Abstract
Background:
The application of natural antimicrobial and antioxidant agents in food and pharmaceutical products has recently become a trend due to the high demand for them from consumers. Postbiotics are bioactive compounds that are produced when the healthy bacteria in the gut ferment fiber.Objectives:
This study aimed to compare the antibacterial and antioxidant properties of postbiotics from 5 different lactic acid bacteria (LAB) including Lactiplantibacillus fermentum, Lactiplantibacillus plantarum, Lactiplantibacillus rhamnosus, Lactobacillus casei, and Lactobacillus acidophilus.Methods:
Two different methods were adopted to obtain postbiotics (M1 and M2). M1 was the simple method in which the centrifugation was employed while in M2 method, ethyl acetate was used to obtain postbiotics. Agar disc diffusion, minimum inhibition concentration, and minimum bactericidal concentration were used to assess the antimicrobial activity of postbiotics. The 2,2-diphenyl-1-picrylhydrazyl (DPPH) test was performed in order for investigating the antioxidant property.Results:
The best results were recorded for L. casei compared to other LABs. Highest values of the agar disc diffusion method were obtained for L. casei. The inhibition zones for Salmonella enterica, Listeria monocytogenes, and Staphylococcus aureus, for examples, were 22 mm, 20 mm, and 19 mm, respectively. The postbiotic of L. casei also exhibited the most potent antioxidant activity among other probiotic bacteria. The data showed that M2 was a more effective method than the other method for acquiring postbiotics.Conclusions:
It was recommended LABs postbiotics should be applied as antioxidant, antimicrobial, and preservatives in food and pharmaceutical industries due to their desired effects and natural characteristics.Keywords
Cell-free Supernatant Probiotic Lactobacillus Antimicrobial Antioxidant
1. Background
Prebiotics, probiotics, and postbiotics have drawn the close attention of researchers over the last decades (1). They are obtained from different sources (e.g., foods (2)) and used in many foods due to their beneficial effects. The shelf life of foods, for instance, is improved by them (3). Probiotics and prebiotics have been used for producing functional foods (4).
A recent growing trend has emerged in the application of postbiotics in various foods due to the consumers’ demand for consumption of foods free from chemical preservative (5). In addition, the application of postbiotics in foods has been found to have some advantages. For examples, postbiotics are more resistant to food processing and they can remain active for longer periods. Application of live probiotic bacteria in food has its own limitations. Live probiotic bacteria are extremely affected by food nature, pH, temperature, etc (6). Currently, massive amounts of postbiotics and postbiotics are applied in food industries (5) in order to produce functional foods, improve shelf life, and enhance the safety of food.
At present, Lactobacillus sp. is the most common bacteria used for producing postbiotics. Many Lactobacillus bacteria (LAB) have been used in foods for various reasons. One of the most common species of Lactobacillus bacteria is Lactiplantibacillus plantarum, which produces several favorable metabolites in foods, such as antimicrobial compounds and vitamins (7). Other beneficial metabolites are also produced by L. plantarum such as hydrogen peroxide, organic acids, bacteriocins, etc. Moreover, L. plantarum functions as an antagonist toward pathogens and spoilage bacteria (7). Although some antibacterial compounds produced by LAB (e.g., bacteriocins) are effective against gram-positive bacteria, other compounds (e.g., organic acids) exhibit antimicrobial effects toward gram-negative bacteria. Herein, LAB has been used for inhibiting the growth of pathogens, such as Salmonella, in the intestine of animals and humans (8).
The postbiotics produced by LAB are generally recognized as safe compounds (GRAS) and, therefore, can be ideally applied in foods (7). Toushik et al. (9) conducted a study on LAB postbiotic to prevent the spoilage of seafood products, and reported that LAB postbiotic significantly increased the shelf life of seafood product and, therefore, suggested that these metabolites should be used as green preservative in seafood. In another study, LAB postbiotics were used to increase the shelf life of chicken drumstick (10). The researchers argued that LAB postbiotic, in addition to improving the shelf life, was effective in killing L. monocytogenes and was able to reduce their number for 1.1 log10 CFU/g. Antimicrobial properties of postbiotics are not only effective in improving the shelf life due to the inhibition of spoilage bacteria, but are also effective in controlling pathogenic bacteria and, thus, enhancing the safety of food.
