Abstract
Background:
Nephrotoxicity is a major side effect of aminoglycoside antibiotics, caused by oxidative damage and inflammation. Fucoidan, a group of sulfated polysaccharides derived from different species of brown algae, are well recognized for their antioxidant and anti-inflammatory activities.Objectives:
In the present study, we aimed to investigate, for the first time, the efficacy of fucoidan extracted from Sargassum angustifolium C. Agardh 1820 against gentamicin-induced nephrotoxicity in rats.Methods:
Twenty-eight male Wistar rats were divided into 4 groups of control, gentamicin (100 mg/kg), and gentamicin plus 50- and 100-mg/kg/day fucoidan pretreatment. In the end, all rats were killed, and then urine, blood, and tissue samples were prepared. Kidney weight (KW), body weight (BW), and 24-hour urine volume, as well as serum creatinine (Cr), blood urea nitrogen (BUN), Cr clearance, and malondialdehyde (MDA) levels and superoxide dismutase (SOD) activity, were measured. Kidney samples were also evaluated for histopathological changes.Results:
Gentamicin significantly increased KW, KW/BW ratio, 24-hour urine volume, serum Cr, MDA, and BUN levels; however, fucoidan pretreatment, especially at a dose of 50 mg/kg, significantly returned these variables near to the control group values. Gentamicin also decreased BW gain, Cr clearance, SOD activity, and the degree of renal tissue damage compared to the control group, while treatment with fucoidan significantly reversed these alterations.Conclusions:
The results show that fucoidan from S. angustifolium C. Agardh 1820 ameliorates gentamicin-induced nephrotoxicity by alleviating oxidative stress and augmenting antioxidant enzymes activity in renal tissue, suggesting the potential use of this fucoidan in a clinical setting.Keywords
1. Background
Aminoglycoside antibiotics are commonly used against a wide variety of systemic infections because of their strong antibacterial effects. Because of complete renal excretion, renal toxicity is one of the most common adverse effects occurring in 10%-25% of patients receiving aminoglycosides drugs (1). However, due to their high efficiency against infections, these drugs are still widely used in the clinic. Several pieces of evidence suggest that the mechanisms of aminoglycosides in the development of renal toxicity are multifactorial. These include hemodynamic changes, interstitial inflammation, and necrosis and apoptosis of tubular epithelial cells. These changes contribute to the reduction of glomerular filtration rate (GFR) and epithelial cell edema, as well as to the elevation of serum urea and creatinine (Cr) levels. The overproduction of reactive oxygen species (ROS) and oxidative stress also contribute to the development of aminoglycoside-induced renal injury (2, 3).
Therefore, using compounds with ROS scavenging activity and antioxidative properties may be an appropriate approach for the prevention and/or treatment of pathologies induced by oxidative stress.
In recent years, substances obtained from marine resources (such as seaweeds) have attracted the attention of researchers due to their ease of access and high bioactivities. Seaweeds contain many bioactive compounds that can potentially be used in many clinical and non-clinical applications. One of these compounds, which exhibit many biological effects, is fucoidan extracted from seaweeds (4). Fucoidans are a family of fucose containing sulfated polysaccharides (5) with extensive nutritional and therapeutic applications. Studies show that fucoidans have antifungal (6), antiviral (7), antidiabetic (8), anti-inflammatory (9), and antioxidant effects besides ROS scavenging activity (10). More than 250 species of different algae have been identified in the coastal lines of the Persian Gulf and Oman Sea (11), but the biological properties of most of them have not yet been discovered. Seaweed Sargassum angustifolium C. Agardh 1820 is a species that dominates in the coastal water of the Persian Gulf, contains high amounts of fucoidan, and exhibits great antioxidant capacity (12-14).
2. Objectives
Given the mechanism of gentamicin-induced nephrotoxicity (which is mainly due to the ROS overproduction and oxidative damage) and the proved antioxidant capacity of fucoidan from seaweed S. angustifolium C. Agardh 1820, the present study aimed to examine the protective effect and mechanism of action of this fucoidan against gentamicin-induced nephrotoxicity in rats.
3. Methods
3.1. Drugs
Gentamicin (as pharmaceutical ampules 80 mg/2 mL) was purchased from Caspian Tamin Pharmaceutical Co, Tehran, Iran. Fucoidan powder was supplied by Persian Gulf Algae Development Technology Co, Bushehr, Iran (patent number: 101428; international classification: A61K36/03).
3.2. Collection and Identification of Sargassum angustifolium C. Agardh 1820
Sargassum angustifolium was collected in March 2019 from the selected coastal areas of Bushehr (ie, Daneshjo Park, Lian Park, and Helileh), Persian Gulf, Iran (28°54'15.8"N 50°49'11.0"E; 28°51'45.6"N 50°51'03.4"E and 28°50'16.4"N 50°52'25.5"E). Collected samples were identified by Persian Gulf Algae Development Technology, Persian Gulf Institute, Persian Gulf University of Bushehr as well as based on a study by Sohrabipour et al. (12). Then, the seaweeds were thoroughly washed in fresh water and dried under shade at room temperature. The dried seaweeds were pulverized to fine particles using a mixer grinder and stored for fucoidan extraction (15). The voucher specimen, coded as 2662 for S. angustifolium, was placed in the herbarium of the School of Pharmacy and Pharmaceutical Sciences of Isfahan University of Medical Sciences, Isfahan, Iran (Figure 1).
