest dilution that showed no visible growth was considered MBC.
3.5. Antibiofilm Activity
The effect of the plant extract on biofilm formation was investigated using the crystal violet assay (20). Serial two-fold dilutions of the extract were prepared in the concentration range between 2.7 to 700 mg/mL. In addition, bacterial colonies were grown at 37°C for 24 h in Trypticase Soy broth (TSB) (Merck, Germany) (108 CFU/mL), and 1:100 dilution was prepared in a fresh TSB medium. Then, 100 μL of this dilution was aliquoted into each well of the sterile flat-bottomed 96-well polystyrene microplate. Five wells containing medium plus bacteria were considered as control. After incubation at 37°C for 24 h, the media were discarded, and the wells were washed completely three times with sterile distilled water. Thereafter, biofilms were air-dried and stained with 1% crystal violet dye for 20 min at room temperature. Then, the excess dye was discarded, and the microplate was washed three times with sterile distilled water. Finally, by adding 100 µL of 95% ethanol to each well, the dye attached to the bacterial cells was solubilized, and after 15 min, the optical density at 570 nm was detected using a microplate reader (ELx808, BioTek, USA).
The percentage of biofilm inhibition was measured using the following equation:
The lowest concentration of the extract that displayed 50% inhibition against the formation of biofilms was considered as biofilm inhibition concentration (BIC50).
3.6. Determining the Main Components and Phenolic Compound
The main constituents of M. sylvestris were detected by using the gas chromatography-mass spectrometry (GC-MS) analysis according to the previously described method (21).
The GC-MS assessment was performed by Agilent technology 7890 A instrument, with a DB-5 fused silica column (30 m × 0.25 mm; 0.25 µm film thickness) (21). Helium with the flow rate of 1.1 mL/min was applied as the gas carrier. The temperature was set from 70°C to 270°C at 10°C/min. The sample was injected by splitting, and the split ratio was 1:100. The operating parameters of MS included the ion source temperature of 200°C and ionization voltage of 70 eV. The compounds were identified by comparison of the retention indexes obtained in the same column with the reference components. Furthermore, each obtained mass spectra was compared with those from usual electronic libraries, including Wiley and Adams.
The separation of phenolic compounds was carried out by high-performance liquid chromatography (HPLC) according to the standard procedure (21). High-performance liquid chromatography assessment was carried out on a Knauer reversed-phase liquid chromatography system (Knauer, Germany).
The separation was performed using an Eclipse XDB-C18 (4.6 mm × 250 mm, 5 µm) and Agilent (USA) columns in gradient elution as the mobile phase using water containing 0.05% formic acid (solvent A) and acetonitrile/methanol (80:20, v/v) (solvent B).
3.7. Statistical Analysis
SPSS version 20 was applied for statistical analysis. The experiments were done in triplicate, and results were expressed as mean and standard deviation (SD). To compare mean values, Turkey’s test was applied, and the significance level was set at P < 0.05.
4. Results
4.1. Agar Well Diffusion Test
The antibacterial potential of M. sylvestris against five pathogenic bacteria was evaluated according to their inhibition zone. Table 1 displays the inhibition zone values of the five bacterial strains. According to the results, the antimicrobial effect of M. sylvestris extract increased with an increase in its concentration. The largest inhibition zone was observed at the concentration of 200 mg/mL of the extract against S. aureus and K. pneumoniae (P < 0.05).
Antimicrobial Activity of Malva sylvestris Methanolic Extract Against Pathogenic Bacteria by Agar Well Diffusion and Microdilution Methoda, b
Bacteria | Inhibition Zone, mm | MIC, mg/mL | MBC, mg/mL | |||||
---|---|---|---|---|---|---|---|---|
M. sylvestris Extract, mg/mL | Antibiotics** | D | ||||||
50 | 100 | 200 | P | S | ||||
Escherichia coli | 10.4 ± 0.2B | 12.7 ± 0.2C | 15.4 ± 0.3C | 8.1 ± 0.1D | 13.1 ± 0.1B | - | 43.7 ± 0.1C | 85.5 ± 0.3C |
Pseudomonas aeruginosa | 9.4 ± 0.2C | 10.8 ± 0.1D | 12.5 ± 0.1D | * | 10 ± 0.1C | - | 51.9 ± 0.5D | 85.5 ± 0.3C |
Klebsiella pneumoniae | 13. ± 0.1A | 15.9 ± 0.2A | 17.8 ± 0.2A | 10.1 ± 0.1C | 15.1 ± 0.1A | - | 43.7 ± 0.3C | 65.7 ± 0.2B |
Enterococcus faecalis | 10.4 ± 0.1B | 13.3 ± 0.3B | 16.3 ± 0.1B | 24 ± 0.1B | * | - | 32.8 ± 0.3B | 65.6 ± 0.4B |
Staphylococcus aureus | 13.3 ± 0.3A | 15.7 ± 0.2A | 18.2 ± 0.05A | 25 ± 0.3A | 15 ± 0.2A | - | 21.9 ± 0.1A | 43.7 ± 0.1A |
4.2. Minimum Inhibitory Concentration and Minimum Bactericidal Concentration
The antibacterial effect of M. sylvestris extract was assessed against the tested microorganisms according to the CLSI guidelines. The MIC values of the M. sylvestris extract ranged from 21.9 ± 0.1 to 51.9 ± 0.5 mg/mL, while the MBC values spanned from 43.7 ± 0.1 to 85.5 ± 0.3 mg/mL (Table 1). S. aureus was the most sensitive species, followed by E. faecalis, K. pneumoniae, and E. coli. The extract showed lower activity against the growth of P. aeruginosa (51.9 ± 0.5 mg/mL).