Moreover, postbiotics obtained from LAB have been reported to show antioxidant activities. This antioxidant properties are attributed to the flavonoids and phenols (11). Incili et al. (10) revealed that some phenolic compounds (e.g., gallic acid, vanilic acid, caffeic acid, and coumaric acid) are the major constituent with radical scavenging activity in postbiotics acquired from LABs.
2. Objectives
There was no earlier study investigating the antimicrobial and antioxidant activities of several lactic acid bacteria. This study, therefore, aimed to exploit LAB metabolites with antimicrobial and antioxidant properties by adopting 2 different methods. This study, moreover, aimed to investigate and compare the antimicrobial and antioxidant activities of 5 different LABs.
3. Methods
3.1. Microorganism
Five different LABs including Lactiplantibacillus fermentum (ATCC 9338), L. plantarum (ATCC 8014), Lactiplantibacillus rhamnosus (ATCC 7469), Lactobacillus casei (ATCC 39392), and Lactobacillus acidophilus (ATCC 4356) were purchased from Iranian Research Organization for Science and Technology, Tehran, Iran. In order to activate the bacteria, they were cultured anaerobically using Whitley Jar Gassing System device in de Man, Rogosa, and Sharpe (MRS) (Merck, Germany) broth medium for 48 h, at 32°C.
3.2. Acquiring Lactobacillus Bacteria Postbiotics
Two different methods were used to obtain metabolites from LABs. In the first method (M1), centrifugation of culture media along with LABs was carried out for 20 m at 4000 g. In this procedure, LAB cells were precipitated at the bottom and supernatant containing extracellular metabolites were extracted. A 0.22 μM membrane was used to filter the supernatant. The metabolites provided by this method are free of any LAB cells (12). In the second method (M2) and after the incubation of LABs in Difco™ Lactobacilli MRS Broth media, ethyl acetate was added to the Erlenmeyer which was, then, placed on a shaker for 3 h to achieve 2 phases. The obtained biphasic solution was filtered through Whatman filter paper. The filtrate was then centrifuged at 4000 g for 20 m (13). The acquired supernatant was transferred to a glass tube and dried at 45°C. In this method, LAB cells were lysed due to the application of ethyl acetate (Merck, Germany), and intracellular metabolites were released into the medium. In fact, the final product contained intracellular and extracellular metabolites of LAB.
3.3. Antimicrobial Activity of 5 Different Lactobacillus Bacteria Metabolites
3.3.1. Agar Disk Diffusion
Agar disc diffusion method was employed to determine the antimicrobial activity of metabolites obtained by 5 different LAB metabolites. Mueller Hinton agar (Merck, Germany) medium was used for performing this test, and 0.5 McFarland of pathogenic bacteria, including Escherichia coli, Listeria monocytogenes, Bacillus cereus, Salmonella enterica, and Staphylococcus aureus were inoculated to the medium. Then 6 mm diameter filter paper disc impregnated with 100 μL of each postbiotics was placed on the agar plates. A caliper was used to record the inhibition zone (14, 15).
3.3.2. Minimum Inhibitory Concentration
Five pathogenic bacteria were cultured in a general medium, such as trypticase soy broth (TSB) (Difco Labo ratories, Detroit, Mich., USA), and used to assess the antimicrobial potency of different postbiotics. Minimum inhibitory concentration (MIC) was measured using microtiter plate assay according to Sevin et al. (16). Postbiotic dilutions (two-fold) ranging from 1.024 to 0.25 mg/mL were used. One hundred microliter of pathogenic bacteria (107 CFU/mL) was added to the postbiotic and placed at 32°C for 24 h. The positive control was pathogenic bacteria without postbiotic, and the negative control was culture media without bacteria and postbiotic. The lowest concentration of each postbiotic preventing the growth of bacteria was considered as MIC.