Herbarium image of Sargassum angustifolium C. Agardh 1820
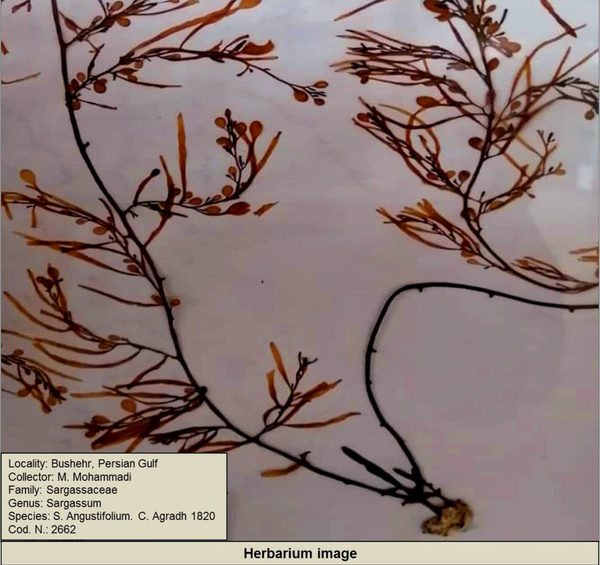
3.3. Animals
Twenty-eight adult male Wistar rats (200 - 250 g) were studied in this study. All procedures carried out according to the National Institutes of Health guidelines for laboratory animals (No. 85-23, revised 1996) and approved by Bushehr University of Medical Sciences Ethics Committee, Bushehr, Iran (code: IR.BPUMS.REC.1398.085; Date: 2019-07-22).
3.4. Experimental Protocol and Treatments
The animals were randomly divided into 4 experimental groups (n = 7 in each group). The control (C) group received intraperitoneal (IP) injection of normal saline; the gentamicin (Gen) group received 100 mg/kg/day of gentamicin by IP injection for 8 consecutive days; the fucoidan pretreated groups of Gen+F50 and Gen+F100 received 50 and 100 mg/kg/day of fucoidan, respectively, 30 minutes before gentamicin injection (Figure 2). Fresh fucoidan solution was prepared daily by dissolving in distilled water and fed orally by gavage. All treatments were performed at the same time, between 8:00 AM and 9:00 AM. The animals that tolerated fucoidan and gentamicin treatments for 8 days and did not develop anuria were included in the study.
Schematic representation of the experimental protocol. Abbreviations: C, control; Gen, gentamicin (100 mg/kg/day; IP for 8 days); Gen+F50, 50 mg/kg/day of fucoidan by oral gavage, 30 minutes before gentamicin administration; Gen+F100, 100 mg/kg/day of fucoidan by oral gavage, 30 minutes before gentamicin administration.
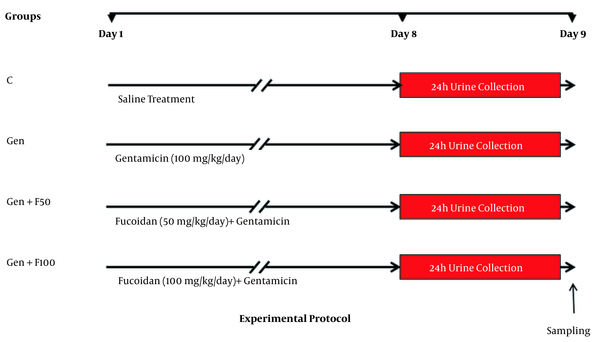
3.5. Sampling and Biochemical Analysis
After the last treatment on the eighth day, the animals were placed individually in a metabolic cage (Razi Rad Co, Tehran, Iran). The 24-hour urine volume was measured and centrifuged at 1000g for 10 minutes at 4°C; then, the samples were stored. Next, the rats were anesthetized by IP injection of ketamine/xylazine (100/10 mg/kg); blood samples were taken directly from the heart, centrifuged at 1000g for 15 minutes, and stored at -20°C to analyze the serum levels of Cr and blood urea nitrogen (BUN). Both kidneys were excised, weighed, and prepared to assess oxidative stress and histopathological alteration.
3.6. Histopathological Examinations
For histopathological evaluation, the right kidney was cut longitudinally into 2 halves, rinsed in ice-cold physiological saline, and fixed in 10% phosphate-buffered formalin. After 48 hours, the tissue paraffin blocks were prepared, and 5 μm-thick sections were stained with hematoxylin and eosin (H&E). The sections were examined under a light microscope to assess histopathological changes by a histopathologist. Tissue changes were assessed in 10 randomly selected fields of each section. The extent of injury was interpreted based on tubular dilatation, cast formation, brush border loss, vascular congestion, infiltration of inflammatory cells, and necrosis.