4.3. Antibiofilm Activity
The results of in vitro antibiofilm effect of the methanolic extract of M. sylvestris are shown in Table 2. The extract displayed antibiofilm effect against both Gram-positive and Gram-negative strains in a dose-dependent manner. The highest antibiofilm activity was observed against S. aureus, E. faecalis, and K. pneumoniae with the BIC50 value of 40 mg/mL.
Anti-Biofilm Activity of the Methanolic Extract of Malva sylvestris
Bacteria | A | P | BIC50 |
---|---|---|---|
Escherichia coli | 0.34 | 93.66 | 87 |
Klebsiella pneumoniae | 0.52 | 95.46 | 40 |
Staphylococcus aureus | 0.78 | 89.19 | 40 |
Pseudomonas aeruginosa | 2.25 | > 100 | 87 |
Enterococcus faecalis | 0.84 | 98.79 | 40 |
4.4. Volatile Compounds of the Extract
Figure 1 shows the GC-MS chromatogram of the methanolic extract. The chemical composition of volatile compounds is also shown in Table 3, where the plant components are presented in order of their elution on the DB-5 column. In GC-MS analysis, 11 substances were detected. According to our results, the major chemical components were observed as 1-heptacosanol (38.41%), 17-pentatriacontene (19.78%), and 6,9,12,15-docosatetraenoic acid, methyl ester (8.08%).
GC-MS chromatogram of the methanolic extract of Malva sylvestris
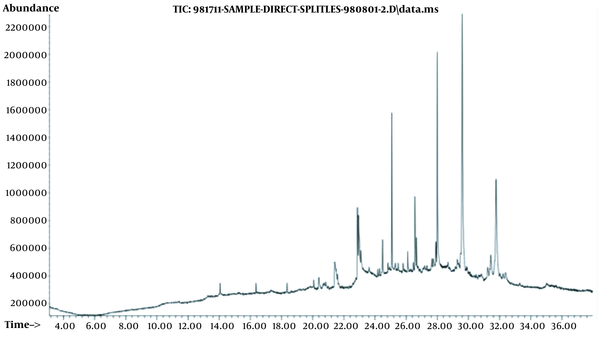
Chemical Compositions, Retention Time, and the Percentage of Volatile Components from the Methanolic Extract of Malva sylvestris
Peak | Retention Time | Name | Percentage, % |
---|---|---|---|
1 | 21.397 | Ethyl iso-allocholate | 5.5 |
2 | 22.87 | 9,12-Octadecadienoic acid, methyl ester | 7.0 |
3 | 22.95 | 6,9,12,15-Docosatetraenoic acid, methyl ester | 8.1 |
4 | 23.09 | Cyclopropanedodecanoic acid, 2-octyl-, methyl ester | 2.8 |
5 | 24.17 | 1-Hexadecanoic acid (hydroxymethyl)-1,2-ethanediyl ester | 0.7 |
6 | 24.48 | 7-Methyl-Z-tetradecen-1-ol acetate | 3.6 |
7 | 25.07 | 3-Methyltricosane | 10.0 |
8 | 26.66 | Oleic acid, 3-(octadecyloxy) propyl ester | 2.1 |
9 | 27.91 | Z-12-Pentacosene | 2.0 |
10 | 29.60 | 1-Heptacosanol | 38.4 |
11 | 31.77 | 17-Pentatriacontene | 19.8 |
Total | - | - | 100 |
4.5. Phenolic Compounds Analyses
Here, our goal was to analyze the phenolic profile of the M. sylvestris methanolic extract to gain insight into its bioactive components. High-performance liquid chromatography was applied to quantify the amount of 18 phenolics, including: gallic acid, 3,4-dihydro benzoic acid, chlorogenic acid, catechin, vanillic acid, caffeic acid, 2,5-dihydro benzoic acid, coumaric acid, syringic acid, frolic acid, chicoric acid, rutin, salicylic acid, rosmarinic acid, quercetin, kaempferol, cinnamic acid, and apigenin. Of these, only apigenin (6.837 ppm) and salicylic acid (1.511 ppm) were identified. The high-performance liquid chromatogram of the extract has been presented in Figure 2.
