Abstract
Background:
Eryngium is a genus with 274 species, which belongs to the Umbelliferae family. The nine species of this genus are endemic to Iran. The existence of terpenoids, saponins, flavonoids, and steroid components in most Eryngium species is the reason behind their anti-inflammatory, anti-cancer, and especially, anti-diabetic effects among the well-known medicinal plants.Objectives:
This study aimed to evaluate the effects of anti-diabetic features of Eryngium thyrsoideum and caucasicum on streptozotocin (STZ)-induced diabetic mice.Methods:
The extract of the plants’ dried samples was prepared by using 70% ethanol solvent and adopting the maceration method. The mice were divided into eight groups, and the mice with a high blood glucose of more than 200 mg/dL were assigned to diabetic groups. Diabetic animals received different doses of the extract orally or through intraperitoneal (IP) injection for eighteen days. The animals were anesthetized by IP injection of ketamine and xylazine, and serum samples were isolated.Results:
The results showed that hydroalcoholic extract of E. thyrsoideum significantly reduced the blood sugar level in diabetic groups receiving the extract (oral and IP) in low (100 mg/kg) and high (300 mg/kg) doses. Overall, oral glucose tolerance decreased significantly after two hours. Coincidental management of STZ and 300 mg/kg of the extract consumed orally reduced the blood sugar level remarkably. Serum malondialdehyde (MDA) and serum insulin levels significantly decreased and increased, respectively, in the diabetic groups receiving low and high doses (oral and IP) of the E. thyrsoideum extract. In the case of E. caucasicum, no significant effect in terms of reducing blood sugar and other factors was observed.Conclusions:
In sum, receiving 100 mg/kg and 300 mg/kg of hydroalcoholic extract (oral and IP) decreased the blood sugar level, while receiving oral glucose tolerance, serum MDA, and serum insulin caused a significant increase.Keywords
Blood Sugar Eryngium thyrsoideum Eryngium caucasicum Streptozotocin OGTT Malondialdehyde Insulin Level
1. Background
Diabetes mellitus (DM) is one of the crucial endocrine diseases, classified as a chronic metabolic disorder. The prominent feature of this disorder is hyperglycemia, which causes symptoms such as atherosclerosis, stroke, peripheral vascular disease, polyuria, polydipsia, polyphagia, retinopathy, neuropathy, nephropathy, glaucoma and if left untreated, blindness (1-3). The prevalence of DM became an epidemic, with 171 million infected people worldwide in 2000, and it is predicted that it will reach 629 million in 2045 (4). Risk factors that increase the susceptibility to DM include high blood pressure, polycystic ovary syndrome (PCO), overweight, age and gender, inactivity, family history, and so forth (5, 6). Due to the high cost of medicines and adverse side effects of chemical drugs, the application of herbal medicines has been recently suggested as an alternative treatment. Many reports have indicated the effectiveness of various types of herbs in treating diabetes therapy. Among the plants with medicinal properties, species of the genus Eryngium have a useful role in lowering blood sugar. The given plants are widespread throughout northern and central parts of Iran (7). Due to their compounds such as flavonoids (8), coumarin (9), saponin (10), tannin (11), caffeic acid (12), chlorogenic acid (13), fatty acids, and terpenoids (14), these plants have several pharmacological effects, which can be antimicrobial (14), anti-inflammatory (15), and antioxidant (16), etc.
2. Objectives
No study had investigated the significance of medicinal herbs in diabetes therapy and the anti-diabetic effects of E. caucasicum and E. thyrsoideum; therefore, this study aimed to evaluate the anti-diabetic effects of the extract from these plants on diabetic mice.
3. Methods
3.1. Provision of Plant Samples and Plant Powders
Eryngium caucasicum and E. thyrsoideum were collected from the Maragheh region, Iran, in August 2016. After the identification, the herbarium samples with numbers TBZ FPH 1250 and 1251 were deposited by the herbarium of Tabriz University of Medical Sciences. The shoot parts of the plants were dried in the open-air condition and converted to a fine powder using an electric mill. The obtained powder was extremely light and had a very low density.
3.2. Provision of Plant Extraction
Extraction was carried out by adopting the maceration (soaking) method. First, 250 grams of plant shoot powder were poured into a large, wide-mouthed Erlenmeyer flask and added 70% ethanol equivalent; then, the contents of the Erlenmeyer were stirred well to mix the powder evenly into the solvent. Afterwards, the Erlenmeyer head was covered with parafilm and placed away from sunlight, and the contents of the Erlenmeyer were stirred every 24 hours. When the solvent color was fixed, this proceeding was stopped, and the supernatant was filtered by a filter paper; then, the resulting extract solvent was dried by using an evaporator under low pressure and temperature, and kept in the freezer for testing.