3.7. Measurement of Tissue Superoxide Dismutase Activity and Malondialdehyde Levels
Renal tissue superoxide dismutase (SOD) activity was evaluated by the pyrogallol assay using the Nasdox-Superoxide Dismutase Assay Kit (Navand Salamat Co, Iran). This assay is based on the amount of pyrogallol autoxidation at alkaline pH, by superoxide anion radicals, as substrate of SOD, in the reaction medium. Presence of SOD in the reaction medium inhibits pyrogallol autooxidation as an indirect indicator of SOD level. The absorbance value of pyrogallol was measured spectrophotometrically at a wavelength of 420 nm, and then the amount of SOD activity was calculated as units/mL. The renal tissue malondialdehyde (MDA) level (as a marker of oxidative stress-induced lipid peroxidation) was assessed by the Nalondi-Lipid Peroxidation Assay Kit (Navand Salamat Co, Iran). This assay is based on the reaction of MDA with thiobarbituric acid at high temperature to produce a pink-color conjugate, which was measured spectrophotometrically at a wavelength of 532 nm (16). The tissue MDA level was calculated as nmol/mL.
3.8. Statistical Analysis
All data were analyzed using SPSS version 19.0 (SPSS Inc, Chicago, Ill, USA) and showed as mean (SEM). Comparison of differences was tested by 1-way analysis of variance (ANOVA) with least significant difference (LSD) post hoc analysis. P < 0.05 was considered statistically significant.
4. Results
4.1. Fucoidan Pretreatment and Gen-Induced Alteration in the Kidney
The results showed that on day 9, the kidneys were clearly pale and enlarged in the gentamicin group compared to the control group. Moreover, severe perirenal edema was observed in the gentamicin group. These findings were correlated with increased kidney weight (KW) and the KW/body weight (BW) ratio. The results of BW measurement showed that gentamicin caused a severe BW loss compared to the control group (Table 1). In gentamicin-treated rats, concomitant 50-mg/kg fucoidan treatment (Gen+F50 group) effectively returned the kidney to the normal appearance, and perirenal edema was obviously disappeared. For standardization, KW was calculated per 100 g of BW. Normalized KW was significantly higher in the gentamicin group compared to the control group (Table 1).
The Effect of Fucoidan on Body Weight, Kidney Weight, Kidney Weight to Body Weight Ratio, and 24-Hour Urine Volume a
Groups | Body Weight (BW) gain (%) | Kidney Weight (KW) (g) | KW/BW Ratio (g/100 g BW) | 24-Hour Urine Volume (mL/100 g BW) |
---|---|---|---|---|
C | 10.4 (2.5) | 1.86 ± 0.07 | 0.75 ± 0.04 | 3.90 ± 0.3 |
Gen | 4.3 (1.0) ** | 2.43 ± 0.16** | 1.09 ± 0.56*** | 8.08 ± 0.8*** |
Gen+F50 | 4.6 (0.62) ** | 2.10 ± 0.09$ | 0.90 ± 0.04*, $$ | 5.70 ± 0.4*, $$ |
Gen+F100 | 1.2 (0.60) *** | 2.47 ± 0.04*** | 1.07 ± 0.01*** | 6.2 ± 0.4** |
4.2. Fucoidan Pretreatment and Gen-Induced Disturbances in the Kidney Function
Gentamicin caused a significant increase in the 24-hour urine volume, showing severe polyuria compared to the control group. Treatment with 50 mg/kg of fucoidan significantly decreased urine volume compared to the gentamicin group, indicating the protective effect of fucoidan against acute tubular injuries, while the decrease caused by the dose of 100 mg was not statistically significant (Table 1).
The results of the biochemical analysis showed that serum Cr and BUN levels significantly increased in gentamicin-treated rats compared to the control group (Figures 3A and B). Moreover, gentamicin significantly reduced the Cr clearance rate (Figure 4). Fucoidan pretreatment at a dose of 50 mg/kg significantly ameliorated the gentamicin-induced increase in serum Cr (Figure 3A), BUN (Figure 3B), and Cr clearance (Figure 4). The results of the Gen+F100 group, however, did not exhibit significant changes in these parameters.
The effect of fucoidan treatment on gentamicin-induced increases in serum creatinine (A) and blood urea nitrogen (B). Abbreviations: C, control; Gen, gentamicin (100 mg/kg/day; IP for 8 days); Gen+F50, 50 mg/kg/day of fucoidan by oral gavage, 30 minutes before gentamicin administration; Gen+F100, 100 mg/kg/day of fucoidan by oral gavage, 30 minutes before gentamicin administration. Values are expressed as mean (SEM). * P < 0.05 and *** P < 0.001 vs control group. $ P < 0.05 and $$ P < 0.01 vs gentamicin group.