3.3.3. Minimum Bactericide Concentration
The minimum bactericide concentration (MBC) was carried out according to Torabi Chafriji et al. (17). The antimicrobial characteristic of each postbiotics was tested against 5 different bacteria including E. coli, L. monocytogenes, B. cereus, S. enterica, and S. aureus. The incubation was carried out at 37°C for 24 h. The lack of microbial growth was considered as bactericidal activity after the incubation.
3.4. 2,2-diphenyl-1-picrylhydrazyl Test
Antioxidant properties of each postbiotic was investigated adopting the method proposed by Alkan Turkucar et al. (18). Aliquot 2.5 mL 2,2-diphenyl-1-picrylhydrazyl (DPPH) (1 mM in methanol) was added to 0.5 mL of each postbiotic and shaken. The glass tubes were then placed at 4°C without any light for 1 h. The 517 nm wavelength was used to measure the absorbance. BHT was used as blank. DPPH was calculated using the following formula:
3.5. Statistical Analysis
Statistical analysis of data, such as means ± standard deviation (SD) and one-way analysis of variance of data, was performed using SPSS (SPSS Statistics Software, version 18, Chicago, IL, USA) and MS Excel software. All analyses were performed in 3 replicates.
4. Results
4.1. Agar Disc Diffusion Method
Antimicrobial activity of postbiotics obtained from 5 different LAB were investigated by adopting agar disc diffusion method. Salmonella enterica was the most susceptible bacteria, and 22 mm inhibition zone was recorded due to the postbiotic acquired from L. casei. Overall, L. casei showed the highest antibacterial characteristics. However, L. plantarum was unable to prevent any pathogenic bacteria in the current study. The data obtained by the agar disc diffusion method are presented in Figure 1.
Antimicrobial activity of postbiotics from 5 different lactic acid bacteria against 5 different pathogenic bacteria (M1: First method to obtain postbiotics, M2: Second method to obtain postbiotics)
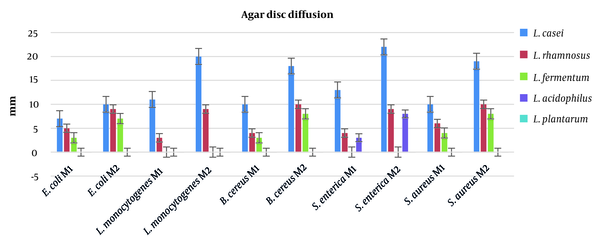
4.2. Minimum Inhibitory Concentration
The MIC is another method to assess the antimicrobial potency of antibacterial agents. The lowest values of MIC were recorded for postbiotics of L. casei, indicating the highest antimicrobial activity. The highest amounts of MIC were determined for L. plantarum, which were unable to inhibit bacterial growth for any pathogen. The data obtained by the MIC method are displayed in Figure 2.
Minimum inhibitory concentration recorded for postbiotics of 5 different lactic acid bacteria against 5 different pathogenic bacteria (M1: First method to obtain postbiotics, M2: Second method to obtain postbiotics)
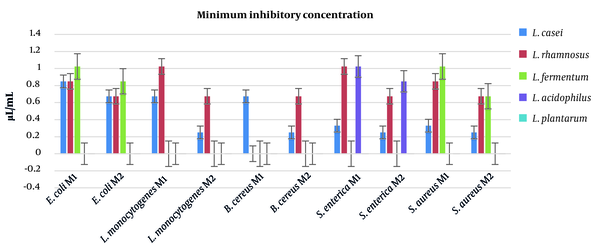
4.3. Minimum Bactericidal Concentration
The MBC for postbiotics are depicted in Figure 3. Lactobacillus acidophilus and L. plantarum were unable to eliminate the pathogenic bacteria even at highest doses of postbiotics (1.024 μL/mL). However, L. casei and L. rhamnosus postbiotics exhibited excellent antimicrobial activity, especially toward E. coli, L. monocytogenes, and S. enterica. Herein, L. casei postbiotic exhibited more antibacterial activity than other bacteria.