HPLC chromatogram of the methanolic extract of Malva sylvestris. Two signals (A) and (B) were identified as salicylic acid and apigenin, respectively
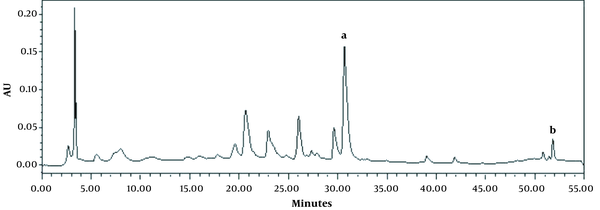
5. Discussion
Biofilm-producing bacteria are inherently resistant to antibacterial drugs, which are the major cause of various infections in human and animals (2). In addition, bacterial biofilms can cause serious problems in the food industry. Nowadays, numerous investigations have focused on the development of nontoxic antibiofilm agents, because such molecules will not lead to drug resistance in the future (5). Herbal derivatives with various bioactive compounds are suitable candidates for the treatment of various infections caused by bacterial biofilms (22).
In this study, the antibacterial and antibiofilm activities of M. sylvestris extract were assessed against some Gram-positive and Gram-negative bacteria. Our results indicated that M. sylvestris extract had antibacterial activity against all the five pathogenic bacteria. These results were obtained by both well diffusion and broth microdilution tests.
In the present study, the inhibition of bacterial growth by M. sylvestris extract was found in a dose-dependent manner. Our findings supported previous results in the literature that the antibacterial activity of this plant is directly related to increased extract concentrations (23).
The results indicated that M. sylvestris methanolic extract inhibited microbial growth with the highest MIC values against S. aureus (21.9 mg/mL) and E. faecalis (32.8 mg/mL). The extract was also active against E. coli, K. pneumoniae (both MIC value of 43.7 mg/mL), and P. aeruginosa (MIC value of 51.9 mg/mL). In a study conducted by Nozohour and Jalilzadeh (24), the ethanolic extract of M. sylvestris could inhibit the growth of E. coli standard strain with the MIC value of 50 mg/mL, which is in line with our finding. Compared to our results, Aminnezhad et al. (25) reported a weaker antimicrobial activity of the ethanolic extract of M. sylvestris against P. aeruginosa with the MIC value of 62.5 mg/mL. However, in a study by Shadid et al. (13), a greater antimicrobial activity was found by the acetonic extract of M. sylvestris against S. aureus, E. coli, and P. aeruginosa with MIC values of 0.125, 12.5, and 3.125, respectively. These differences in antimicrobial activity may be due to the difference in the solvent system applied in these studies.
The results showed that the plant extract had a more effect on Gram-positive bacteria, and the highest antibacterial properties were related to S. aureus. This finding is consistent with the results of other studies in the literature. For instance, Koohsari et al. (26) investigated the antimicrobial activity of six native plants in north of Iran against six Gram-positive (S. aureus, S. epidermidis, and vancomycin-resistant E. faecalis) and Gram-negative (E. coli, Salmonella typhimurium, Shigella dysentery) pathogenic bacteria. They found that Gram-positive strains were more sensitive than Gram- negative strains, and the most sensitive bacterium was S. aureus (26). In another study, the antibacterial activity of 39 methanolic extracts of 25 Australian herbs against two Gram-positive (Bacillus cereus and B. subtilis) and two Gram-negative (P. aeruginosa and Aeromonas hydrophila) was investigated, the results of which confirmed the sensitivity of Gram- positive bacteria (27).
In fact, Gram-negative bacteria showed less sensitivity to plant extracts than Gram-positive bacteria. According to the studies, cell walls of Gram-positive bacteria are more penetrable and sensitive to antimicrobial agents compared with Gram-negative bacteria. The lipopolysaccharides layer and periplasmic space of Gram-negative bacteria may be the reason for their relative resistance to antimicrobials (26). In Gram-positive bacteria, peptidoglycan layers are porous and allow antibiotics, chemical compounds, and even herbal drugs to access the cell membrane.