3.3. Animals
This study was performed in several steps, including the injection of streptozotocin (STZ), extracts gavage and injection, blood sample collection, as well as mensuration of serum insulin and malondialdehyde (MDA). To this end, eighty male NMRI mice were purchased from Pasteur Institute, Iran. The mice were twelve weeks old with a weight range of 30 g. They were kept under standard conditions, including 12h light-dark, room temperature (20°C) with 60 ± 10% humidity, and were provided with suitable nutrition until the initiation of the tests. Then they were randomly divided into eight groups (n = 6), including group 1: Control group that received only water and food and experienced no intervention; group 2: Diabetic control group that received only STZ without medication; group 3: Diabetic group in which a low dose of extracts (100 mg/kg) was injected intraperitoneally; group 4: Diabetic group in which a high dose of the extracts (300 mg/kg) was injected intraperitoneally (17); group 5: Diabetic group that received a low dose of extracts (100 mg/kg) by gavage (oral); group 6: Diabetic group in which 300 mg/kg of extracts was gavaged; group 7: Control group in which 300 mg/kg of extracts was gavaged; and group 8: The group that received STZ and gavaged with 300 mg/kg of extracts simultaneously (for five days) (17-19).
3.4. Preparation of STZ
STZ is a powder which cannot be injected intraperitoneally directly; therefore, sodium citrate dihydrate buffer should be used to dissolve STZ in order for performing the intraperitoneal (IP) injection of STZ. Each mice needs 150 mg/kg of STZ. Since STZ is sensitive to light, all these steps should be followed in a dark place until injection (20, 21).
3.5. Stimulation of Diabetes in Mice by STZ
First, the mice were weighed, and their blood sugar was measured. Then, 150 mg/kg of STZ was used for each mouse based on his weight (20, 21). The measurement of blood sugar was carried out using mice’s tails vein. In addition, STZ was injected after disinfection of mice tails with 70% ethanol. After three days, the monitored mice were weighed, and their blood sugar was re-measured; then, the mice with more than 200 mg/dL of blood sugar were assigned to diabetic groups, and the blood glucose of the diabetic mice was measured every three days (22).
3.6. Treatment of Diabetic Mice by Extract in IP/Oral form and Their Surgery
Diabetic mice (i.e., groups 3, 4, 5, and 6) received the extracts for 18 days, and were anesthetized using 80 - 100 mg/kg ketamine and 3 - 10 mg/kg xylazine on the 21st day (23-25). The anesthetized mice were checked by adopting the cervical dislocation method to ensure the vital signs stop after anesthesia (26). The mice were placed on their back, then their forelimbs were stretched out using a pin on their front feet. A deep incision was made in the armpit to create a cupped area. Afterwards, the blood vessels were cut with a scalpel, and the blood was collected as it pooled. The blood was centrifuged at 4500 rpm and 4°C for 30 minutes and refrigerated at 4°C for two hours, then the serum of blood samples was kept at -70°C (27).
3.7. Oral Glucose Tolerance (OGTT) Test
On the 18th day, the mice were gavaged 2 g/kg glucose after 12h of fasting. Blood samples were drawn from the tail vein at zero (before using glucose) and 30, 60, and 120 min after using the glucose (28).
3.8. Evaluation of Serum Insulin Levels
Enzyme-linked immunosorbent assay (ELISA) was carried out for measuring the samples of serum insulin using a Shibayagi kit. Biotin conjugated to insulin monoclonal antibody was added in coated wells, including 10 µ serum samples for 2 h at room temperature. After washing the wells with wash buffer, streptavidin in conjugation with horseradish peroxidase (HRP) was added and incubated for 30 min. After washing again, chromogen reagent was added to react with streptavidin-conjugated HRP residue for 30 min, then the reaction was halted by adding the solution. The absorption rate was measured at 450 nm using a microplate reader (Bio-Rad, Hercules, CA, USA) (29).
3.9. Measuring of MDA Level
The level of MDA was evaluated using the colorimetry method and thiobarbutyric acid reagent (TBARS) at 350 nm absorption rate according to the manufacturer’s protocol (Jiancheng Institute of Bioengineering Company, Nanjing, China) (30).
3.10. Measuring of Serum Total Antioxidant Capacity (TAC)
The serum total antioxidant capacity (TAC) was measured in plasma samples and assessed using the ferric reducing ability of plasma (FRAP) method. This method is based on the principle of the ferric-tripyridyltriazine complex reduction to the ferrous form by the antioxidants of a sample and causes the development of an intense blue color, which is spectrophotometrically measurable at 593 nm. This change in absorbance is related to the antioxidant capacity of the samples (31).
3.11. Statistical Analysis of Data
Data were represented as mean ± SD (standard deviations) and analyzed using one-way ANOVA and post-hoc Tukey. The P < 0.05 was considered a significant variance.