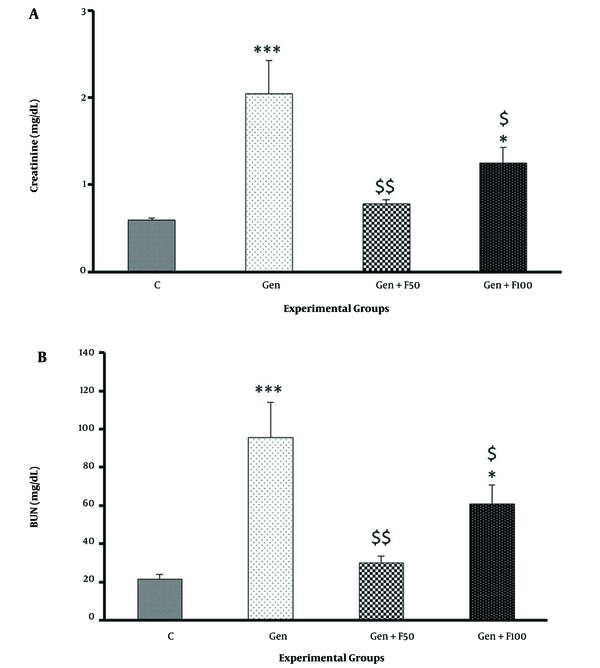
The effect of fucoidan treatment on the gentamicin-induced decrease in creatinine clearance. Abbreviations: C, control; Gen, gentamicin (100 mg/kg/day; IP for 8 days); Gen+F50, 50 mg/kg/day of fucoidan by oral gavage, 30 minutes before gentamicin administration; Gen+F100; 100 mg/kg/day of fucoidan by oral gavage, 30 minutes before gentamicin administration. Values are expressed as mean (SEM). ** P < 0.01 and *** P < 0.001 vs control group. $$ P < 0.01 vs gentamicin group.
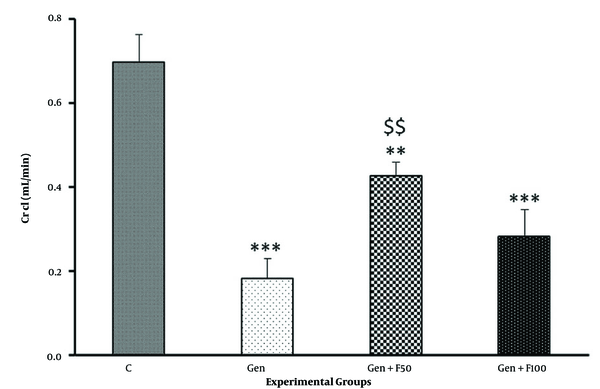
4.3. Fucoidan Pretreatment and Gen-Induced Changes in Renal Tissue Superoxide Dismutase and Malondialdehyde
Gentamicin significantly decreased SOD activity in rat renal tissue homogenate and increased MDA levels. Fucoidan pretreatment at a dose of 50 mg/kg significantly increased SOD activity compared to the gentamicin group (Figure 5A). This treatment also caused a significant decrease in MDA levels vs the gentamicin group (Figure 5B), showing the potent antioxidant effect of fucoidan from S. angustifolium. The results obtained in the Gen+F100 group did not show a significant difference compared to the gentamicin group.
The effect of fucoidan treatment on gentamicin-induced changes in superoxide dismutase activity (A) and malondialdehyde level (B). Abbreviations: C, control; Gen, gentamicin (100 mg/kg/day; IP for 8 days); Gen+F50, 50 mg/kg/day of fucoidan by oral gavage, 30 minutes before gentamicin administration; Gen+F100, 100 mg/kg/day of fucoidan by oral gavage, 30 minutes before gentamicin administration. Values are expressed as mean (SEM). * P < 0.05 vs control group, ** P < 0.01 vs control group, and $ P < 0.05 vs gentamicin group.
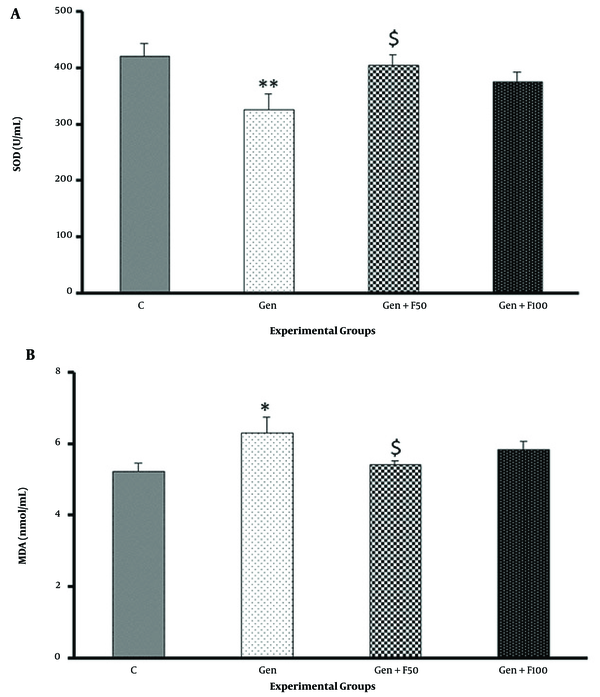
4.4. The Effects of Fucoidan on Gen-Induced Alteration in Kidney Histopathology
The histopathological examination of kidney sections from the control group demonstrated that the renal tissue had a normal appearance in the cortex area (Figure 6A). However, gentamicin-treated rats showed apparent cortical pathological lesions characterized by Bowman’s space enlargement, severe proximal tubular injury, acute tubular necrosis, intratubular cast, recruitments of inflammatory cells, and vascular congestion (Figure 6B).