It is assumed that the antimicrobial activities of the plant should be attributed to the presence of various antimicrobial compounds in the M. sylvestris extract. Naphthoquinones, anthocyanidins, flavonoids, or mucilaginous polysaccharides, that are present in high amounts, are among the most significant compounds of M. sylvestris that have antimicrobial properties (11, 28).
A previous study revealed the antimicrobial effect of anthocyanin extracted from M. sylvestris. Anthocyanin showed promising antimicrobial activity against S. aureus but had no cytotoxic activity against both Aspergillus niger and E. coli. Interestingly, with increasing anthocyanin content, the bacteriostatic activity was increased (29).
Based on GC-MS analysis, the most detected compounds of M. sylvestris extract were 1-heptacosanol (38.4 %), 17-pentatriacontene (19.8%), and 6,9,12,15-docosatetraenoic acid, methyl ester (8.1%). 1-Heptacosanol is a long-chain primary fatty alcohol. As this compound has already been reported to have nematocidal, anticancer, antioxidant, and antimicrobial activities (30-32), some of the antimicrobial properties of M. sylvestris extract may be depend on the presence of 1-heptacosanol. Another most detected compound, 17-pentatriacontene, which has also been identified from the leaves extract of Eichhornia crassipes, indicated anti-inflammatory, anticancer, antibacterial, and antiarthritic properties (33). In a study by Tabaraki et al. (28), the GC-MS analysis of M. sylvestris methanolic extract identified 18 compounds, of which 2-methoxy-4-vinylphenol was the most abundant compound. In another study, Miranda-Martinez et al. (9) investigated the GC-MS analysis of the hydroalcoholic extract of M. sylvestris and observed an abundance of hydrocarbons, which is in line with our findings. In their study, the most abundant compound was α-linolenic acid (30.61%), followed by hexadecanoic acid (12.14%) and octadecanoic acid (8.31%).
M. sylvestris is reported to have high phenols and total flavonoids contents (9). Flavonoids and phenolic compounds are associated with a wide range of health benefits due to their ability to modulate the key functions of cellular enzymes (34). However, the concentrations of flavonoids and phenolic compounds in plants are very sensitive to geographical location and environmental and climate conditions (34).
In the present study, HPLC analysis was performed to quantify the amount of the 18 phenolics, of which only apigenin (6.837 ppm) and salicylic acid (1.511 ppm) were identified. In a study conducted by Daniela et al. (35), HPLC analysis of the M. sylvestris methanolic extract allowed the identification of apigenin, quercetin, kaempferol, genistein, myricetin, and 5,7-dimethoxycoumarin. Benso et al. (36) investigated the HPLC analysis of the ethanol extract of M. sylvestris and identified the bioactive compound rutin. Rutin is a flavonoid and has diverse pharmacological activities, including reducing the risk of chronic diseases and promoting health (36).
Apigenin, as a plant-derived flavonoid, has displayed antifungal, antiparasitic, antiviral, and antibacterial activities (37). The antimicrobial activity of apigenin against Proteus mirabilis, S. Typhi, P. aeruginosa (38), and S. aureus, including the methicillin-resistant and methicillin-sensitive S. aureus (39, 40), has been reported previously. Besides, synergistic interaction has been observed between apigenin and other antibiotics (41). A previous study revealed that cell wall/membrane and nucleic acid processing enzymes may be the main targets of apigenin on bacteria. It affects the type II fatty acid synthetic pathway and D-Alanine, both of which are involved in cell membrane/wall synthesis (37).
The antibacterial activity of salicylic acid, as an organic acid, was also documented previously. Adamczak et al. (42) reported that salicylic acid could strongly inhibit the growth of P. aeruginosa, E. coli, S. aureus, and E. faecalis at the MIC range of 250 - 500 µg/mL. Furthermore, salicylic acid has anti-inflammatory activity and can be applied as a topical antibacterial agent due to its potency to increase exfoliation (43, 44).
As a result, although M. sylvestris extract contains antimicrobial compounds that contribute to the antimicrobial properties of the extract, the simultaneous presence of these compounds may strengthen the plant’s antibacterial effects (45).
A large number of investigations have examined the antimicrobial properties of M. sylvestris derivatives; nonetheless, there is a limited number of studies investigating the antibiofilm potential of this plant against pathogenic bacteria. Furthermore, it is an open gate for future investigations. Bacterial biofilm, because of its high resistance to treatment and the potency to produce nosocomial infections, remains a global threat. More importantly, some biofilm-forming species, such as S. aureus, E. coli, and P. aeruginosa, can cause problems associated with food-borne diseases and food spoilage. Consequently, the inhibition of biofilm formation by natural antimicrobial compounds is expected to be an alternative to traditional chemical sanitizers (46). Accordingly, research for finding new efficient molecules to overcome this problem is recommended (2, 5).