4. Results
4.1. Evaluation of the Blood Sugar Change Rates
No remarkable changes were observed in blood sugar rates in the control group during the test. The extract of E. caucasicum was found ineffective in decreasing blood sugar or increasing blood insulin after repeating the experiments for several times (data are not shown). Therefore, the next experiments on E. caucasicum were halted, and the experiments on extract of E. thyrsoideum were carried out. As shown in Figure 1, the blood sugar level of the control group gavaged with 300 mg/kg extract did not change significantly in comparison with that of the control group. The blood sugar level in the diabetic control group was increased from the 3rd day onwards, and this increase was significantly different from that recorded from the 6th day onwards (P < 0.001). Blood glucose in the diabetic group treated with 100 mg/kg extract by gavage, compared to that in the diabetic control group, significantly decreased on days 9th (P < 0.05), 12th to 15th (P < 0.01), and 18th (P < 0.05), and it continued to decrease until the last days (P < 0.01). The blood sugar level of the diabetic group treated with 300 mg/kg extract by gavage, compared to that of the diabetic control group, showed a considerable decrease on days 12th (P < 0.05), and this decrease became significant from the days 12th until the last day of the test (P < 0.001). Our finding revealed a significant decrease in blood glucose levels of the diabetic group treated with the injection of 100 mg/kg extract on days 12th and 15th (P < 0.001), 18th (P < 0.01), and 21st (P < 0.05). The blood glucose level of the diabetic group treated with an injection of 300 mg/kg extract, compared to that of the diabetic control group, was significantly reduced on days 12th (P < 0.01), 15th, 18th (P < 0.001), and the 21st (P < 0.01).
Alterations in blood sugar in control and diabetic groups (the data in the chart is reported as mean ± SEM).
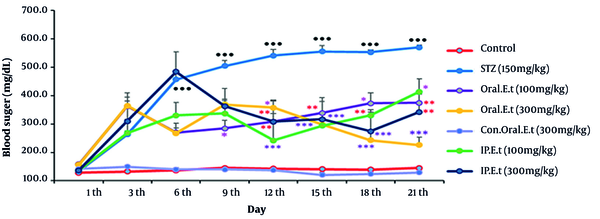
4.2. Blood Sugar Changes in Coincidental Management of STZ and 300 mg/kg Extract
According to our findings about coincidental management of STZ at a dose of 150 mg/kg by IP injection and extract (300 mg/kg) by gavage (oral), the blood sugar level of the samples was significantly reduced compared to that of the diabetic group on the 5th day (Figure 2).
Alterations in blood sugar level of samples during coincidental management of STZ and 300 mg/kg extract by gavage (the data in the chart is reported as mean ± SEM).
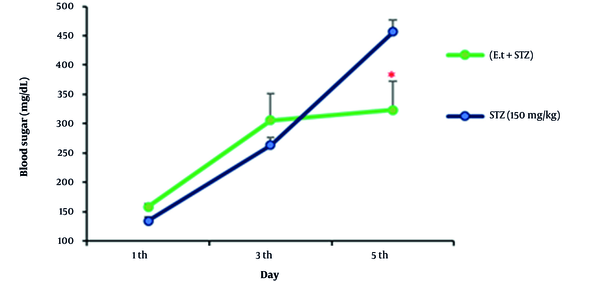
4.3. Measurement of the Weight Changes
The weights of the control group and the control group treated with 300 mg/kg extract by gavage increased during the experiment (Figure 3). In addition, the diabetic control group’s weight decreased significantly on the 3rd day (P < 0.05). The weight of diabetic group treated with 100 mg/kg (low range) and 300 mg/kg (high range) extracts by gavage decreased during the experiment. The weight of diabetic group treated with the low dose extract (100 mg/kg) by IP injection decreased gradually during the test. Finally, a significant reduction was observed in the weight of the diabetic group treated with 300 mg/kg extract by gavage on the 3rd day compared to that of the diabetic control group, and the weight continued to reduce during the test (P < 0.05).
Weight variation in control and diabetic groups (the data in the chart is reported as mean ± SEM).
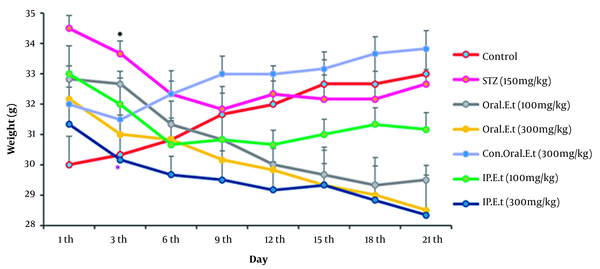
4.4. Weight Alterations in Coincidental Management of STZ and Extract with a Dose of 300 mg/kg
No changes were detected in the samples’ weights during coincidental management of STZ at a dose of 150 mg/kg by IP injection and extract at a dose of 300 mg/kg by gavage (oral), compared to the control (Figure 4).
Weight variation of samples during coincidental management of STZ and 300 mg/kg extract by gavage (the data in the chart is reported as mean ± SEM).
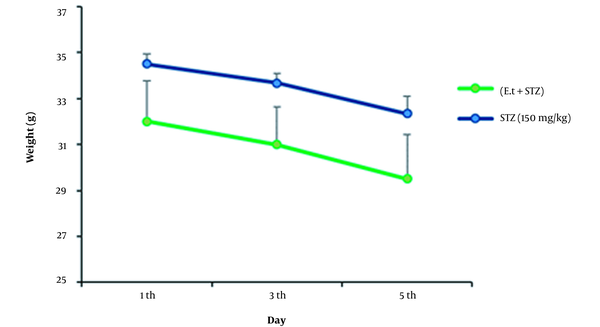
4.5. Alterations in Blood Sugar on the Oral Glucose Tolerance Test
Blood sugar levels in the control group and the control group treated with 300 mg/kg extract by gavage increased 30 min after receiving the oral glucose, but they decreased slowly over time. Blood glucose level significantly increased in the diabetic control group 30 min after receiving the oral glucose in comparison with that in the control group (P < 0.001). No significant variation was observed in blood sugar level of the diabetic groups treated with low and high-dose extract by IP injection 30 min after receiving the oral glucose; however, a significant decrease was detected in blood sugar level of these groups after the initiation of the test (P > 0.001). The blood sugar level increased in diabetic groups treated with low and high doses of the extract by gavage 30 min after receiving the oral glucose, but it decreased gradually over time. Finally, a significant decrease was discovered in blood sugar levels of these groups at 120 min (P < 0.001) (Figure 5).