Minimum bactericidal concentration determined for postbiotics of 5 different lactic acid bacteria against 5 different pathogenic bacteria (M1: First method to obtain postbiotics, M2: Second method to obtain postbiotics)
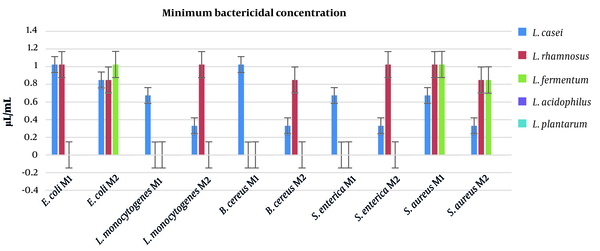
4.4. Antioxidant Activity of the Postbiotics
As shown in Figure 4, L. casei was the most potent postbiotic, followed by L. rhamnosus, L. fermentum, L. acidophilus, and L. plantarum. Lactiplantibacillus plantarum almost showed no effect and exhibited the weakest antioxidant properties.
Antioxidant activity assessed for postbiotics of 5 different lactic acid bacteria against 5 different pathogenic bacteria (M1: First method to obtain postbiotics, M2: Second method to obtain postbiotics)
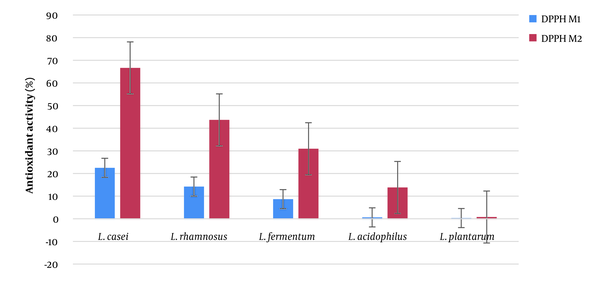
5. Discussion
Postbiotics acquired from probiotic bacteria have recently attracted the close attention of the researchers due to their desirable effects with minimum number of adverse effects. These compounds are natural and pose no risk of bacteremia for immunocompromised individuals (19, 20). Therefore, they have great potential for industrial application associated with food and pharmaceutical products. In the present study, 2 different methods were used to produce postbiotic from 5 different LAB, and their antioxidant and antimicrobial effects were evaluated.
In this study, 3 different tests were carried out to assess the antimicrobial activity of the postbiotics. The postbiotic obtained from L. casei showed the best results for gram-positive and gram-negative bacteria, such as L. monocytogenes and S. enterica. The inhibition zones for L. casei postbiotics were determined to be 20 mm and 22 mm against L. monocytogenes and S. enterica, respectively. Moreover, L. casei postbiotics also showed excellent antimicrobial activity against S. aureus (19 mm) and B. cereus (18 mm). The MIC and MBC generated similar results in the agar disc diffusion test. The lowest amounts of MIC were recorded for L. casei postbiotics toward L. monocytogenes, B. cereus, S. enterica, and S. aureus. These results recorded for postbiotics of M2 proved the efficiency of the second method. Similar results were observed in MBC analysis. The best results in MBC test were reported for postbiotics of L. casei acquired by the second method against L. monocytogenes, B. cereus, S. aureus, and S. enterica (MBC = 0.33 μL/mL). The data obtained by this study revealed that L. plantarum and L. acidophilus were unable to inhibit the growth of pathogenic bacteria, and also exhibited no potential to eliminate the pathogenic bacteria.
Our study results were consistent with the findings from a survey carried out by Azami et al. (21), which reported excellent antibacterial properties of L. casei. Moreover, Campana et al. discovered the exquisite antibacterial activity of L. casei postbiotic (22). They cultivated L. casei in microaerophilic conditions, obtained cell-free supernatant (postbiotic), and investigated its antimicrobial properties against Cronobacter sakazakii. They also argued that L. casei postbiotic was able to eliminate 99% of C. sakazakii after 2 hours, indicating strong antimicrobial activity of the given postbiotics. In contrast, a study on the antibacterial effects of 3 different postbiotics from L. casei, L. salivarius, and L. acidophilus revealed that L. casei was the weakest postbiotic compared to other LABs (6).