The effect of fucoidan on gentamicin-induced histopathological changes in the renal cortex stained with hematoxylin and eosin (× 400). Kidney photomicrograph in the control group shows interstitium; glomeruli and tubules are normal (A). Administration of 100 mg/kg/day gentamicin in the Gen group induced structural disturbances characterized by Bowman’s space enlargement (black arrow), severe proximal tubular injury (white arrow), acute tubular necrosis (black bent-up arrow), intratubular cast (white bent-up arrow), inflammatory cells (white curved-up arrow), and vascular congestion (black curved-up arrow) (B). Fucoidan treatment (C and D), especially at a dose of 50 mg/kg (Gen+F50), partially attenuated the glomerular and tubular damages.
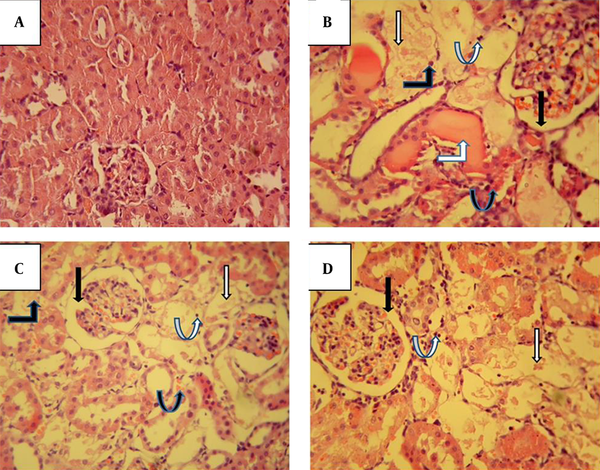
Fucoidan co-treatment, especially at a dose of 50 mg/kg, could partially preserve kidney tissue morphology as demonstrated by attenuated severity of proximal tubular injury and acute tubular injury. Furthermore, the infiltration of inflammatory cells, vascular congestion, and tubular cast were reduced to a great extent by fucoidan treatment (Figures 6C and D).
5. Discussion
Our results showed that fucoidan treatment reversed gentamicin-induced alteration in renal structural and functional parameters. Fucoidan administration also enhanced SOD enzyme activity and decreased MDA levels in kidney tissue.
One of the expected effects of gentamicin administration, as confirmed in previous studies, was a severe decrease in BW gain and an increase in KW and KW/BW ratio. This pattern of changes characterized the occurrence of acute kidney injury (AKI) induced by gentamicin (17). The loss of BW after gentamicin administration may arise from either insufficient water reabsorption due to damage to the renal tubules (which leads to dehydration and weight loss) or reduced food intake due to the general gastrointestinal side effects of aminoglycosides and decreased appetite (18). The KW increase may result from inflammation and renal tissue edema caused by gentamicin-induced acute tubular necrosis (19).
Generally, gentamicin nephrotoxicity is associated with elevation of serum Cr, BUN, and uric acid, as well as a simultaneous increase in urine volume and proteinuria. Consistent with previous studies (17, 19-21), the results of the present study also showed that gentamicin administration induced severe nephrotoxicity, which was manifested by an increase in serum Cr and BUN, indicating glomerular and tubular damage. These disturbances may result from decreased GFR due to the alteration of the glomerular filtration barrier and impaired reabsorptive capacity of tubular epithelial cells, especially in the cortical part of the nephrons (22).
Mechanistically, gentamicin initiates inflammatory processes by activating the nuclear factor-κB (NF-κB) signaling pathway at the site of injury (23), disrupts the respiratory chain, reduces ATP synthesis, and, eventually, by increased production of ROS, leads to oxidative stress and apoptotic cell death (24-27). Gentamicin also enhances lipid peroxidation in the renal cortex, leading to the deterioration of renal structure and function (20). Our results showed that lipid peroxidation increased in the gentamicin group, as evidenced by enhanced tissue MDA levels. This finding has been previously confirmed (28).
On the other hand, 50-mg/kg fucoidan pretreatment imposed a significant decrease in MDA levels, showing the amelioration of gentamicin-induced nephrotoxicity. Furthermore, SOD activity was decreased in renal tissue of gentamicin-treated rats, which was significantly returned to the normal value by 50-mg/kg fucoidan treatment. These findings are in line with the results of a previous study, showing the potent antioxidant property of fucoidan extracted from S. myriocystum against gentamicin-induced nephrotoxicity (29).
Our results also showed that fucoidan co-treatment at a dose of 50 mg/kg significantly decreased perirenal edema, KW, and KW/BW ratio compared to the gentamicin-treated group. These effects appear to be due to the anti-inflammatory effect of fucoidan (30). It has been shown that inflammation worsens the progression of renal diseases by activation of various intracellular targets. NF-κB is one of the transcription factors that can modulate several pro-inflammatory genes.