Changes in blood sugar in mice during the Oral Glucose Tolerance (OGTT) test (the data in the chart is reported as mean ± SEM).
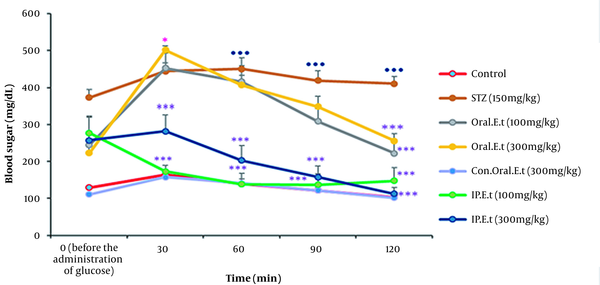
4.6. Investigation of Insulin Levels in Rat Serum
No significant change was found in serum insulin levels of the control group treated with 300 mg/kg extract orally in comparison with those of the control group. In addition, the level of serum insulin was significantly decreased in the diabetic control group in comparison with that of the control group (P < 0.001). There was a remarkable increase in serum insulin levels of diabetic groups treated with 100 mg/kg extract in doses of 100 mg/kg by gavage and 300 mg/kg extract by IP injection (P < 0.001) (Figure 6).
Serum insulin levels among groups (the data in the chart is reported as mean ± SEM; Con, control; IP, intraperitoneal; E.t, Eryngium thyrsoideum; STZ, streptozotocin).
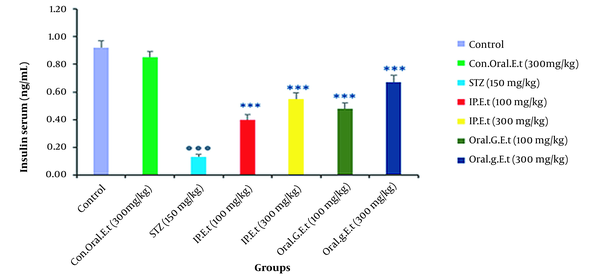
4.7. Investigation of MDA in the Serum of Mice
No significant change was observed in serum MDA of the control group treated with 300 mg/kg extract by gavage compared to that of the control group (Figure 7). Furthermore, there was a significant increase in the diabetic control group in comparison with the control group in this regard (P < 0.001). Serum MDA was remarkably reduced in diabetic groups treated with 100 mg/kg by gavage and 300 mg/kg extract by IP injection (P < 0.001).
Serum MDA levels among groups (the data in the chart is reported as mean ± SEM; Con, control; IP, intraperitoneal; E.t, Eryngium thyrsoideum; STZ, streptozotocin).
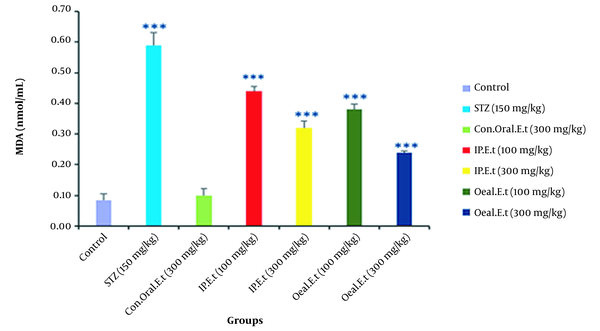
4.8. Investigation of TAC in the Serum of Mice
The amount of serum TAC in the diabetic control group was significantly higher than that in the healthy control group (P < 0.05), which was increased due to the large oxidative stress induced by STZ. Although the elevation of TAC occurred in both extract-receiving groups in comparison to the healthy control group, there was no significant difference between the given groups and the diabetic control group (Figure 8).
Serum total antioxidant capacity (TAC) levels among groups (the data in the chart is reported as mean ± SEM; Con, control; STZ, streptozotocin).