In this research, the bioactivity of M. sylvestris extract was assessed against the biofilms of some pathogenic bacteria. The results (Table 2) showed that the tested extract inhibited biofilm formation in all the tested bacteria. In other words, 87, 40, 40, 87, and 40 mg/mL of M. sylvestris extract inhibited 50% of biofilm formation in E. coli, K. pneumonia, S. aureus, P. aeruginosa, and E. faecalis, respectively.
In a similar study, the antibiofilm activity of M. sylvestris ethanolic extract against Candida albicans biofilm was proven (47). Extracts from this plant may influence biofilm formation through interaction with bacterial adhesion (48), damaging microbial membrane structures (23), inhibition of exopolysaccharide production, inhibition of peptidoglycan synthesis, and/or interference with quorum sensing (QS) (49). Additionally, some of the antibiofilm activity of M. sylvestris extract may be related to the presence of compounds that exert antibiofilm effects via the disruption of cell to cell communications or quorum sensing inhibition (50).
The limitation of the present work is that our study reports the antimicrobial and antibiofilm activity of M. sylvestris crude extract, and the evaluation of the activity of active components in pure form is necessary to understand the reported effect.
5.1. Conclusions
M. sylvestris extract showed antibacterial property against both Gram-negative and Gram-positive strains, and the highest activity was observed against S. aureus. The extract was able to inhibit biofilm formation in the all tested bacteria. Our findings support the traditional use of M. sylvestris in the treatment of various diseases. The results obtained in this study indicated that the methanolic extract of M. sylvestris is a valuable source of pharmaceutical natural products, and it can be used for the development of new therapeutic formulations. Based on the detected antibiofilm activity, which is reported for the first time in the present study, M. sylvestris methanolic extract can be a promising alternative to antibiotics or food additives against biofilm-producing bacteria.
References
-
1.
Alvarez-Ordonez A, Coughlan LM, Briandet R, Cotter PD. Biofilms in Food Processing Environments: Challenges and Opportunities. Annu Rev Food Sci Technol. 2019;10:173-95. [PubMed ID: 30653351]. https://doi.org/10.1146/annurev-food-032818-121805.
-
2.
Stewart PS, Costerton JW. Antibiotic resistance of bacteria in biofilms. Lancet. 2001;358(9276):135-8. [PubMed ID: 11463434]. https://doi.org/10.1016/s0140-6736(01)05321-1.
-
3.
Jamal M, Ahmad W, Andleeb S, Jalil F, Imran M, Nawaz MA, et al. Bacterial biofilm and associated infections. J Chin Med Assoc. 2018;81(1):7-11. [PubMed ID: 29042186]. https://doi.org/10.1016/j.jcma.2017.07.012.
-
4.
Landini P, Antoniani D, Burgess JG, Nijland R. Molecular mechanisms of compounds affecting bacterial biofilm formation and dispersal. Appl Microbiol Biotechnol. 2010;86(3):813-23. [PubMed ID: 20165945]. https://doi.org/10.1007/s00253-010-2468-8.
-
5.
Goode D, Jackson C, Aber K, Shah A, Hensel L. Discovery, Efficacy Testing, and Potential Target Identification of Antibiofilm Compounds. FASEB J. 2018;32(S1). https://doi.org/10.1096/fasebj.2018.32.1_supplement.810.13.
-
6.
Rios JL, Recio MC. Medicinal plants and antimicrobial activity. J Ethnopharmacol. 2005;100(1-2):80-4. [PubMed ID: 15964727]. https://doi.org/10.1016/j.jep.2005.04.025.
-
7.
Kim YG, Lee JH, Gwon G, Kim SI, Park JG, Lee J. Essential Oils and Eugenols Inhibit Biofilm Formation and the Virulence of Escherichia coli O157:H7. Sci Rep. 2016;6:36377. [PubMed ID: 27808174]. [PubMed Central ID: PMC5093407]. https://doi.org/10.1038/srep36377.
-
8.
Gasparetto JC, Martins CA, Hayashi SS, Otuky MF, Pontarolo R. Ethnobotanical and scientific aspects of Malva sylvestris L.: a millennial herbal medicine. J Pharm Pharmacol. 2012;64(2):172-89. [PubMed ID: 22221093]. https://doi.org/10.1111/j.2042-7158.2011.01383.x.