Studies show that inhibition of the NF-κB signaling pathway ameliorates renal injury, suggesting the importance of inflammation in worsening the severity of renal diseases (31). According to this finding, Jia et al. found that fucoidan treatment suppresses NF-κB activation, decreases the expression of COX-2 and MCP-1, and protects the kidney by reducing interstitial inflammation and fibrosis (32). The anti-inflammatory effects of fucoidan also seem to be mediated by inhibition of TNF-α-NO and COX-2/PGE2 pathways, which are considered the 2 main causes of inflammatory processes (33, 34). In this way, fucoidan reduces the NO and PGE2 production and suppresses the activation of inflammatory processes. Fucoidan also decreases the mobilization of macrophages into the tissue and thus prevents the progression of this process (34).
The results of the present study showed that fucoidan co-treatment induced a statistically significant increase in GFR (estimated by Cr clearance) with a concomitant decrease in serum Cr and BUN. These findings are consistent with Ataman et al., who demonstrated that oral administration of fucoidan attenuated serum Cr and BUN increase induced by gentamicin (35). In addition, Chen et al. showed that 100-mg/kg fucoidan treatment attenuated renal ischemia-reperfusion injury revealed by decreased serum Cr, BUN, lactate dehydrogenase (LDH), and histology ischemic score (36). Likewise, Jia et al. showed that low molecular weight fucoidan improved renal function and protected kidney tissue against albumin overload damage by suppressed expression of pro-inflammatory factors (ie, cyclooxygenase-2 and MCP-1) (32).
In accordance with the change in functional and/or biochemical parameters, the histology results also revealed that gentamicin-treated rats showed apparent pathological lesions characterized by Bowman’s space enlargement, severe proximal tubular injury, acute tubular necrosis, intratubular casts, recruitments of inflammatory cells, and vascular congestion. Fucoidan treatment, especially at a dose of 50 mg/kg, partially preserved kidney tissue morphology as demonstrated by attenuated severity of proximal tubular injury. Furthermore, a marked reduction of inflammatory cells, vascular congestion, and tubular casts were observed in the fucoidan-treated groups.
5.1. Conclusions
The present study, for the first time, demonstrated that pretreatment of rats with fucoidan derived from Persian Gulf algae (ie, S. angustifolium C. Agardh 1820) alleviated gentamicin-induced renal dysfunction and attenuated histopathological changes. Based on the results, it seems that these protective effects may be due to the antioxidant effects of this fucoidan. These findings support the potential use of marine resources for therapeutic purposes in clinical settings.
References
-
1.
Lopez-Novoa JM, Quiros Y, Vicente L, Morales AI, Lopez-Hernandez FJ. New insights into the mechanism of aminoglycoside nephrotoxicity: an integrative point of view. Kidney Int. 2011;79(1):33-45. [PubMed ID: 20861826]. https://doi.org/10.1038/ki.2010.337.
-
2.
Abuelezz SA, Hendawy N, Abdel Gawad S. Alleviation of renal mitochondrial dysfunction and apoptosis underlies the protective effect of sitagliptin in gentamicin-induced nephrotoxicity. J Pharm Pharmacol. 2016;68(4):523-32. [PubMed ID: 27019059]. https://doi.org/10.1111/jphp.12534.
-
3.
Walker PD, Barri Y, Shah SV. Oxidant mechanisms in gentamicin nephrotoxicity. Ren Fail. 1999;21(3-4):433-42. [PubMed ID: 10416224]. https://doi.org/10.3109/08860229909085109.
-
4.
Salehi B, Sharifi-Rad J, Seca AML, Pinto D, Michalak I, Trincone A, et al. Current Trends on Seaweeds: Looking at Chemical Composition, Phytopharmacology, and Cosmetic Applications. Molecules. 2019;24(22). [PubMed ID: 31752200]. [PubMed Central ID: PMC6891420]. https://doi.org/10.3390/molecules24224182.
-
5.
Citkowska A, Szekalska M, Winnicka K. Possibilities of Fucoidan Utilization in the Development of Pharmaceutical Dosage Forms. Mar Drugs. 2019;17(8). [PubMed ID: 31387230]. [PubMed Central ID: PMC6722496]. https://doi.org/10.3390/md17080458.
-
6.
Perez MJ, Falque E, Dominguez H. Antimicrobial Action of Compounds from Marine Seaweed. Mar Drugs. 2016;14(3). [PubMed ID: 27005637]. [PubMed Central ID: PMC4820306]. https://doi.org/10.3390/md14030052.
-
7.
Shi Q, Wang A, Lu Z, Qin C, Hu J, Yin J. Overview on the antiviral activities and mechanisms of marine polysaccharides from seaweeds. Carbohydr Res. 2017;453-454:1-9. [PubMed ID: 29102716]. https://doi.org/10.1016/j.carres.2017.10.020.
-
8.
Gokce G, Haznedaroglu MZ. Evaluation of antidiabetic, antioxidant and vasoprotective effects of Posidonia oceanica extract. J Ethnopharmacol. 2008;115(1):122-30. [PubMed ID: 17977678]. https://doi.org/10.1016/j.jep.2007.09.016.