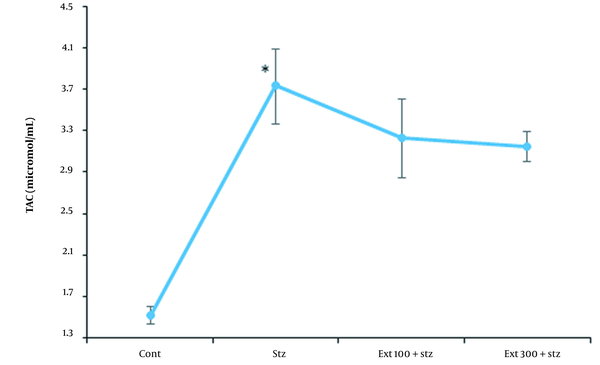
5. Discussion
This study mainly aimed to investigate the effects of the extracts from two species of Eryngium on blood glucose, serum insulin, and serum MDA in diabetic male mice. STZ was used as a single dose to induce diabetes. After the injection of STZ, the pancreatic beta cells alkylate DNA by glucose transporter (GLUT2). DNA damage activates poly ADP-ribosylation that leads to a decreased cellular NAD+ and ATP. Eventually, STZ destroyes pancreatic beta cells (32). The results of studies on species of the Eryngium genus have indicated that the extracts from different species of this plant have anti-different diabetic effects. The results of Jaghabir's study have shown that oral consumption of E. creticum plant significantly reduces blood glucose concentration (33). A study also found that glycosidic flavonols obtained from the ethyl acetate fraction of the dried aerial extract of E. caeruleum had anti-diabetic effects (34). Our findings demonstrated that between two species of Eryngium, only the hydroalcoholic extract of E. thyrsoideum had the potential to decrease blood sugar and increase serum insulin in diabetic groups receiving the extract by gavage (oral) and IP injection in low and high doses in the last ten days of the test (P < 0.001). In our study, however, no significant change was detected in blood sugar or serum insulin through the hydroalcoholic extract of E. caucasicum. In 2019, a study investigating the anti-diabetic effects of hydroalcoholic extract from E. caucasicum on diabetic rats with STZ for 30 days found that this plant was not remarkably effective in reducing blood sugar at 100 mg/kg extract (35). Seemingly, different species of the plant have various medical features based on their phylogenetic, growth condition, different chemical constituents, or even the soil compounds on which they grow. Our results from the statistical data analysis, as well as the findings from previous studies on STZ, showed that STZ caused weight loss in mice (36). Receiving E. thyrsoideum hydroalcoholic extract by gavage and IP injection in 100 and 300 mg/kg doses was found to reduce the weight of diabetic rats. To evaluate the effect of the hydroalcoholic extract of E. thyrsoideum on glucose homeostasis in mice, Oral Glucose Tolerance (OGTT) was used as an indicator to investigate the islets of Langerhans' function. When insulin secretion is insufficient, blood glucose levels can increase extremely within two hours (after oral use of glucose), which indicates glucose tolerance (37). The OGTT results of our study indicated that the diabetic control group tolerated the high level of glucose. However, no significant increase was observed in blood sugar levels of the diabetic groups receiving hydroalcoholic extract at doses (100 mg/kg) and (300 mg/kg) by IP injection. No increase was detected in their blood sugar level 30 min after receiving oral glucose, but the decrease in blood sugar level was remarkable since the initiation of the text (***P < 0.001). Moreover, the level of blood sugar was increased in diabetic groups treated with hydroalcoholic extract of the plant 30 min after receiving oral glucose at doses of 100 mg/kg and 300 mg/kg, but this level was gradually decreased so that a considerable decrease was observed in blood sugar levels of these groups after 120 min (***P < 0.001). Increased levels of oxidative stress act as a risk factor leading to insulin resistance and metabolic syndrome in type 2 diabetes (38). Most Eryngium species contain carotene and other antioxidant compounds such as tannins, sucrose, saponins, and alkaloids (39). In addition, acetylene, flavonoids, coumarin, triterpenes, sesquiterpenes, and monoterpenes (40, 41) are considered different compounds with antioxidant capability. New sources of natural antioxidants, such as the methanolic extract of Eryngium leaves have been recognized, which has a good antioxidant effect on H2O2 in the flowering stage, on NO in the non-flowering stage, and on lipids through inhibition of their peroxidation. Methanolic extract of E. billardieri dramatically inhibits lipid peroxidation (42). To evaluate the protective effect of E. thyrsoideum extract in diabetes induced by STZ, the coincidental management of STZ (IP) and 300 mg/kg extract was performed on mice. Our study results revealed that diabetes developed on the 3rd day; this implied that the gavage at 300 mg/kg extract had failed to completely protect the pancreatic beta cells from STZ damage and, therefore, mice became diabetic on the 3rd day. Considering the therapeutic mechanism of antidiabetic drugs aiming at lowering the blood sugar levels and increasing serum insulin levels, our study results may have introduced two possible mechanisms regarding the function of the hydroalcoholic extract of E. thyrsoideum. By way of explanation, the plant extract may have been able to prevent further damage to pancreatic beta cells by STZ, or the extract of this plant may have had the potential to stimulate the secretion of insulin from the remaining healthy beta cells of the pancreas. As anti-diabetic chemical drugs have various side effects, finding novel anti-diabetic natural products has been strongly suggested. Among the studied species of Eryngium, only the anti-diabetic effect of E. thyrsoideum is recognized since it increases the serum insulin and diminishes the serum MDA levels. In the current study, therefore, the formulation of E. thyrsoideum extract as a natural anti-diabetic drug was investigated. Furthermore, using natural drugs may help to reduce the dosage of chemical anti-diabetic drugs as well as their side effects. Therefore, it was recommended that further clinical human studies should be conducted in this regard. However, the application of this plant has some limitations which should be identified and overcome by conducting more studies aiming at identifying its chemical and pharmacological properties, especially its effective compounds. It was also suggested that robust and viable models should be developed to assess the herb-insulin interactions before the extension of clinical studies. The existing clinical evidence supports the application of lower level of herbal medicine in the treatment of diabetic; however, scant research attention has given to the mechanisms of this plant activity and its mechanism of action. Although the herb-drug interactions may often produce therapeutic benefits via enhancing drug effects or reducing adverse reactions, the potential risk factors associated with these interactions and their concurrent application with pharmaceutical medicines have not been ever investigated in diabetic patients. In this study, therefore, it was suggested that further studies should be carried out to investigate: (1) the hydroalcoholic extract of the plant in terms of phytochemistry in order for identifying its effective chemical and anti-diabetic compounds; (2) the antidiabetic effect of the extract from this plant in other solvents; (3) the antidiabetic effect of the essential oil from this plant on a clinical level; and (4) the anti-diabetic functional mechanism of the extract from this plant as well as the effects of the studied plant extract comparatively and simultaneously with several classes of anti-diabetic drugs in order for recognizing the similarities of the functional mechanisms of this plant’s extract.
