Abstract
Background:
The Conocarpus extract possesses antioxidant, antibacterial, and anti-inflammatory properties, which may prove to be beneficial in the healing of skin wounds.Objectives:
This study investigated the protective effects of Conocarpus leaf extract on HSF-PI 17 fibroblast cells against the harmful effects of UVB radiation.Methods:
After culturing the cells, the MTT assay was employed to evaluate the cytotoxicity of the Conocarpus extract. The cells were divided into three groups: The control group, the group receiving radiation only, and the group receiving radiation along with the Conocarpus leaf extract. Trypan blue staining was utilized to quantify the count of viable cells. The level of reactive oxygen species (ROS) production was determined by measuring the intensity of fluorescence color. Real-time PCR was utilized to evaluate gene expression, while Western blotting was employed to determine protein expression.Results:
Conocarpus extract had no toxic effects on HSF-PI 17 cells at doses ranging from 0.001 to 1 g/mL. Exposure to UVB radiation led to a notable rise in the production of ROS and a considerable decline in cell growth rate compared to the control group (P < 0.05). In the third group, the Conocarpus extract significantly moderated the reduction in growth and production of ROS compared to the second group (P < 0.05). TGF-β and SMAD2/3 gene expressions, as well as collagen protein levels, were significantly lower in the second group than in the control group (P < 0.05). TGF-β and SMAD2/3 gene expressions, as well as collagen protein expression, showed a significant increase in the third group compared to the second group (P < 0.05).Conclusions:
Conocarpus leaf extract reduces the harmful effects of UVB radiation on HSF-PI 17 skin fibroblast cells.Keywords
1. Background
The skin is the largest organ and the first protective layer of the body. Nowadays, with the growing emphasis on health and beauty worldwide, there has been a rise in oxidizing and harmful chemical factors such as sunlight and active oxygen species. As a result, many researchers are exploring methods to prevent skin damage and aid in skin repair (1). Many of the chemical or herbal compounds used in this field have unfavorable side effects or are ineffective at preventing aging and skin inflammation (2).
Conocarpus erectus (C. erectus), an evergreen tree, is an ornamental mangrove (3). The pharmaceutical industry greatly benefits from the abundance of biologically active substances found in mangrove trees (4). Conocarpus erectus, a plant with spherical flowers, is common in tropical and subtropical areas of the world (5). The cultivation of C. erectus in Iran’s south and southwest has received widespread approval due to its superior pruning capabilities and high survival rate (6). Studies on C. erectus use in medicine have demonstrated its anti-inflammatory, antimicrobial, and anticancer properties. This plant has also been used to treat conjunctivitis, cataracts, diabetes, heatstroke, tissue swelling, and fever (7). Although C. erectus extract has many beneficial qualities, it has not received the attention it deserves, and most people are unaware of its therapeutic capabilities (7). Given the antibacterial and anti-inflammatory properties of the C. erectus leaf extract, its effectiveness in healing skin wounds, ease of cultivation, and low cost of upkeep, it can potentially be a desirable treatment for preventing skin aging (8-10) if its effects on skin protection are proven. Conocarpus erectus is a resilient plant that can store water to endure hot climates (11). This plant is widely cultivated in the southern regions of Iran, including the city of Ahvaz (12). The effects of C. erectus leaf extract on preventing aging and rejuvenating skin cells have not been studied.
The current study investigated the protective effect of the leaf extract of C. erectus against ultraviolet B (UVB) radiation in skin fibroblast cells. This study applied C. erectus plant extract to human skin fibroblast cells (HSF-PI 17) exposed to UVB radiation.
2. Objectives
The study aimed to investigate the impact of C. erectus leaf extract on the cell growth rate, viability, and collagen production by activating the transforming growth factor-β/suppressor of mothers against decapentaplegic 2/3 (TGF-β/SMAD2/3) pathway, as well as its effect on the rate of free radical production.
3. Methods
3.1. Preparation of Plant Extract
The leaves of C. erectus were gathered from Ahvaz Jundishapur University of Medical Sciences’ medicinal plant garden. The plant was identified by Khuzestan Agricultural and Natural Resources Research and Education Center herbarium. The voucher number is 10925. The leaves were separated from the branches and dried entirely in an oven at 50°C for 24 hours. After thoroughly grinding the leaves using a mortar, 200 g of the resulting powder was dissolved in 1000 mL of 80% methanol solution. The plant extract was harvested for six hours using a Soxhlet apparatus (Farazma Research, Iran) (13).
3.2. Cell Culture
The cellular experiment was approved by the Animal Experiments Committee of the Ahvaz Jundishapur University of Medical Sciences (IR.AJUMS.REC.1401.474). We purchased HSF-PI 17 human skin fibroblast cells (NCBI Code: C193) in passage number 3 from Pasteur Cell Bank in Tehran. Cells were cultured in flasks containing Dulbecco’s Modified Eagle Medium-High Glucose (DMEM-HG) (Sigma brand, USA), 10% Fetal Bovine Serum (FBS) (Sigma brand, USA), and 1% penicillin/streptomycin (Pen/Strep) (Sigma brand, USA) in an incubator containing 5% CO2 at 37°C. The culture medium of the cells was changed every two or three days. The cells were passaged when the cell density reached about 90% (14, 15).