-
9.
Miranda-Martinez M, Sarmiento-TomalÁ GM, ChÓEz-Guaranda IA, GutiÉRrez-GaitÉN YI, Delgado-HernÁNdez R, Carrillo-Lavid G. Pharmacognostic, chemical and mucolytic activity study of Malva pseudolavatera Webb & Berthel. and Malva sylvestris L. (Malvaceae) leaf extracts, grown in Ecuador. Biodiversitas Journal of Biological Diversity. 2020;21(10). https://doi.org/10.13057/biodiv/d211040.
-
10.
Vahabi S, Hakemi-Vala M, Gholami S. In vitro Antibacterial Effect of Hydroalcoholic Extract of Lawsonia inermis, Malva sylvestris, and Boswellia serrata on Aggregatibacter actinomycetemcomitans. Adv Biomed Res. 2019;8:22. [PubMed ID: 31016180]. [PubMed Central ID: PMC6446579]. https://doi.org/10.4103/abr.abr_205_18.
-
11.
Razavi SM, Zarrini G, Molavi G, Ghasemi G. Bioactivity of malva sylvestris L., a medicinal plant from iran. Iran J Basic Med Sci. 2011;14(6):574-9. [PubMed ID: 23493458]. [PubMed Central ID: PMC3586856].
-
12.
Gurbuz I, Ozkan AM, Yesilada E, Kutsal O. Anti-ulcerogenic activity of some plants used in folk medicine of Pinarbasi (Kayseri, Turkey). J Ethnopharmacol. 2005;101(1-3):313-8. [PubMed ID: 16085377]. https://doi.org/10.1016/j.jep.2005.05.015.
-
13.
Shadid KA, Shakya AK, Naik RR, Jaradat N, Farah HS, Shalan N, et al. Phenolic Content and Antioxidant and Antimicrobial Activities of Malva sylvestris L., Malva oxyloba Boiss., Malva parviflora L., and Malva aegyptia L. Leaves Extract. J Chem. 2021;2021:1-10. https://doi.org/10.1155/2021/8867400.
-
14.
Mohammed HJ. Screening of Antibacterial Properties for Some Iraqi Plants Against Salmonella typhimurium. Iraqi J Vet Med. 2011;35(2):28-35. https://doi.org/10.30539/iraqijvm.v35i2.572.
-
15.
Zohra SF, Meriem B, Samira S. Fatty acids profile and antimicrobial activities of the seed oil of Malva sylvestris L. from Algeria. Int J Chem Environ Biol Sci. 2013;1(2):233-5.
-
16.
Cardoso AMR, Cavalcanti YW, de Almeida LDFD, de Lima Pérez ALA, Padilha WWN. Antifungal activity of plant-based tinctures on Candida. RSBO Revista Sul-Brasileira de Odontologia. 2012;9(1):25-30.
-
17.
Behbahani BA, Shahidi F, Yazdi FT, Mortazavi SA, Mohebbi M. Antioxidant activity and antimicrobial effect of tarragon (Artemisia dracunculus) extract and chemical composition of its essential oil. J Food Measure Character. 2017;11(2):847-63. https://doi.org/10.1007/s11694-016-9456-3.
-
18.
Balouiri M, Sadiki M, Ibnsouda SK. Methods for in vitro evaluating antimicrobial activity: A review. J Pharm Anal. 2016;6(2):71-9. [PubMed ID: 29403965]. [PubMed Central ID: PMC5762448]. https://doi.org/10.1016/j.jpha.2015.11.005.
-
19.
Novy P, Davidova H, Serrano-Rojero CS, Rondevaldova J, Pulkrabek J, Kokoska L. Composition and Antimicrobial Activity of Euphrasia rostkoviana Hayne Essential Oil. Evid Based Complement Alternat Med. 2015;2015:734101. [PubMed ID: 26000025]. [PubMed Central ID: PMC4427012]. https://doi.org/10.1155/2015/734101.
-
20.
Sharifi A, Mohammadzadeh A, Zahraei Salehi T, Mahmoodi P. Antibacterial, antibiofilm and antiquorum sensing effects of Thymus daenensis and Satureja hortensis essential oils against Staphylococcus aureus isolates. J Appl Microbiol. 2018;124(2):379-88. [PubMed ID: 29144601]. https://doi.org/10.1111/jam.13639.
-
21.
Proestos C, Sereli D, Komaitis M. Determination of phenolic compounds in aromatic plants by RP-HPLC and GC-MS. Food Chem. 2006;95(1):44-52. https://doi.org/10.1016/j.foodchem.2004.12.016.
-
22.