The most resistant pathogenic bacterium to L. casei postbiotic was E. coli, which is the most important pathogenic bacteria in foods. Incili et al. (23) conducted a study to investigate the antimicrobial activity of LAB postbiotics against E. coli, and reported that a combination of chitosan and LAB postbiotics had the potential to exert excellent antibacterial effect on E. coli. In another study, postbiotic of L. casei was found effective in controlling pathogens and reducing 99% of E. coli (24).
There are several studies in the literature reporting the antibacterial activity of L. rhamnosus. Shi et al. (25) reported significant antibacterial effect of L. rhamnosus postbiotic on S. enteritidis. They also introduced postbiotics as the next possible generation of antibiotics. Herein, another study revealed the antibacterial effect of L. rhamnosus postbiotics on S. typhimurium (26). Rezaei et al. (27) also demonstrated the antibacterial effects of L. rhamnosusi on L. monocytogenes and Pseudomonas aeruginosa. De Keersmaecker et al. (26) conducted an interesting study to reveal the causality of antibacterial activity of L. rhamnosus postbiotics, and discovered that antibacterial agent produced by L. rhamnosus was non-proteinaceous and heat stable. They also found that this agent acted in acidic pH and was disabled in pH = 6.6. Therefore, they concluded that antibacterial agent of L. rhamnosus present in postbiotic was lactic acid.
Several researchers investigated the desirable effects of postbiotics. Dunand et al. (28), for instance, explored the antibacterial effects of postbiotics and found that fermented milk containing postbiotics of LAB was able to protect mice from S. enterica serovar Typhimurium. Furthermore, Hamad et al. (29) applied postbiotic of L. rhamnosus on poultry meat to inhibit the Clostridium perfringens and documented the significant antibacterial effect of L. rhamnosus postbiotic, concluding that its application on poultry meat may have limited the growth of C. perfringens.
In addition, comparing the first and second methods of obtaining postbiotics revealed that the inhibition zone for each pathogenic bacterium was increased from first method to second method. This may have been due to the additional compounds released from the cytoplasm of lactic acid bacteria. These additional compounds can be bacteriocin-like products and/or peptides with antibacterial activity. Also, the antimicrobial effects of postbiotics obtained by the second method may have been attributed to the presence of hydrogen peroxide and/or organic acids (26).
In the current study, 5 different LABs were used to obtain postbiotics with favorable attributes, such as antimicrobial and antioxidant properties. Antioxidant properties of different postbiotics were analyzed by employing DPPH method. The best results were observed for L. casei, followed by L. rhamnosus, L. fermentum, L. acidophilus, and L. plantarum. Antioxidant activity of LABs had been reported previously. Hamad et al. (29) studied the antioxidant activity of postbiotics obtained from 4 LABs, including L. fermentum, L. rhamnosus, L. acidilactici, and L. delbrueckii, and found the higher antioxidant activity of L. rhamnosus compare to other bacteria. Although Hamad et al. (29) did not assess the antioxidant activity of L. casei, their results were consistent with our study findings. Kumari et al. (30) isolated LABs postbiotics from beetroot and reported antioxidant activity for them. The postbiotics of LABs are the source of antioxidant compounds with different mechanisms of action with radical scavenging potential. Higher antioxidant properties of L. casei in our study may have been due to the higher content of phenolic compounds in its postbiotic. Another possibility is the presence of significantly higher total flavonoids content (29). It has been demonstrated that LABs are capable of producing flavonoid and phenolic compounds, which is strain dependent (31). Production of several phenolic compounds, such as quercetin, ferulic, vanillin, and gallic acid have been reported for LABs (31).
5.1. Conclusions
In this study, 5 different LABs including L. fermentum, L. plantarum, L. rhamnosus, L. casei, and L. acidophilus were selected, and analyzed to determine their antimicrobial and antioxidant activities. To this end, 2 different methods were adopted to obtain postbiotics (M1 and M2). Three different antimicrobial assay methods were implemented (i.e., agar disc diffusion, minimum inhibition concentration, and minimum bactericidal concentration) and DPPH test was performed for investigating the antioxidant property. The data showed that the best postbiotic with antimicrobial and antioxidant characteristics was the one obtained by M2 for L. casei. However, it was recommended that further studies should be conducted in order to determine the antimicrobial and antioxidant compounds in produced postbiotics. It was also suggested that postbiotics should be introduced and applied as the modern antibiotics with minimum adverse effects, which may have been used in food and pharmaceutical industries.