3.3. MTT Assay
Cells were cultured at a density of 104 cells per well in a 96-well culture plate in triplicate. After 24 h of incubation at 37°C, C. erectus extract concentrations of 0.001, 0.01, 0.1, and 1 g/mL in phosphate buffer saline (PBS) (Sigma brand, USA) were applied to the cells. Three wells were considered as control. After 24 h of incubation at 37°C, the cell supernatant was removed, and 100 μL of 0.5 mg/mL 3-(4,5-Dimethylthiazol-2-yl)-2,5-Diphenyltetrazolium Bromide (MTT) reagent (Sigma, USA) was added to each well. After 4 h of incubation in the dark at 37°C, 100 μL of dimethyl sulfoxide (DMSO) reagent (Sigma, USA) was added to each well, and the plate was placed in a shaker for 15 min. Then, the optical spectrum of the samples was read by ELISA reader (Bio-RAD, USA) at a wavelength of 570 nm. The cell survival percentage of each group was calculated with the formula (16):
3.4. Ultraviolet B Radiation Therapy and/or Conocarpus erectus Extract Application
The cells were divided into three groups: The control group, the group receiving UVB radiation with an intensity of 144 mJ/cm2 (17), and the group receiving 0.016 g/mL of C. erectus extract in addition to UVB radiation (18).
3.5. Proliferation Assay
The cells of the three groups were cultured in triplicate with a density of 105 cells per well in a 12-well culture plate. After 24 h of incubation at 37°C, the cells of groups 2 and 3 were exposed to UVB radiation with an intensity of 144 mJ/cm2. The 0.016 g/mL extract of C. erectus was added to the third group. After 24 h of incubation, the cells were detached from the bottom of the flask using a 0.25% Trypsin/Ethylenediaminetetraacetic acid (EDTA) solution (Sigma, USA) and stained with trypan blue. Then, the number of viable cells in each well was determined using an inverted microscope (Olympus, Germany). The average number of viable cells in each group was determined. The cells were cultured again in the 12-well plate. The number of viable cells was counted again 24 h later. The process was done for ten consecutive days, and the average was used to draw the cell growth curve.
3.6. Estimating Reactive Oxygen Species Production
The Oxi SelectTM assay kit measured the intracellular reactive oxygen species (ROS) production level. Their production was determined based on the production of green fluorescent dye dichlorodihydro fluorescein (DCF). The cells were cultured in triplicate in 96-well culture plates. After 24 h of incubation at 37°C, the supernatant was discarded, and 100 µL of DA-DCFH 1X was added to each well and incubated at 37°C for 30 min. Then, the supernatant was removed, and three washing processes were applied. After administration of radiation and/or C. erectus extract, 100 µL of culture medium and 100 µL of lysing buffer were added to each well. The plates were then incubated for another 5 min at 37°C. The amount of fluorescence was measured at 480 and 530 nm wavelengths using a Dako flow cytometer (Troy, USA). Data were analyzed using Flow Jo software.
3.7. Real-time Polymerase Chain Reaction Method
The cells were cultured in triplicate on six culture plates for real-time PCR analysis. After irradiation or addition of C. erectus extract, the cell precipitate was isolated by treating with Trypsin/EDTA and incubating at 37°C for 24 hours. First, RNA was extracted. Then, cDNA synthesis was performed, followed by gene amplification using real-time PCR.
3.8. RNA Extraction
The RNA was extracted using a Sinaclon kit (Iran) by column chromatography following the kit’s instructions. The quality of the extracted RNA was assessed by measuring the OD260/OD280 ratio in a Nanodrop device (Thermo, Canada). The OD260/OD280 ratios between 1.8 and 2 indicated acceptable quality for RNA.
3.9. cDNA Synthesis
The CycleScript RT PreMix cDNA synthesis kit (Bioneer, South Korea) was used to conduct the cDNA synthesis reaction. An oligo dT primer was applied to construct cDNA. According to the kit’s instructions, the reactions were performed in 20 µL.
3.10. Real-time Polymerase Chain Reaction
The primers for TGF-β and Smad2/3 were designed using the Primer3 online software. The designed primers were as follows. The human TGF-β1 forward and reverse primers were 5’-CCCAGCATCTGCAAAGCTC-3’ and 5’-GTCAATGTACAGCTGCCGCA-3’. The forward primer for Smad2 was 5’-CCGGAATTCATGTCGTCCATCTTGCCATTCAC-3’, and the reverse primer was 5’-TCCGCTCGAGTTATGACATGCTTGAGCA ACGCAC-3’. The forward primer for Smad3 was 5’-CGCGGATCCATGTCGTCCATCCTGCCTTTC-3’, and the reverse primer was 5’-TCCGCTCGAGTCAAGACACACTGGAAC AGCGGA-3’. The GAPDH forward and reverse primers were 5’-TGTTGCCATCAATGACCCCTT-3’ and CTCCACGACGTACTCAGCG, respectively (19, 20). Each primer was used at 0.5 μM concentration. The Ampliqon RealQ Plus Master kit for SYBR Green I, made in Denmark, was used to carry out the PCR in a total volume of 12.5 µL according to the kit’s instructions. The American-made Roche Lightcycler Detection System managed to run the PCR through 45 temperature cycles. Two negative control reactions, one without RNA and the other without cDNA, were used to confirm the reaction’s accuracy. The expression levels of various genes were compared based on the 2-ΔΔCt method. The reactions were performed in triplicate. The GAPDH gene was used as a reference gene.
