Abstract
Background:
As the repair capacity of the nervous system is low, stem cell therapy is a trend for replacement therapy. Dental pulp stem cells (DPSCs) have the potential to differentiate into many tissues, such as neurons. Harmine (7-methoxy-1methyl-9H-pyrido[3,4-b] indole) is an alkaloidal component of medicinal plants with a long history in traditional medicine. Alginate is a biocompatible hydrogel widely used as a biomaterial base in various scaffolds.Objectives:
This study investigated whether harmine and encapsulation of cells in alginate hydrogel could improve DPSCs differentiation into neural cells.Methods:
DPSCs were cultured under standard stem cell culture conditions, then encapsulated in alginate hydrogel, and treated with differentiation medium with and without harmine. After 14 days, cell proliferation and differentiation were assessed by the 3-(4,5-dimethylthiazol-2-yl)-2,5-diphenyl-2H-tetrazolium bromide (MTT) assay, real-time polymerase chain reaction (RT-PCR), and flow cytometry.Results:
Harmine (5 and 10 μM) significantly increased the proliferation and viability of DPSCs compared to the control group in both two-dimensional and three-dimensional culture systems (P < 0.05). The expression levels of three neural cell markers (nestin, microtubule-associated protein [MAP-2], and β-tubulin III) in DPSCs-derived neural cells cultured in two-dimensional and three-dimensional culture systems were significantly increased in harmine-treated two-dimensional and three-dimensional culture systems compared to the control group (P < 0.05).Conclusions:
Either harmine or alginate hydrogel had an accelerating effect on DPSCs differentiation into neural cells. Harmine also increased the proliferation of the cells.Keywords
Alginate Cell Differentiation Dental Pulp Harmine Hydrogels Neurons Stem Cells
1. Background
Nerve injury and neurodegenerative diseases are among the clinical problems due to the low repair rate in the nervous system, often leading to permanent sensory and motor disabilities. Stem cells derived from many sources, including dental pulp, can remarkably differentiate into tissues, such as neurons. On the other hand, by setting up both two-dimensional and three-dimensional culture systems, neural tissue engineering aims to develop biomaterial scaffolds for neuron regeneration after nerve damage and neurodegenerative diseases (1). In addition, medicinal plants and their derivatives could improve stem cell differentiation. However, there are no reports on the effect of harmine (7-methoxy-1methyl-9H-pyrido[3,4-b] indole) on dental pulp stem cells (DPSCs) differentiation into neurons in either two-dimensional or three-dimensional culture systems.
In tissue engineering, cells are usually cultured on a scaffold similar to the extracellular matrix of soft tissues to develop a three-dimensional culture system (1). A three-dimensional culture system (2) is more reliable than a two-dimensional culture system regarding cell growth, cell metabolism, gene expression, extracellular matrix proteins, and cell morphology (3). Furthermore, scaffolds are highly hydrated three-dimensional networks of cross-linked hydrophilic polymers swelling greatly in aqueous media (4). The space and mechanical stability created by these cross-links can be useful for filling irregular defects created by injury and forming new tissue (5-7).
The three-dimensional alginate hydrogel scaffold has attracted attention due to its ease of preparation and ability to encapsulate cells (8-12). Alginate is a water-soluble polysaccharide isolated from brown algae, consisting of D-mannuronic acid (M) and guluronic acid (G)-L monomers due to its ability to jellify and its high viscosity in aqueous solutions (13-16). Alginates have useful properties, such as biocompatibility and non-stimulation of the immune system, probably related to their hydrophilic property (17), and is also abundant, inexpensive, and non-toxic (11). As cells are not damaged during the process of gelation and formation of ionic cross-links, alginates are adequately used for drug release, cell encapsulation, and tissue regeneration (18-23).
Mesenchymal stem cells, present in various human tissues and organs, have been reported to have the capacity for self-renewal and multilineage differentiation and the potential for regenerative cell therapy. They can differentiate into osteocytes, chondrocytes, adipocytes, and odontoblasts (24). However, the lack of endogenous stem cells is one of the major limitations in this field (25). Therefore, it is necessary to find new sources for them. DPSCs isolated from dental pulp tissue have osteogenic, dentinogenic, adipogenic, chondrogenic, myogenic, neurogenic, and regenerative potentials than many other stem cells (25, 26).