Lee JH, Park JH, Cho HS, Joo SW, Cho MH, Lee J. Anti-biofilm activities of quercetin and tannic acid against Staphylococcus aureus. Biofouling. 2013;29(5):491-9. [PubMed ID: 23668380]. https://doi.org/10.1080/08927014.2013.788692.
-
23.
Gonelimali FD, Lin J, Miao W, Xuan J, Charles F, Chen M, et al. Antimicrobial Properties and Mechanism of Action of Some Plant Extracts Against Food Pathogens and Spoilage Microorganisms. Front Microbiol. 2018;9:1639. [PubMed ID: 30087662]. [PubMed Central ID: PMC6066648]. https://doi.org/10.3389/fmicb.2018.01639.
-
24.
Nozohour Y, Jalilzadeh G. Antibacterial Activities of Ethanolic Extract of Malva sylvestris L. Against Salmonella enterica and Escherichia coli Isolated from Diarrheic Lambs. Iran J Med Microbiol. 2021;15(1):121-9. https://doi.org/10.30699/ijmm.15.1.121.
-
25.
Aminnezhad S, Kasra KR, Mirzai M, Rashidiani J. Effectiveness of malva sylvestris extract on growth and biofilmformation of Pseudomonas aeruginosa. N Cell Mol Biotech J. 2017;7(28):33-41.
-
26.
Koohsari H, Ghaemi EA, Sadegh Sheshpoli M, Jahedi M, Zahiri M. The investigation of antibacterial activity of selected native plants from North of Iran. J Med Life. 2015;8(Spec Iss 2):38-42. [PubMed ID: 28255395]. [PubMed Central ID: PMC5327717].
-
27.
Cock I. Antibacterial Activity of Selected Australian Native Plant Extracts. Internet J Microbiol. 2008;4(2). https://doi.org/10.5580/8d1.
-
28.
Tabaraki R, Yosefi Z, Asadi GHA. Chemical Composition and Antioxidant Properties of Malva sylvestris L. 2012.
-
29.
Cheng C, Wang Z. Bacteriostasic activity of anthocyanin of Malva sylvestris. J Forestry Res. 2006;17(1):83-5. https://doi.org/10.1007/s11676-006-0020-6.
-
30.
Al-Abd NM, Mohamed Nor Z, Mansor M, Azhar F, Hasan MS, Kassim M. Antioxidant, antibacterial activity, and phytochemical characterization of Melaleuca cajuputi extract. BMC Complement Altern Med. 2015;15:385. [PubMed ID: 26497742]. [PubMed Central ID: PMC4619549]. https://doi.org/10.1186/s12906-015-0914-y.
-
31.
Everlyne IM, Sangilimuthu AY, Darsini DTP. Spectral analyses of the bioactive compounds present in the ethanolic leaf extract of Strobilanthes kunthiana (Nees) T. Anderson ex. Benth. Adv Bio Res. 2015;6(3):65-71.
-
32.
de Oliveira AM, Conserva LM, de Souza Ferro JN, de Almeida Brito F, Lyra Lemos RP, Barreto E. Antinociceptive and anti-inflammatory effects of octacosanol from the leaves of Sabicea grisea var. grisea in mice. Int J Mol Sci. 2012;13(2):1598-611. [PubMed ID: 22408410]. [PubMed Central ID: PMC3291979]. https://doi.org/10.3390/ijms13021598.
-
33.
Kumar D, Karthik M, Rajakumar R. GC-MS analysis of bioactive compounds from ethanolic leaves extract of Eichhornia crassipes (Mart) Solms and their pharmacological activities. 2018.
-
34.
Najjaa H, Abdelkarim BA, Doria E, Boubakri A, Trabelsi N, Falleh H, et al. Phenolic composition of some Tunisian medicinal plants associated with anti-proliferative effect on human breast cancer MCF-7 cells. EuroBiotech J. 2020;4(2):104-12. https://doi.org/10.2478/ebtj-2020-0012.
-
35.
Daniela A, Pichichero E, Canuti L, Cicconi R, Karou D, D’Arcangelo G, et al. Identification of phenolic compounds from medicinal and melliferous plants and their cytotoxic activity in cancer cells. Caryologia. 2013;60(1-2):90-5. https://doi.org/10.1080/00087114.2007.10589552.
-
36.
Benso B, Franchin M, Massarioli AP, Paschoal JAR, Alencar SM, Franco GCN, et al. Anti-inflammatory, anti-osteoclastogenic and antioxidant effects of Malva sylvestris extract and fractions: in vitro and in vivo studies. PLoS One. 2016;11(9). e0162728. https://doi.org/10.1371/journal.pone.0162728.g007.