3.11. Western Blotting
The cells were detached from the flask bottom for Western blotting to assess the collagen expression level. The cell pellet was dissolved in PBS and centrifuged at 448 g for 5 min. Afterward, the PBS supernatant was discarded, and the cell pellet was resuspended in 100 µL of radioimmunoprecipitation assay buffer (RIPA) lysis buffer (Sigma, USA). Following a 30-min ice-incubation period, the samples were centrifuged at 11,200 g for 20 min. The supernatant was collected, and the total protein concentration was calculated using the Bradford method. Then, 10 µL of the cell supernatant and 10 µL of the mercapto ethanol-containing buffer were combined. The resulting mixture was incubated at 70°C for 10 min. The final samples were loaded onto a 12% sodium dodecyl sulfate (SDS) PAGE gel and a nitrocellulose membrane. Membranes were blocked by 5% dry milk in PBS. After washing with PBS/Tween, GAPDH and primary collagen antibodies (ab 8245, ab34710) (Abcam, USA) were diluted at a 1/1000 ratio and added to membranes. Then, secondary antibodies conjugated with HRP (P0048 Dako) (Dako, Denmark) were diluted at a 1/1000 ratio and added. The membranes were incubated with diaminobenzidine (DAB) for 10 min at room temperature and dried in a 37°C incubator for 15 min. The densitometry of collagen was determined compared to reference protein GAPDH using Image J software. All tests were done in triplicate.
3.12. Statistical Analysis
Data were analyzed using SPSS software (SPSS Inc., Chicago, IL, USA). All experiments were done in triplicate. The mean values were compared using the one-way Analysis of Variance (ANOVA) and Tukey post-hoc statistical tests.
4. Results
4.1. Determination of Conocarpus erectus Extract Toxicity
The MTT results revealed that C. erectus leaf extract at concentrations of 0, 0.001, 0.01, 0.1, and 1 g/mL had no toxic effect on HSF-PI 17 fibroblast cells (P > 0.05) (Figure 1).
Cell viability changes after administration of various Conocarpus erectus extract concentrations. The MTT assay results showed that the administration of C. erectus extracts at 0, 0.001, 0.01, 0.1, and 1 g/mL concentrations had no effect on cell viability (P > 0.05). All experiments were carried out in triplicate.
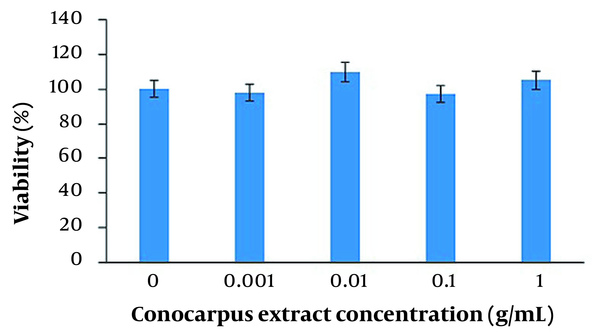
4.2. Cell Proliferation
Cell counting revealed that UVB radiation causes a significant decrease in the number of living cells compared to the control group (P < 0.05) (Figures 2 and 3). The group that received UVB and C. erectus extract simultaneously showed a significantly elevated number of viable cells compared to the group that received radiation only (P < 0.05) (Figures 2 and 3).
Changes in the morphology of HSF-PI17 cells after treatment with 144 mJ/cm2 Ultraviolet B (UVB) and 0.016 g/cm2Conocarpus erectus leaf extract. Untreated cells contained many viable fibroblasts with spindle-like morphology (A). The cells that received 144 mJ/cm2 UVB had fewer viable cells and shorter cell morphology (B). The cells were treated with 0.016 g/mL of C. erectus leaf extract, reducing UVB administration’s lethal effect (C). The scale bar was set to 100X.

HSF-PI17 cell proliferation assay after exposure to 144 mJ/cm2 Ultraviolet B (UVB) and/or 0.016 g/mL Conocarpus erectus leaf extract. The 144 mJ/cm2 UVB group cells had a significantly lower proliferation rate than control fibroblast cells. Treatment of the cells with 0.016 g/mL C. erectus leaf resulted in a significant increase in proliferation rate compared with group 2.
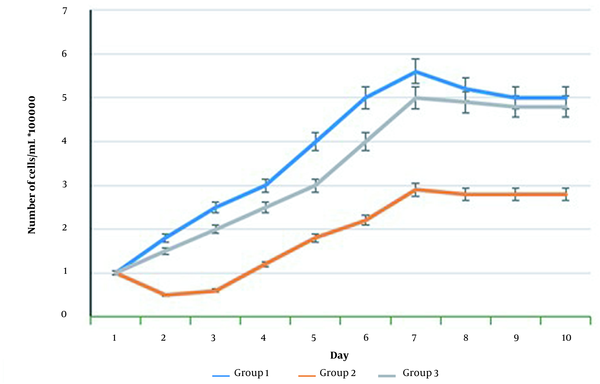
4.3. Reactive Oxygen Species Production
In the second group, UVB significantly increased the production of the oxidants in cells compared to the control group (P < 0.05) (Figure 4). While in the third group, the addition of C. erectus extracts caused a substantial reduction in ROS production compared to the second group (P < 0.05) (Figure 4).