Acknowledgements
References
-
1.
Alemohammad SMA, Noori SMR, Samarbafzadeh E, Noori SMA. The role of the gut microbiota and nutrition on spatial learning and spatial memory: a mini review based on animal studies. Mol Biol Rep. 2022;49(2):1551-63. [PubMed ID: 35028854]. https://doi.org/10.1007/s11033-021-07078-2.
-
2.
Afshari A, Hashemi M, Tavassoli M, Eraghi V, Noori SMA. Probiotic bacteria from 10 different traditional Iranian cheeses: Isolation, characterization, and investigation of probiotic potential. Food Sci Nutr. 2022;10(6):2009-20. [PubMed ID: 35702287]. [PubMed Central ID: PMC9179165]. https://doi.org/10.1002/fsn3.2817.
-
3.
López de Lacey AM, López-Caballero ME, Montero P. Agar films containing green tea extract and probiotic bacteria for extending fish shelf-life. LWT Food Sci Technol. 2014;55(2):559-64. https://doi.org/10.1016/j.lwt.2013.09.028.
-
4.
Misra S, Pandey P, Mishra HN. Novel approaches for co-encapsulation of probiotic bacteria with bioactive compounds, their health benefits and functional food product development: A review. rends Food Sci Technol. 2021;109:340-51. https://doi.org/10.1016/j.tifs.2021.01.039.
-
5.
Hamad G, Ombarak RA, Eskander M, Mehany T, Anees FR, Elfayoumy RA, et al. Detection and inhibition of Clostridium botulinum in some Egyptian fish products by probiotics cell-free supernatants as bio-preservation agents. Lwt. 2022;163. https://doi.org/10.1016/j.lwt.2022.113603.
-
6.
Moradi M, Mardani K, Tajik H. Characterization and application of postbiotics of Lactobacillus spp. on Listeria monocytogenes in vitro and in food models. Lwt. 2019;111:457-64. https://doi.org/10.1016/j.lwt.2019.05.072.
-
7.
Arena MP, Silvain A, Normanno G, Grieco F, Drider D, Spano G, et al. Use of Lactobacillus plantarum Strains as a Bio-Control Strategy against Food-Borne Pathogenic Microorganisms. Front Microbiol. 2016;7:464. [PubMed ID: 27148172]. [PubMed Central ID: PMC4829616]. https://doi.org/10.3389/fmicb.2016.00464.
-
8.
Ruvalcaba-Gomez JM, Villagran Z, Valdez-Alarcon JJ, Martinez-Nunez M, Gomez-Godinez LJ, Ruesga-Gutierrez E, et al. Non-Antibiotics Strategies to Control Salmonella Infection in Poultry. Animals (Basel). 2022;12(1). [PubMed ID: 35011208]. [PubMed Central ID: PMC8749512]. https://doi.org/10.3390/ani12010102.
-
9.
Toushik SH, Park JH, Kim K, Ashrafudoulla M, Senakpon Isaie Ulrich M, Mizan MFR, et al. Antibiofilm efficacy of Leuconostoc mesenteroides J.27-derived postbiotic and food-grade essential oils against Vibrio parahaemolyticus, Pseudomonas aeruginosa, and Escherichia coli alone and in combination, and their application as a green preservative in the seafood industry. Food Res Int. 2022;156:111163. [PubMed ID: 35651029]. https://doi.org/10.1016/j.foodres.2022.111163.
-
10.
Incili GK, Karatepe P, Akgol M, Gungoren A, Koluman A, Ilhak OI, et al. Characterization of lactic acid bacteria postbiotics, evaluation in-vitro antibacterial effect, microbial and chemical quality on chicken drumsticks. Food Microbiol. 2022;104:104001. [PubMed ID: 35287820]. https://doi.org/10.1016/j.fm.2022.104001.