-
9.
Barbalace MC, Malaguti M, Giusti L, Lucacchini A, Hrelia S, Angeloni C. Anti-Inflammatory Activities of Marine Algae in Neurodegenerative Diseases. Int J Mol Sci. 2019;20(12). [PubMed ID: 31234555]. [PubMed Central ID: PMC6628294]. https://doi.org/10.3390/ijms20123061.
-
10.
Rocha de Souza MC, Marques CT, Guerra Dore CM, Ferreira da Silva FR, Oliveira Rocha HA, Leite EL. Antioxidant activities of sulfated polysaccharides from brown and red seaweeds. J Appl Phycol. 2007;19(2):153-60. [PubMed ID: 19396353]. [PubMed Central ID: PMC2668642]. https://doi.org/10.1007/s10811-006-9121-z.
-
11.
Ghannadi A, Shabani L, Yegdaneh A. Cytotoxic, antioxidant and phytochemical analysis of Gracilaria species from Persian Gulf. Adv Biomed Res. 2016;5:139. [PubMed ID: 27656608]. [PubMed Central ID: PMC5025924]. https://doi.org/10.4103/2277-9175.187373.
-
12.
Sohrabipour J, Nejadsattari T, Assadi M, Rabiei R. The marine benthic algae and seagreasses of the Southern coast of Iran. Iran J Bot. 2004;10(2):83-93.
-
13.
Dawczynski C, Schubert R, Jahreis G. Amino acids, fatty acids, and dietary fibre in edible seaweed products. Food Chem. 2007;103(3):891-9. https://doi.org/10.1016/j.foodchem.2006.09.041.
-
14.
Mehdinezhad N, Ghannadi A, Yegdaneh A. Phytochemical and biological evaluation of some Sargassum species from Persian Gulf. Res Pharm Sci. 2016;11(3):243-9. [PubMed ID: 27499794]. [PubMed Central ID: PMC4962305].
-
15.
Salehpour R, Amrollahi Biuki N, Mohammadi M, Dashtiannasab A, Ebrahimnejad P. The dietary effect of fucoidan extracted from brown seaweed, Cystoseira trinodis (C. Agardh) on growth and disease resistance to WSSV in shrimp Litopenaeus vannamei. Fish Shellfish Immunol. 2021;119:84-95. [PubMed ID: 34560287]. https://doi.org/10.1016/j.fsi.2021.09.005.
-
16.
Zeb A, Ullah F. A Simple Spectrophotometric Method for the Determination of Thiobarbituric Acid Reactive Substances in Fried Fast Foods. J Anal Methods Chem. 2016;2016:9412767. [PubMed ID: 27123360]. [PubMed Central ID: PMC4830699]. https://doi.org/10.1155/2016/9412767.
-
17.
Udupa V, Prakash V. Gentamicin induced acute renal damage and its evaluation using urinary biomarkers in rats. Toxicol Rep. 2019;6:91-9. [PubMed ID: 30581763]. [PubMed Central ID: PMC6297903]. https://doi.org/10.1016/j.toxrep.2018.11.015.
-
18.
Ali BH, Abdel Gayoum AA, Bashir AA. Gentamicin nephrotoxicity in rat: some biochemical correlates. Pharmacol Toxicol. 1992;70(6 Pt 1):419-23. [PubMed ID: 1438019]. https://doi.org/10.1111/j.1600-0773.1992.tb00500.x.
-
19.
Erdem A, Gundogan NU, Usubutun A, Kilinc K, Erdem SR, Kara A, et al. The protective effect of taurine against gentamicin-induced acute tubular necrosis in rats. Nephrol Dial Transplant. 2000;15(8):1175-82. [PubMed ID: 10910441]. https://doi.org/10.1093/ndt/15.8.1175.
-
20.
Mestry SN, Gawali NB, Pai SA, Gursahani MS, Dhodi JB, Munshi R, et al. Punica granatum improves renal function in gentamicin-induced nephropathy in rats via attenuation of oxidative stress. J Ayurveda Integr Med. 2020;11(1):16-23. [PubMed ID: 29555255]. [PubMed Central ID: PMC7125389]. https://doi.org/10.1016/j.jaim.2017.09.006.
-
21.
Medic B, Stojanovic M, Rovcanin B, Kekic D, Skodric SR, Jovanovic GB, et al. Pioglitazone attenuates kidney injury in an experimental model of gentamicin-induced nephrotoxicity in rats. Sci Rep. 2019;9(1):13689. [PubMed ID: 31548602]. [PubMed Central ID: PMC6757036]. https://doi.org/10.1038/s41598-019-49835-1.
-
22.
Randjelovic P, Veljkovic S, Stojiljkovic N, Sokolovic D, Ilic I. Gentamicin nephrotoxicity in animals: Current knowledge and future perspectives. EXCLI J. 2017;16:388-99. [PubMed ID: 28507482]. [PubMed Central ID: PMC5427480]. https://doi.org/10.17179/excli2017-165.
-
23.