Reactive oxygen species production in HSF-PI17 cells after exposure to 144 mJ/cm2 UVB and/or 0.016 g/mL Conocarpus erectus leaf extract. Ultraviolet B (UVB) administration significantly increased ROS production compared to the control group (P < 0.05). However, the group that received 0.016 g/mL C. erectus leaf extract showed a significant reduction in ROS production compared to group 2 (P < 0.05). All experiments were carried out in triplicate. The data are presented as mean and standard deviation. Bars with different letters differ significantly.
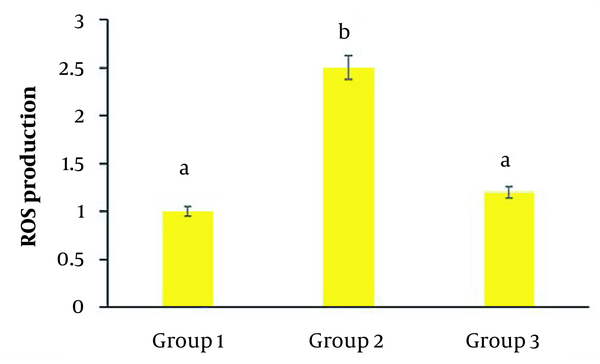
4.4. TGF-β and Smad2/3 Expression
The group that received UVB showed significantly decreased levels of TGF-β and Smad2/3 genes compared to the control group (P < 0.05) (Figure 5A and B ). The third group, which received radiation and C. erectus extract at the same time, revealed a substantial elevation in TGF-β and Smad2/3 expression compared to group 2 (P < 0.05) (Figure 5A and B ).
TGF- and Smad2/3 expression in HSF-PI17 cells after 144 mJ/cm2 Ultraviolet B (UVB) and/or 0.016 g/mL C. erectus leaf extract exposure. TGF- and Smad 2/3 expression in HSF-PI 17 cells was significantly reduced in the group that received 144 mJ/cm2 UVB (P < 0.05). TGF- and Smad 2/3 expressions were significantly modulated by 0.016 g/mL C. erectus leaf extract (P < 0.05) (A, B). All experiments were carried out in triplicate. The data are presented as mean and standard deviation. The differences between bars with different letters are significant.
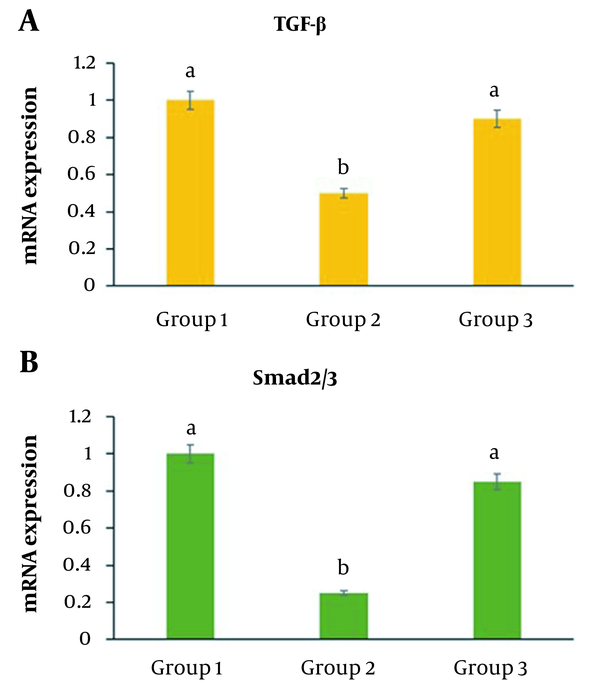
4.5. Collagen Expression
The results of Western blotting showed that in group 2, which received radiation, the level of collagen protein expression was significantly lower than in the control group (P < 0.05) (Figure 5 A and B ). While in the third group, the addition of C. erectus extract moderated the expression of collagen protein compared to the second group (P < 0.05) (Figure 6).
Collagen protein expression in HSF-PI17 cells after 144 mJ/cm2 Ultraviolet B (UVB) and/or 0.016 g/mL C. erectus leaf extract treatment. The ability of HSF-PI 17 cells to express collagen type 1 was significantly reduced in the group that received 144 mJ/cm2 UVB (P < 0.05). However, 0.016 g/mL of C. erectus leaf extract significantly modulated collagen type 1 expression (P < 0.05). All experiments were carried out in triplicate. The data are presented as mean and standard deviation. The differences between bars with different letters are significant.