5.1. Conclusions
Although two species of Eryngium were assessed for their anti-diabetic effect, only E. thyrsoideum extract was found to increase serum insulin and diminish serum MDA. It was determined that the hydroalcoholic extract of E. thyrsoideum significantly reduced MDA levels in both IP injection and gavage (oral) injection modes (***P < 0.001); this suggested that the hydroalcoholic extract of the plant was able to improve antioxidant activity in the body and reduce the damage to body organs caused by oxidative stress. According to our study results, therefore, it was concluded that the hydroalcoholic extract of E. thyrsoideum had the potential to protect pancreatic beta cells from STZ damage by stimulating the remaining healthy pancreatic beta cells to secret insulin, and by increasing the serum insulin levels alone, which contributed to the reduction of blood sugar and serum MDA.
References
-
1.
Jarald E, Joshi SB, Jain D. Diabetes and herbal medicines. Iran J Pharmacol Ther. 2008;7(1):97-106.
-
2.
Altan VM. The pharmacology of diabetic complications. Curr Med Chem. 2003;10(15):1317-27. [PubMed ID: 12871132]. https://doi.org/10.2174/0929867033457287.
-
3.
Sarwar N, Gao P, Seshasai SR, Gobin R, Kaptoge S, Di Angelantonio E, et al. Diabetes mellitus, fasting blood glucose concentration, and risk of vascular disease: a collaborative meta-analysis of 102 prospective studies. Lancet. 2010;375(9733):2215-22. [PubMed ID: 20609967]. [PubMed Central ID: PMCPmc2904878]. https://doi.org/10.1016/s0140-6736(10)60484-9.
-
4.
Saeedi P, Petersohn I, Salpea P, Malanda B, Karuranga S, Unwin N, et al. Global and regional diabetes prevalence estimates for 2019 and projections for 2030 and 2045: Results from the International Diabetes Federation Diabetes Atlas, 9(th) edition. Diabetes Res Clin Pract. 2019;157:107843. [PubMed ID: 31518657]. https://doi.org/10.1016/j.diabres.2019.107843.
-
5.
Morrow ES, Allscripts E. Natural medicines in the clinical management of diabetes. San Frncisco, USA: Natural Medicines; 2018, [cited 2022]. Available from: https://naturalmedicines.therapeuticresearch.com.
-
6.
Khansa L, Davis Z, Davis H, Chin A, Irvine H, Nichols L, et al. Health information technologies for patients with diabetes. Technol Soc. 2016;44:1-9. https://doi.org/10.1016/j.techsoc.2015.11.001.
-
7.
Mozaffarian V. Studies on the flora of Iran, new species and new records. The Iranian Journal of Botany. 1994;6(2):235-44.
-
8.
Zheng XK, Zhang L, Wang WW, Wu YY, Zhang QB, Feng WS. Anti-diabetic activity and potential mechanism of total flavonoids of Selaginella tamariscina (Beauv.) Spring in rats induced by high fat diet and low dose STZ. J Ethnopharmacol. 2011;137(1):662-8. [PubMed ID: 21718776]. https://doi.org/10.1016/j.jep.2011.06.018.
-
9.
Ramesh B, Pugalendi KV. Antihyperglycemic effect of umbelliferone in streptozotocin-diabetic rats. J Med Food. 2006;9(4):562-6. [PubMed ID: 17201645]. https://doi.org/10.1089/jmf.2006.9.562.
-
10.
Yang CY, Wang J, Zhao Y, Shen L, Jiang X, Xie ZG, et al. Anti-diabetic effects of Panax notoginseng saponins and its major anti-hyperglycemic components. J Ethnopharmacol. 2010;130(2):231-6. [PubMed ID: 20435129]. https://doi.org/10.1016/j.jep.2010.04.039.
-
11.