-
11.
Asghari Ozma M, Abbasi A, Sabahi S. Characterization of Postbiotics Derived from Lactobacillus paracasei ATCC 55544 and its Application in Malva sylvestris Seed Mucilage Edible Coating to the Improvement of the Microbiological, and Sensory Properties of Lamb Meat During Storage. Biointerface Res Appl Chem. 2022;13(3). https://doi.org/10.33263/briac133.267.
-
12.
Bungenstock L, Abdulmawjood A, Reich F. Suitability of lactic acid bacteria and deriving antibacterial preparations to enhance shelf-life and consumer safety of emulsion type sausages. Food Microbiol. 2021;94:103673. [PubMed ID: 33279060]. https://doi.org/10.1016/j.fm.2020.103673.
-
13.
Amin M, Moradi Choghakabodi P, Alhassan Hamidi M, Najafian M, Farajzadeh Sheikh A. In vitro antimicrobial activities of metabolites from vaginal Lactobacillus strains against Clostridium perfringens isolated from a woman's vagina. J Chin Med Assoc. 2017;80(1):29-33. [PubMed ID: 27751758]. https://doi.org/10.1016/j.jcma.2016.04.009.
-
14.
Nafis A, Iriti M, Ouchari L, El Otmani F, Marraiki N, Elgorban AM, et al. New Insight into the Chemical Composition, Antimicrobial and Synergistic Effects of the Moroccan Endemic Thymus atlanticus (Ball) Roussine Essential Oil in Combination with Conventional Antibiotics. Molecules. 2021;26(19). [PubMed ID: 34641393]. [PubMed Central ID: PMC8510371]. https://doi.org/10.3390/molecules26195850.
-
15.
Mohammadi R, Moradi M, Tajik H, Molaei R. Potential application of postbiotics metabolites from bioprotective culture to fabricate bacterial nanocellulose based antimicrobial packaging material. Int J Biol Macromol. 2022;220:528-36. [PubMed ID: 35988726]. https://doi.org/10.1016/j.ijbiomac.2022.08.108.
-
16.
Sevin S, Karaca B, Haliscelik O, Kibar H, Omeroglou E, Kiran F. Postbiotics secreted by Lactobacillus sakei EIR/CM-1 isolated from cow milk microbiota, display antibacterial and antibiofilm activity against ruminant mastitis-causing pathogens. Ital J Anim Sci. 2021;20(1):1302-16. https://doi.org/10.1080/1828051x.2021.1958077.
-
17.
Torabi Chafjiri F, Hashemabadi D, Kaviani B, Asadpour L. Detecting the Active Ingredients in the Extract and Essential Oil of the Ornamnetal and Medicinal Plant Species Rue (Ruta graveolens L.) and Studying their Antimicrobial Activity. Journal of Ornamental Plants. 2022.
-
18.
Alkan Turkucar S, Aktas Karacelik A, Karakose M. Phenolic compounds, essential oil composition, and antioxidant activity of Angelica purpurascens (Ave-Lall.) Gill. Turk J Chem. 2021;45(3):956-66. [PubMed ID: 34385879]. [PubMed Central ID: PMC8326465]. https://doi.org/10.3906/kim-2101-28.
-
19.
Hawar S, Vevers W, Karieb S, Ali BK, Billington R, Beal J. Biotransformation of patulin to hydroascladiol by Lactobacillus plantarum. Food Control. 2013;34(2):502-8. https://doi.org/10.1016/j.foodcont.2013.05.023.
-
20.
Yoon SJ, Yu M, Sim GS, Kwon ST, Hwang JK, Shin JK, et al. Screening and characterization of microorganisms with fibrinolytic activity from fermented foods. J Microbiol Biotechnol. 2002;12(4):649-56.
-
21.
Azami S, Arefian E, Kashef N. Postbiotics of Lactobacillus casei target virulence and biofilm formation of Pseudomonas aeruginosa by modulating quorum sensing. Arch Microbiol. 2022;204(2):157. [PubMed ID: 35106661]. https://doi.org/10.1007/s00203-022-02770-8.