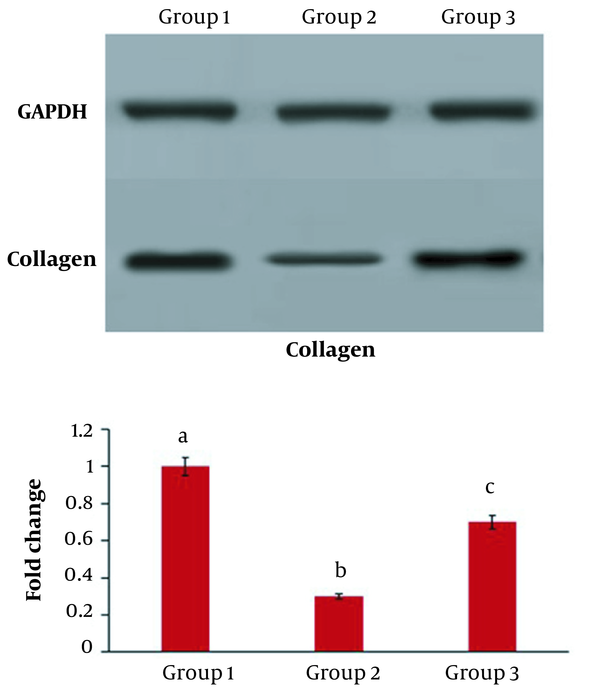
5. Discussion
The skin is the body’s largest organ that plays a wide range of protective functions against pathogen entry. Thus, efforts to protect skin health not only help prevent the entry of various bacteria and pathogens but also maintain a constant body temperature. Additionally, healthy skin supports a sense of freshness and individual self-confidence (21). Skin aging is a natural and unavoidable process characterized by a decrease in the skin’s ability to synthesize collagen, thickening of the epidermis layer, and the formation of wrinkles on the skin. Today, we are exposed to numerous oxidizing agents, such as harmful radiation, industrial pollutants, hazardous chemicals, and pathogens. Environmental oxidizing agents can cause premature destruction of skin layers and accelerate the aging process (22). Researchers around the world are working to address the issue of premature skin aging. Nowadays, various natural plant extracts are used to protect the skin. Plant extracts contain skin-restoring ingredients such as antioxidants, proteins, peptides, retinoids, and hydroxy acids, which aid in the re-synthesis of collagen and elastin and the prevention of oxidative reactions (23). Previous research has demonstrated the benefits of pomegranate and coffee extracts as antioxidant drugs in skin protection (24). Ameedi et al. demonstrated that the hydroalcoholic extract of clove and C. erectus buds have valuable antibacterial and anti-inflammatory medicinal properties both in vitro and in vivo (9). Previous research has also found that chamomile extract is anti-inflammatory in skin protection (25). The mint extract can also help firm the skin (26). Coconut and jojoba plant oils soften the skin’s texture (27).
Qves et al.’s research showed the beneficial cytotoxic and antifungal effects of methanolic extract of C. erectus in cancer cells (28). Some researchers demonstrated that C. erectus leaf extract could reduce inflammation in mouse fibroblast cells (29, 30). Da Silva et al. researched the effect of hyaluronic acid extract of C. erectus on wound healing in Wistar rats. Their study found that the hydroalcoholic extract of C. erectus leaves could considerably enhance cell proliferation and collagen storage in connective tissue (31). Yasin and Al-Azawi confirmed the beneficial role of C. erectus leaf extract in healing bacterial infections caused by burns (32). Khalil et al. investigated the antibacterial and anticancer properties of a phenolic extract of C. erectus. These studies demonstrated that C. erectus extract is antibacterial against gram-positive bacteria. Furthermore, C. erectus plant extract increased cell survival in the liver cancer cell line (8).
The current study examined the effects of C. erectus leaf extract on human skin fibroblast cells. In the first stage, different extract concentrations were applied to the cells, and their survival rate was compared to that of the control group. The MTT assay results showed that the extract had no toxic effect on fibroblast cells at concentrations ranging from 0.001 to 1 g/mL. According to the findings of Saadullah et al., the average lethal dose of C. erectus extracts for mice is around 10,000 mg/kg. However, extract concentrations greater than 5,000 mg/kg have toxic effects on the liver and kidneys of mice. Their study also demonstrated that C. erectus extract does not affect red blood cell hemolysis (33). In the current study, C. erectus extract modulated the decreased growth rate of HSFP-I 17 cells caused by UVB radiation. The findings of Alsaraf et al. confirm our findings. They discovered that even though C. erectus leaf extract significantly reduced the growth and survival of breast cancer cells, it had no toxic effect on normal fibroblast cells (34). Faraj and Shawkat also confirmed no harmful effect of C. erectus extract on normal cells. As a result, it can be concluded that the therapeutic application of C. erectus extract in low doses is safe (35). Our research also found that the extract of C. erectus significantly reduced the production of ROS by HSF-PI 17 cells. Santos et al.’s findings support the antioxidant and immune-modulating properties of the pectin-like polypeptide extracted from C. erectus (36). Abdel-Hameed et al. conducted a study to examine the impact of extracts from the leaves, stems, fruits, and flowers of the C. erectus plant on CCl4-induced liver damage in rats. The researchers concluded that an extract of C. erectus neutralizes the toxic effects of CCl4 on rat liver cells, which are caused by increased oxidizing compounds in the blood. As a result, C. erectus extract may have potent antioxidant properties (37). According to Nishimoto et al. and Bhatnagar et al., platelet lysate significantly affects skin wound healing. TGF-β protein is one of the components of this lysate. As a result, any factor that increases TGF-β production will accelerate fibroblast growth and skin wound healing (38, 39). Verrecchia et al. demonstrated that increasing TGF-β protein expression activates the SMAD2/3 pathway, ultimately leading to increasing procollagen production (40).