Balakumar P, Rohilla A, Thangathirupathi A. Gentamicin-induced nephrotoxicity: Do we have a promising therapeutic approach to blunt it? Pharmacol Res. 2010;62(3):179-86. [PubMed ID: 20434560]. https://doi.org/10.1016/j.phrs.2010.04.004.
-
24.
Cuzzocrea S, Mazzon E, Dugo L, Serraino I, Di Paola R, Britti D, et al. A role for superoxide in gentamicin-mediated nephropathy in rats. Eur J Pharmacol. 2002;450(1):67-76. [PubMed ID: 12176111]. https://doi.org/10.1016/s0014-2999(02)01749-1.
-
25.
Jaikumkao K, Pongchaidecha A, Thongnak LO, Wanchai K, Arjinajarn P, Chatsudthipong V, et al. Amelioration of Renal Inflammation, Endoplasmic Reticulum Stress and Apoptosis Underlies the Protective Effect of Low Dosage of Atorvastatin in Gentamicin-Induced Nephrotoxicity. PLoS One. 2016;11(10). e0164528. [PubMed ID: 27727327]. [PubMed Central ID: PMC5058561]. https://doi.org/10.1371/journal.pone.0164528.
-
26.
Ozbek E, Cekmen M, Ilbey YO, Simsek A, Polat EC, Somay A. Atorvastatin prevents gentamicin-induced renal damage in rats through the inhibition of p38-MAPK and NF-kappaB pathways. Ren Fail. 2009;31(5):382-92. [PubMed ID: 19839839]. https://doi.org/10.1080/08860220902835863.
-
27.
Askari H, Zeinali F, Haghi-Aminjan H, Hafizi SM, Alirezaei A. The protective effects of Ocimum basilicum extract against gentamicin-induced nephrotoxicity in male rats; an anti-inflammatory, anti-oxidative and antiapoptotic action. Immunopathologia Persa. 2019;5(2):e21. https://doi.org/10.15171/ipp.2019.21.
-
28.
Feyzi R, Yari S, Karamian R, Hasanein P. Preventive effect of Stachys lavandulifolia against gentamicin-induced oxidative stress and nephrotoxicity in rats. Comp Clin Path. 2020;29(6):1127-35. https://doi.org/10.1007/s00580-020-03160-6.
-
29.
Vaishnu Devi D, Viswanathan P. Sulphated polysaccharide from Sargassum myriocystum confers protection against gentamicin-induced nephrotoxicity in adult zebrafish. Environ Toxicol Pharmacol. 2019;72:103269. [PubMed ID: 31585298]. https://doi.org/10.1016/j.etap.2019.103269.
-
30.
Apostolova E, Lukova P, Baldzhieva A, Katsarov P, Nikolova M, Iliev I, et al. Immunomodulatory and Anti-Inflammatory Effects of Fucoidan: A Review. Polymers (Basel). 2020;12(10). [PubMed ID: 33066186]. [PubMed Central ID: PMC7602053]. https://doi.org/10.3390/polym12102338.
-
31.
Ding W, Yang L, Zhang M, Gu Y. Chronic inhibition of nuclear factor kappa B attenuates aldosterone/salt-induced renal injury. Life Sci. 2012;90(15-16):600-6. [PubMed ID: 22406301]. https://doi.org/10.1016/j.lfs.2012.02.022.
-
32.
Jia Y, Sun Y, Weng L, Li Y, Zhang Q, Zhou H, et al. Low molecular weight fucoidan protects renal tubular cells from injury induced by albumin overload. Sci Rep. 2016;6:31759. [PubMed ID: 27545472]. [PubMed Central ID: PMC4992848]. https://doi.org/10.1038/srep31759.
-
33.
Kyung J, Kim D, Park D, Yang YH, Choi EK, Lee SP, et al. Synergistic anti-inflammatory effects of Laminaria japonica fucoidan and Cistanche tubulosa extract. Lab Anim Res. 2012;28(2):91-7. [PubMed ID: 22787482]. [PubMed Central ID: PMC3389844]. https://doi.org/10.5625/lar.2012.28.2.91.
-
34.
Jeong J, Hwang SJ, Han MH, Lee D, Yoo JS, Choi I, et al. Fucoidan inhibits lipopolysaccharide-induced inflammatory responses in RAW 264.7 macrophages and zebrafish larvae. Mol Cell Toxicol. 2017;13(4):405-17. https://doi.org/10.1007/s13273-017-0045-2.
-
35.
Ataman N, Mert H, Yildirim S, Mert N. The effect of fucoidan on changes of some biochemical parameters in nephrotoxicity induced by gentamicin in rats. Vet Fak Derg. 2018;65(1):9-14. https://doi.org/10.1501/Vetfak_0000002821.
-
36.
Chen J, Wang W, Zhang Q, Li F, Lei T, Luo D, et al. Low molecular weight fucoidan against renal ischemia-reperfusion injury via inhibition of the MAPK signaling pathway. PLoS One. 2013;8(2). e56224. [PubMed ID: 23418539]. [PubMed Central ID: PMC3572020]. https://doi.org/10.1371/journal.pone.0056224.