-
22.
Campana R, Federici S, Ciandrini E, Manti A, Baffone W. Lactobacillus spp. inhibit the growth of Cronobacter sakazakii ATCC 29544 by altering its membrane integrity. J Food Sci Technol. 2019;56(8):3962-7. [PubMed ID: 31413422]. [PubMed Central ID: PMC6675796]. https://doi.org/10.1007/s13197-019-03928-x.
-
23.
Incili GK, Karatepe P, Akgol M, Tekin A, Kanmaz H, Kaya B, et al. Impact of chitosan embedded with postbiotics from Pediococcus acidilactici against emerging foodborne pathogens in vacuum-packaged frankfurters during refrigerated storage. Meat Sci. 2022;188:108786. [PubMed ID: 35278973]. https://doi.org/10.1016/j.meatsci.2022.108786.
-
24.
Peng M, Reichmann G, Biswas D. Lactobacillus casei and its byproducts alter the virulence factors of foodborne bacterial pathogens. J Funct Foods. 2015;15:418-28. https://doi.org/10.1016/j.jff.2015.03.055.
-
25.
Shi S, Gong L, Yu H, He G, Zhang J, Han Y, et al. Antagonistic activity and mechanism of Lactobacillus rhamnosus SQ511 against Salmonella enteritidis. 3 Biotech. 2022;12(6):126. [PubMed ID: 35573802]. [PubMed Central ID: PMC9095802]. https://doi.org/10.1007/s13205-022-03176-5.
-
26.
De Keersmaecker SC, Verhoeven TL, Desair J, Marchal K, Vanderleyden J, Nagy I. Strong antimicrobial activity of Lactobacillus rhamnosus GG against Salmonella typhimurium is due to accumulation of lactic acid. FEMS Microbiol Lett. 2006;259(1):89-96. [PubMed ID: 16684107]. https://doi.org/10.1111/j.1574-6968.2006.00250.x.
-
27.
Rezaei Z, Khanzadi S, Salari A. Biofilm formation and antagonistic activity of Lacticaseibacillus rhamnosus (PTCC1712) and Lactiplantibacillus plantarum (PTCC1745). AMB Express. 2021;11(1):156. [PubMed ID: 34825290]. [PubMed Central ID: PMC8617238]. https://doi.org/10.1186/s13568-021-01320-7.
-
28.
Dunand E, Burns P, Binetti A, Bergamini C, Peralta GH, Forzani L, et al. Postbiotics produced at laboratory and industrial level as potential functional food ingredients with the capacity to protect mice against Salmonella infection. J Appl Microbiol. 2019;127(1):219-29. [PubMed ID: 30973185]. https://doi.org/10.1111/jam.14276.
-
29.
Hamad GM, Abdelmotilib NM, Darwish AMG, Zeitoun AM. Commercial probiotic cell-free supernatants for inhibition of Clostridium perfringens poultry meat infection in Egypt. Anaerobe. 2020;62:102181. [PubMed ID: 32092413]. https://doi.org/10.1016/j.anaerobe.2020.102181.
-
30.
Kumari VBC, Huligere SS, Ramu R, Naik Bajpe S, Sreenivasa MY, Silina E, et al. Evaluation of Probiotic and Antidiabetic Attributes of Lactobacillus Strains Isolated From Fermented Beetroot. Front Microbiol. 2022;13:911243. [PubMed ID: 35774469]. [PubMed Central ID: PMC9237538]. https://doi.org/10.3389/fmicb.2022.911243.
-
31.
Rupasinghe HPV, Parmar I, Neir SV. Biotransformation of Cranberry Proanthocyanidins to Probiotic Metabolites by Lactobacillus rhamnosus Enhances Their Anticancer Activity in HepG2 Cells In Vitro. Oxid Med Cell Longev. 2019;2019:4750795. [PubMed ID: 31316718]. [PubMed Central ID: PMC6604286]. https://doi.org/10.1155/2019/4750795.