Research shows chronic and irreversible wounds have low TGF-β content (41). TGF-β isoforms bind to TBR-I and TBR-II cell surface receptors. TGF-binding to its receptor results in receptor phosphorylation and activating the Smad 2/3 pathway. The activation of Smads ultimately activates the message of procollagen synthesis in the cell nucleus. It causes active collagen production, significantly affecting wound healing and skin rejuvenation (42). The presence of collagen promotes fibroblast absorption into the wound bed. It promotes new collagen synthesis, enabling angiogenesis and creating fresh epithelial tissue (43). Zhao et al. discovered that in pulmonary fibrosis mouse models, a lack of Smad-3 expression inhibits the mRNA expression of the procollagen gene (44). In the current study, C. erectus extract moderated the effects of UVB on TGF-β and Smad2/3 expression. It caused a significant increase in the expression of these two genes compared to the UVB group. Furthermore, C. erectus extract significantly increased collagen protein expression compared to the radiation-treated group. These findings suggest that C. erectus extract plays an active role in repairing skin damage caused by UVB radiation.
5.1. Conclusions
According to the current study findings, the methanolic extract of C. erectus leaf has no toxic effect on the survival and growth of HSF-PI 17 fibroblast cells. Also, UVB radiation induces cell proliferation reduction through free radical production. The application of C. erectus extracts moderates the decrease in TGF-β and Smad2/3 gene expression, as well as collagen protein expression. Given the beneficial effects of the methanolic extract of C. erectus leaf in repairing UVB radiation damage in HSF-PI 17 skin fibroblast cells in the current study, it is suggested that the protective effects of this extract be investigated in other skin types. Furthermore, using the extract in animal models exposed to UVB radiation and studying its protective effects in preventing skin damage can be seen as a significant step towards advancing the medical application of this extract in clinical trials.
Acknowledgements
References
-
1.
Abolhasani R, Araghi F, Tabary M, Aryannejad A, Mashinchi B, Robati RM. The impact of air pollution on skin and related disorders: A comprehensive review. Dermatol Ther. 2021;34(2). e14840. [PubMed ID: 33527709]. https://doi.org/10.1111/dth.14840.
-
2.
Do NHN, Truong QT, Le PK, Ha AC. Recent developments in chitosan hydrogels carrying natural bioactive compounds. Carbohydr Polym. 2022;294:119726. [PubMed ID: 35868739]. https://doi.org/10.1016/j.carbpol.2022.119726.
-
3.
Abbas MF, Rafiq M, Al-Sadi AM, Alfarraj S, Alharbi SA, Arif M, et al. Molecular characterization of leaf spot caused by Alternaria alternata on buttonwood (Conocarpus erectus L.) and determination of pathogenicity by a novel disease rating scale. PLoS One. 2021;16(5). e0251471. [PubMed ID: 33984023]. [PubMed Central ID: PMC8118258]. https://doi.org/10.1371/journal.pone.0251471.
-
4.
Genilar LA, Kurniawaty E, Mokhtar RAM, Audah KA. Mangroves and their medicinal benefit: A mini review. Ann Romanian Soc Cell Biol. 2021;25(4):709-695.
-
5.
Diniz UM, de Lima Nadia T, Mello MAR, Machado IC. Few plants and one dominant fly shape a unique pollination network in a Neotropical mangrove. Aquatic Botany. 2022;180:103526.
-
6.
Ochoa-Gomez JG, Acosta-Velazquez J, Anguamea-Valenzuela CA, Martinetto P. Distribution and structure of conocarpus erectus l.(combretaceae) in the northern limit of the pacific ocean (gulf of California). Ocean Coast Manag. 2021;209:105645.
-
7.
Bashir M, Uzair M, Chaudhry BA. A review of phytochemical and biological studies on Conocarpus erectus (Combretaceae). Pak J Pharm Res. 2015;1(1):1-8.
-
8.
Khalil R, Ali Q, Hafeez MM, Malik A. Phenolic acid profiling by RP-HPLC: Evaluation of antibacterial and anticancer activities of Conocarpus erectus plant extracts. Biol Clinical Sci Res J. 2020;2020(1).
-
9.
Al-Ameedi AI, Nahi H. Therapeutic effects of hydro-alcoholic extract of clove buds and econocapus erictus on infected wounds healing in dogs. Adv Anim Vet Sci. 2019;7(5):389-96.
-
10.
Shohayeb M, Abdel-Hameed E, Bazaid S. Antimicrobial activity of tannins and extracts of different parts of Conocarpus erectus L. Int J Pharm Bio Sci. 2013;3(2):544-53.
-
11.
Ayoub NA. A trimethoxyellagic acid glucuronide from Conocarpus erectus leaves: Isolation, characterization and assay of antioxidant capacity. Pharm Biol. 2010;48(3):328-32. [PubMed ID: 20645821]. https://doi.org/10.3109/13880200903131567.
-
12.
Zargaran Khouzani MR. Assessing the role, position and challenges facing the development of planting Conocarpus erectus L. in order to improve the environmental conditions affected by pollutants and dust (Case study on Ahvaz city). Cent Asian j environ sci technol. 2022;3(3):81-7.
-
13.
Kadim NA, AL-Azawi AH. Evaluation of the antibacterial activity of the polyherbal (conocarpus lancifolius l., capparis spinosa l. And dodonaea viscosa) leaves extracts cultivated iN IRAQ. Biochem Cell Arch. 2021;21(2):3873-80.