-
37.
Wang M, Firrman J, Liu L, Yam K. A Review on Flavonoid Apigenin: Dietary Intake, ADME, Antimicrobial Effects, and Interactions with Human Gut Microbiota. Biomed Res Int. 2019;2019:7010467. [PubMed ID: 31737673]. [PubMed Central ID: PMC6817918]. https://doi.org/10.1155/2019/7010467.
-
38.
Basile A, Giordano S, Lopez-Saez JA, Cobianchi RC. Antibacterial activity of pure flavonoids isolated from mosses. Phytochemistry. 1999;52(8):1479-82. [PubMed ID: 10647220]. https://doi.org/10.1016/s0031-9422(99)00286-1.
-
39.
Cushnie TP, Hamilton VE, Lamb AJ. Assessment of the antibacterial activity of selected flavonoids and consideration of discrepancies between previous reports. Microbiol Res. 2003;158(4):281-9. [PubMed ID: 14717448]. https://doi.org/10.1078/0944-5013-00206.
-
40.
Sato Y, Suzaki S, Nishikawa T, Kihara M, Shibata H, Higuti T. Phytochemical flavones isolated from Scutellaria barbata and antibacterial activity against methicillin-resistant Staphylococcus aureus. J Ethnopharmacol. 2000;72(3):483-8. [PubMed ID: 10996290]. https://doi.org/10.1016/s0378-8741(00)00265-8.
-
41.
Cushnie TP, Lamb AJ. Antimicrobial activity of flavonoids. Int J Antimicrob Agents. 2005;26(5):343-56. [PubMed ID: 16323269]. [PubMed Central ID: PMC7127073]. https://doi.org/10.1016/j.ijantimicag.2005.09.002.
-
42.
Adamczak A, Ozarowski M, Karpinski TM. Antibacterial Activity of Some Flavonoids and Organic Acids Widely Distributed in Plants. J Clin Med. 2019;9(1). [PubMed ID: 31906141]. [PubMed Central ID: PMC7019947]. https://doi.org/10.3390/jcm9010109.
-
43.
Sedghi M, Gholi-Toluie S. Influence of Salicylic Acid on the Antimicrobial Potential of Stevia (Stevia rebaudiana Bertoni, Asteraceae) Leaf Extracts against Soybean Seed-Borne Pathogens. Trop J Pharm Res. 2014;12(6). https://doi.org/10.4314/tjpr.v12i6.25.
-
44.
Kantouch A, El-Sayed AA, Salama M, El-Kheir AA, Mowafi S. Salicylic acid and some of its derivatives as antibacterial agents for viscose fabric. Int J Biol Macromol. 2013;62:603-7. [PubMed ID: 24076193]. https://doi.org/10.1016/j.ijbiomac.2013.09.021.
-
45.
Nychas GJE. Natural antimicrobials from plants. New Methods of Food Preservation. Springer; 1995. p. 58-89. https://doi.org/10.1007/978-1-4615-2105-1_4.
-
46.
Orhan-Yanıkan E, da Silva-Janeiro S, Ruiz-Rico M, Jiménez-Belenguer AI, Ayhan K, Barat JM. Essential oils compounds as antimicrobial and antibiofilm agents against strains present in the meat industry. Food Control. 2019;101:29-38. https://doi.org/10.1016/j.foodcont.2019.02.035.
-
47.
Alizadeh F, Khodavandi A, Faraji FS. Malva sylvestris inhibits Candida albicans biofilm formation. J Herbmed Pharmacol. 2017;6(2):62-8.
-
48.
Li XH, Lee JH. Antibiofilm agents: A new perspective for antimicrobial strategy. J Microbiol. 2017;55(10):753-66. [PubMed ID: 28956348]. https://doi.org/10.1007/s12275-017-7274-x.
-
49.
He Z, Wang Q, Hu Y, Liang J, Jiang Y, Ma R, et al. Use of the quorum sensing inhibitor furanone C-30 to interfere with biofilm formation by Streptococcus mutans and its luxS mutant strain. Int J Antimicrob Agents. 2012;40(1):30-5. [PubMed ID: 22578766]. https://doi.org/10.1016/j.ijantimicag.2012.03.016.
-
50.
Manner S, Skogman M, Goeres D, Vuorela P, Fallarero A. Systematic exploration of natural and synthetic flavonoids for the inhibition of Staphylococcus aureus biofilms. Int J Mol Sci. 2013;14(10):19434-51. [PubMed ID: 24071942]. [PubMed Central ID: PMC3821565]. https://doi.org/10.3390/ijms141019434.