Velayutham R, Sankaradoss N, Ahamed KF. Protective effect of tannins from Ficus racemosa in hypercholesterolemia and diabetes induced vascular tissue damage in rats. Asian Pac J Trop Med. 2012;5(5):367-73. [PubMed ID: 22546653]. https://doi.org/10.1016/s1995-7645(12)60061-3.
-
12.
Jung UJ, Lee MK, Park YB, Jeon SM, Choi MS. Antihyperglycemic and antioxidant properties of caffeic acid in db/db mice. J Pharmacol Exp Ther. 2006;318(2):476-83. [PubMed ID: 16644902]. https://doi.org/10.1124/jpet.106.105163.
-
13.
Hunyadi A, Martins A, Hsieh TJ, Seres A, Zupkó I. Chlorogenic acid and rutin play a major role in the in vivo anti-diabetic activity of Morus alba leaf extract on type II diabetic rats. PLoS One. 2012;7(11). e50619. [PubMed ID: 23185641]. [PubMed Central ID: PMCPmc3503931]. https://doi.org/10.1371/journal.pone.0050619.
-
14.
Karimi S, Farzaneh F, Asnaashari S, Parina P, Sarvari Y, Hazrati S. Phytochemical Analysis and Anti-microbial Activity of Some Important Medicinal Plants from North-west of Iran. Iran J Pharm Res. 2019;18(4):1871-83. [PubMed ID: 32184854]. [PubMed Central ID: PMCPmc7059075]. https://doi.org/10.22037/ijpr.2019.1100817.
-
15.
García MD, Sáenz MT, Gómez MA, Fernández MA. Topical antiinflammatory activity of phytosterols isolated from Eryngium foetidum on chronic and acute inflammation models. Phytother Res. 1999;13(1):78-80. [PubMed ID: 10189959]. https://doi.org/10.1002/(sici)1099-1573(199902)13:1<78::aid-ptr384>3.0.co;2-f.
-
16.
Zarei A, Ashtiyani SC, Hamidizadeh S, Rezaei A, Ramezani M, Aalizadeh A. The study of the effects hydro-alcoholic extract of Eryngium billardieri on lipid profiles levels and liver and renal functions tests in hypercholesterolemic rats. J Chem Pharm Res. 2015;7(2):200-6.
-
17.
Jaafari A, Baradaran Rahimi V, Vahdati-Mashhadian N, Yahyazadeh R, Ebrahimzadeh-Bideskan A, Hasanpour M, et al. Evaluation of the Therapeutic Effects of the Hydroethanolic Extract of Portulaca oleracea on Surgical-Induced Peritoneal Adhesion. Mediators Inflamm. 2021;2021:8437753. [PubMed ID: 34381307]. [PubMed Central ID: PMC8352699]. https://doi.org/10.1155/2021/8437753.
-
18.
Rakhshandeh H, Rajabi Khasevan H, Saviano A, Mahdinezhad MR, Baradaran Rahimi V, Ehtiati S, et al. Protective Effect of Portulaca oleracea on Streptozotocin-Induced Type I Diabetes-Associated Reproductive System Dysfunction and Inflammation. Molecules. 2022;27(18). [PubMed ID: 36144807]. [PubMed Central ID: PMC9506021]. https://doi.org/10.3390/molecules27186075.
-
19.
Khani S, Abdollahi M, Asadi Z, Nazeri M, Nasiri MA, Yusefi H, et al. Hypoglycemic, hepatoprotective, and hypolipidemic effects of hydroalcoholic extract of Eryngium billardieri root on nicotinamide/streptozotocin-induced type II diabetic rats. Res Pharm Sci. 2021;16(2):193-202. [PubMed ID: 34084206]. [PubMed Central ID: PMCPmc8102925]. https://doi.org/10.4103/1735-5362.310526.
-
20.
Etuk EU. Animals models for studying diabetes mellitus. Agric Biol JN Am. 2010;1(2):130-4.
-
21.
Tsutsumi T, Kobayashi S, Liu YY, Kontani H. Anti-hyperglycemic effect of fangchinoline isolated from Stephania tetrandra Radix in streptozotocin-diabetic mice. Biol Pharm Bull. 2003;26(3):313-7. [PubMed ID: 12612439]. https://doi.org/10.1248/bpb.26.313.
-
22.
Pournaghi P, Sadrkhanlou RA, Hasanzadeh S, Foroughi A. An investigation on body weights, blood glucose levels and pituitary-gonadal axis hormones in diabetic and metformin-treated diabetic female rats. Vet Res Forum. 2012;3(2):79-84. [PubMed ID: 25653751]. [PubMed Central ID: PMCPmc4312800].
-
23.
Chaves AA, Weinstein DM, Bauer JA. Non-invasive echocardiographic studies in mice: influence of anesthetic regimen. Life Sci. 2001;69(2):213-22. [PubMed ID: 11441911]. https://doi.org/10.1016/s0024-3205(01)01123-7.
-
24.
Fish R, Danneman PJ, Brown M, Karas A. Anesthesia and analgesia in laboratory animals. Massachusetts, USA: Academic press; 2011.
-
25.