-
14.
Dayer D, Tabandeh MR, Moghimipour E, Hashemi Tabar M, Ghadiri A, Allah Bakhshi E, et al. MafA overexpression: A new efficient protocol for in vitro differentiation of adipose-derived mesenchymal stem cells into functional insulin-producing cells. Cell J. 2019;21(2):169-78. [PubMed ID: 30825290]. [PubMed Central ID: PMC6397604]. https://doi.org/10.22074/cellj.2019.5669.
-
15.
Hashemi Tabar M, Tabandeh MR, Moghimipour E, Dayer D, Ghadiri AA, Allah Bakhshi E, et al. The combined effect of Pdx1 overexpression and Shh manipulation on the function of insulin-producing cells derived from adipose-tissue stem cells. FEBS Open Bio. 2018;8(3):372-82. [PubMed ID: 29511614]. [PubMed Central ID: PMC5832980]. https://doi.org/10.1002/2211-5463.12378.
-
16.
Dayer D, Tabandeh MR, Kazemi M. The radio-sensitizing effect of pharmacological concentration of ascorbic acid on human pancreatic cancer cells. Anticancer Agents Med Chem. 2020;20(16):1927-32. [PubMed ID: 32532196]. https://doi.org/10.2174/1871520620666200612144124.
-
17.
Li L, Hwang E, Ngo HTT, Lin P, Gao W, Liu Y, et al. Antiphotoaging effect of prunus yeonesis blossom extract via inhibition of MAPK/AP-1 and regulation of the TGF-betaI/Smad and Nrf2/ARE signaling pathways. Photochem Photobiol. 2018;94(4):725-32. [PubMed ID: 29421853]. https://doi.org/10.1111/php.12894.
-
18.
Mirzajania R, Gorjian F, Kolahi M. [Study of phytochemical and anticancer effect hydroalcoholic extract leaves Conocarpus erectus L. Caco-2 cells on colorectal cancer]. Shahid Chamran University, Ahvaz, Iran. Iranian applied chemistry seminar; 2016. Persian.
-
19.
Xie S, Macedo P, Hew M, Nassenstein C, Lee KY, Chung KF. Expression of transforming growth factor-beta (TGF-beta) in chronic idiopathic cough. Respir Res. 2009;10(1):40. [PubMed ID: 19463161]. [PubMed Central ID: PMC2688489]. https://doi.org/10.1186/1465-9921-10-40.
-
20.
Redshaw N, Camps C, Sharma V, Motallebipour M, Guzman-Ayala M, Oikonomopoulos S, et al. TGF-beta/Smad2/3 signaling directly regulates several miRNAs in mouse ES cells and early embryos. PLoS One. 2013;8(1). e55186. [PubMed ID: 23390484]. [PubMed Central ID: PMC3559380]. https://doi.org/10.1371/journal.pone.0055186.
-
21.
Inchingolo AD, Cazzolla AP, Di Cosola M, Greco Lucchina A, Santacroce L, Charitos IA, et al. The integumentary system and its microbiota between health and disease. J Biol Regul Homeost Agents. 2021;35(2 Suppl. 1):303-21. [PubMed ID: 34281327]. https://doi.org/10.23812/21-2supp1-30.
-
22.
Krutmann J, Bouloc A, Sore G, Bernard BA, Passeron T. The skin aging exposome. J Dermatol Sci. 2017;85(3):152-61. [PubMed ID: 27720464]. https://doi.org/10.1016/j.jdermsci.2016.09.015.
-
23.
Yasin ZAM, Ibrahim F, Rashid NN, Razif MFM, Yusof R. The importance of some plant extracts as skin anti-aging resources: A review. Curr Pharm Biotechnol. 2017;18(11):864-76. [PubMed ID: 29256348]. https://doi.org/10.2174/1389201019666171219105920.
-
24.
Boo YC. Can plant phenolic compounds protect the skin from airborne particulate matter? Antioxidants (Basel). 2019;8(9). [PubMed ID: 31500121]. [PubMed Central ID: PMC6769904]. https://doi.org/10.3390/antiox8090379.
-
25.
Khan M, Liu H, Wang J, Sun B. Inhibitory effect of phenolic compounds and plant extracts on the formation of advance glycation end products: A comprehensive review. Food Res Int. 2020;130:108933. [PubMed ID: 32156381]. https://doi.org/10.1016/j.foodres.2019.108933.
-
26.
Sero L, Sanguinet L, Blanchard P, Dang BT, Morel S, Richomme P, et al. Tuning a 96-well microtiter plate fluorescence-based assay to identify AGE inhibitors in crude plant extracts. Molecules. 2013;18(11):14320-39. [PubMed ID: 24256925]. [PubMed Central ID: PMC6270619]. https://doi.org/10.3390/molecules181114320.
-
27.
Lin TK, Zhong L, Santiago JL. Anti-inflammatory and skin barrier repair effects of topical application of some plant oils. Int J Mol Sci. 2017;19(1). [PubMed ID: 29280987]. [PubMed Central ID: PMC5796020]. https://doi.org/10.3390/ijms19010070.
-
28.