Xu Q, Ming Z, Dart AM, Du XJ. Optimizing dosage of ketamine and xylazine in murine echocardiography. Clin Exp Pharmacol Physiol. 2007;34(5-6):499-507. [PubMed ID: 17439422]. https://doi.org/10.1111/j.1440-1681.2007.04601.x.
-
26.
Boivin GP, Hickman DL, Creamer-Hente MA, Pritchett-Corning KR, Bratcher NA. Review of CO₂ as a Euthanasia Agent for Laboratory Rats and Mice. J Am Assoc Lab Anim Sci. 2017;56(5):491-9. [PubMed ID: 28903819]. [PubMed Central ID: PMCPmc5605172].
-
27.
Hoff J. Methods of blood collection in the mouse. Lab Animal. 2000;29(10):47-53.
-
28.
Chen Z, Wang C, Pan Y, Gao X, Chen H. Hypoglycemic and hypolipidemic effects of anthocyanins extract from black soybean seed coat in high fat diet and streptozotocin-induced diabetic mice. Food Funct. 2018;9(1):426-39. [PubMed ID: 29220052]. https://doi.org/10.1039/c7fo00983f.
-
29.
Han X, Tao YL, Deng YP, Yu JW, Cai J, Ren GF, et al. Metformin ameliorates insulitis in STZ-induced diabetic mice. PeerJ. 2017;5. e3155. [PubMed ID: 28439456]. [PubMed Central ID: PMCPmc5399881]. https://doi.org/10.7717/peerj.3155.
-
30.
Hu J, Zhang YX, Wang L, Ding L, Huang GY, Cai GW, et al. Protective effects of Xinji'erkang on myocardial infarction induced cardiac injury in mice. BMC Complement Altern Med. 2017;17(1):338. [PubMed ID: 28651598]. [PubMed Central ID: PMCPmc5485507]. https://doi.org/10.1186/s12906-017-1846-5.
-
31.
Benzie IF, Strain JJ. The ferric reducing ability of plasma (FRAP) as a measure of "antioxidant power": the FRAP assay. Anal Biochem. 1996;239(1):70-6. [PubMed ID: 8660627]. https://doi.org/10.1006/abio.1996.0292.
-
32.
Szkudelski T. The mechanism of alloxan and streptozotocin action in B cells of the rat pancreas. Physiol Res. 2001;50(6):537-46. [PubMed ID: 11829314].
-
33.
Jaghabir M. Hypoglycemic effects ofEryngium creticum. Arch Pharm Res. 1991;14(4):295-7. https://doi.org/10.1007/bf02876873.
-
34.
Rehman AU, Hashmi MA, Tehseen Y, Khan A, Khan SS, Iqbal J, et al. Antidiabetic flavonol glycosides from Eryngium caeruleum. Rec Nat Prod. 2017;11(2):229-34.
-
35.
Afshari M, Mohammadshahi M, Malayeri AR, Zaheri L. Antidiabetic, hepato-protective and hypolipidemic effects of Eryngium caucasicum extract in streptozotocin-nicotinamide induced type 2 diabetes in male rats. Iraq Med J. 2019;3(1):11-6.
-
36.
Babby A, Elanchezhiyan C, Suhasini S, Chandirasegaran G. Antihyperglycemic effect of tannic acid in streptozotocin induced diabetic rats. Int J Curr Res. 2014;6(3):5396-8.
-
37.
Liu W, Zheng Y, Zhang Z, Yao W, Gao X. Hypoglycemic, hypolipidemic and antioxidant effects of Sarcandra glabra polysaccharide in type 2 diabetic mice. Food Funct. 2014;5(11):2850-60. [PubMed ID: 25254968]. https://doi.org/10.1039/c4fo00430b.
-
38.
Meigs JB, Larson MG, Fox CS, Keaney JJ, Vasan RS, Benjamin EJ. Association of oxidative stress, insulin resistance, and diabetes risk phenotypes: the Framingham Offspring Study. Diabetes Care. 2007;30(10):2529-35. [PubMed ID: 17586736]. https://doi.org/10.2337/dc07-0817.
-
39.
Semnani KM, Azadbakht M, Houshmand A. [Composition of the essential oils of aerial parts of Eryngium bungei Boiss. and Eryngium caeruleum MB]. Pharm Sci. 2003;(1):43-8. Persian.
-
40.
Paul JH, Seaforth CE, Tikasingh T. Eryngium foetidum L.: a review. Fitoterapia. 2011;82(3):302-8. [PubMed ID: 21062639]. https://doi.org/10.1016/j.fitote.2010.11.010.
-
41.
Promkum C, Butryee C, Tuntipopipat S, Kupradinun P. Anticlastogenic effect of Eryngium foetidum L. assessed by erythrocyte micronucleus assay. Asian Pac J Cancer Prev. 2012;13(7):3343-7. [PubMed ID: 22994758]. https://doi.org/10.7314/apjcp.2012.13.7.3343.
-
42.
Wang P. Phytochemical Constituents and Pharmacological Activities of Eryngium L. (Apiaceae). Pharm Crop. 2012;3(1):99-120. https://doi.org/10.2174/2210290601203010099.