Oves M, Ahmar Rauf M, Aslam M, Qari HA, Sonbol H, Ahmad I, et al. Green synthesis of silver nanoparticles by Conocarpus Lancifolius plant extract and their antimicrobial and anticancer activities. Saudi J Biol Sci. 2022;29(1):460-71. [PubMed ID: 35002442]. [PubMed Central ID: PMC8716933]. https://doi.org/10.1016/j.sjbs.2021.09.007.
-
29.
SOARES CLR. [Evaluation of in vitro and in vivo cicatrizing activity of Laguncularia racemosa (L) C.F. Gaertn]. Recife, Brazil: Universidade Federal de Pernambuco; 2018. Portuguese.
-
30.
Melo CMLD, Sousa GFD, Silva GADS, Silva RSD, Bezerra Júnior NDS, Santos DKDDN, et al. Pectin-like polysaccharide extracted from the leaves caesalpinia pulcherrima is a promising antioxidant and immunomodulator agent. Braz arch biol technol. 2022;65. https://doi.org/10.1590/1678-4324-2022200718.
-
31.
Da Silva Oliveira K, Da Silva A, Rodrigues M, Do Nascimento D, Barbosa J, Santana M. Histomorphometric analysis of reepitelization and collagen matrix of cutaneous wounds treated with hydroalcoolic extract from the leaves of conocarpus erectus linnaeus. Am J Clin Dermatol Res Rev. 2019;2(14).
-
32.
Yasin SA, Al-Azawi AH. Antibacterial activity of Conocarpus erectus leaves extracts on some microorganisms isolated from patients with burn infection. Plant Archives. 2019;19(2):583-9.
-
33.
Saadullah M, Asif M, Sattar A, Rehman K, Shah S, Saleem M, et al. Cytotoxic and antioxidant potentials of ellagic acid derivatives from Conocarpus lancifolius (Combretaceae). Trop J Pharm Res. 2020;19(5):1037-80.
-
34.
Alsaraf KM, Mohammad MH, Al-Shammari AM, Abbas IS. Investigating the anti-proliferation activity of Conocarpus erectus Against breast tumor cells in vitro. Sys Rev Pharm. 2020;11(12).
-
35.
Faraj AK, Shawkat MS. Cytotoxic effect of flavonoids extracted from conocarpus erectus leaves on hela cell and ref. Plant Archiv. 2020;20(1):3476-80.
-
36.
Santos D, de Almeida VS, de Araujo DRC, Harand W, Soares AKA, Moreira LR, et al. Evaluation of cytotoxic, immunomodulatory and antibacterial activities of aqueous extract from leaves of Conocarpus erectus Linnaeus (Combretaceae). J Pharm Pharmacol. 2018;70(8):1092-101. [PubMed ID: 29744882]. https://doi.org/10.1111/jphp.12930.
-
37.
Abdel-Hameed ES, Bazaid SA, Sabra ANA. Protective effect of conocarpus erectus extracts on CCl4-induced chronic liver injury in mice. Glob J Pharmacol. 2013;7(1):52-60.
-
38.
Nishimoto S, Kawai K, Tsumano T, Fukuda K, Fujiwara T, Kakibuchi M. Impacts of bone marrow aspirate and peripheral blood derived platelet-rich plasma on the wound healing in chronic ischaemic limb. J Plast Surg Hand Surg. 2013;47(3):169-74. [PubMed ID: 23621096]. https://doi.org/10.3109/2000656X.2012.752739.
-
39.
Bhatnagar P, Law JX, Ng S. Delivery systems for platelet derived growth factors in wound healing: A review of recent developments and global patent landscape. J Drug Deliv Sci Technol. 2022;71. https://doi.org/10.1016/j.jddst.2022.103270.
-
40.
Verrecchia F, Chu ML, Mauviel A. Identification of novel TGF-beta /Smad gene targets in dermal fibroblasts using a combined cDNA microarray/promoter transactivation approach. J Biol Chem. 2001;276(20):17058-62. [PubMed ID: 11279127]. https://doi.org/10.1074/jbc.M100754200.
-
41.
Philipp K, Riedel F, Sauerbier M, Hormann K, Germann G. Targeting TGF-beta in human keratinocytes and its potential role in wound healing. Int J Mol Med. 2004;14(4):589-93. [PubMed ID: 15375586].
-
42.
Cutroneo KR. TGF-beta-induced fibrosis and SMAD signaling: Oligo decoys as natural therapeutics for inhibition of tissue fibrosis and scarring. Wound Repair Regen. 2007;15 Suppl 1:S54-60. [PubMed ID: 17727468]. https://doi.org/10.1111/j.1524-475X.2007.00226.x.
-
43.
Carlson MA, Longaker MT. The fibroblast-populated collagen matrix as a model of wound healing: A review of the evidence. Wound Repair Regen. 2004;12(2):134-47. [PubMed ID: 15086764]. https://doi.org/10.1111/j.1067-1927.2004.012208.x.
-
44.
Zhao J, Shi W, Wang YL, Chen H, Bringas PJ, Datto MB, et al. Smad3 deficiency attenuates bleomycin-induced pulmonary fibrosis in mice. Am J Physiol Lung Cell Mol Physiol. 2002;282(3):L585-93. [PubMed ID: 11839555]. https://doi.org/10.1152/ajplung.00151.2001.