Harmine, a tricyclic β-carboline alkaloid, was originally isolated from various medicinal plants, such as the seeds of Peganum harmala and Banisteriopsis caapi (27), and has been used for medicinal purposes in the Middle East and Asia (28). In vitro and in vivo studies have shown that harmine is involved in many cellular and molecular processes, including anti-tumor, osteoblast differentiation, and antioxidation (29).
Accordingly, harmine has the potential to affect cellular and molecular cascades, pulp-derived stem cells could differentiate into neural cells, and the three-dimensional culture system is better than the two-dimensional culture system.
2. Objectives
This study was conducted to investigate the effect of harmine on DPSCs differentiation into neural cells in two-dimensional and three-dimensional cultures.
3. Methods
3.1. Cell Culture and Encapsulation in Alginate Hydrogel
The DPSCs line was obtained from the Iranian Biological Resource Center and cultured under standard conditions with α-minimum essential medium (α-MEM) culture medium (Gibco, Germany) and 20% fetal bovine serum (FBS) (Gibco, Germany) and maintained in 5% carbon dioxide (CO2) at 37°C.
The cells were divided into 6 groups: Two-dimensional cultures (3 groups) and three-dimensional cultures (3 groups). The experiments were prolonged for 14 days, and the doses of harmine were determined according to the 3-(4,5-dimethylthiazol-2-yl)-2,5-diphenyl-2H-tetrazolium bromide (MTT) assay. In the two-dimensional culture, cells were not treated with any reagents in the control group or treated with harmine (5 or 10 μM). In the three-dimensional culture, the control group was cultured in encapsulated alginate solution (1.2% v/w), while in two harmine groups, harmine (5 or 10 μM) was added to culture media containing encapsulated alginate solution (1.2% v/w).
For encapsulation, 1 × 106 cells were transferred to a 5 mL syringe containing 1 mL alginate solution (1.2% v/w) (Gibco, Germany). The alginate solution containing the stem cells was dropped into the wells of 24-well plates containing calcium chloride (CaCl2) solution (100 mM) (Gibco, Germany). The plate was incubated for half an hour. The CaCl2 solution was discarded, and the wells were washed with 20 mM Tris buffer and culture medium. The culture medium was added to the wells, and the plates were returned to the incubator.
3.2. The 3-(4,5-Dimethylthiazol-2-yl)-2,5-Diphenyl-2H-Tetrazolium Bromide Assays for Cell Proliferation
The MTT assay was performed to find non-toxic concentrations of harmine. Cells were seeded in 96-well plates (15×103 cells/well) and incubated overnight. The medium on the cells was replaced with fresh serum-free medium containing 0, 0.1, 1, 5, 10, 20, 40, 80, and 100 μM harmine and incubated. After 14 days, the medium was discarded, and after washing with phosphate-buffered saline (PBS) (Invitrogen Life Technologies, Germany), 50 μL MTT solution (Sigma, USA) (5 mg/mL in PBS) was added to the wells. The cells were incubated in the dark for 3 hours, 200 μL dimethyl sulfoxide was added to each well, and the plate was shaken for 20 minutes. The absorbance was then read using an enzyme-linked immunosorbent assay (ELISA) reader at 570 and 630 nm. Cell proliferation was calculated using the following formula:
Viability (%) = (Absorbance of treated cells / Absorbance of control cells) × 100
This test was also used to assess the proliferation and survival rates of cells encapsulated in alginate hydrogel after 14 days.
3.3. Neural Induction
After proliferation and from the third passage onwards, the cells were divided into two groups of two-dimensional and three-dimensional cultures. In each group, there were subgroups of control and harmine treated (5 μM and 10 μM). The following protocol was used to induce DPSCs differentiation: 20 ng/mL fibroblast growth factor 2 (FGF-2) (Sigma, USA), 20 ng/mL epidermal growth factor (EGF) (Sigma, USA), and 2% B27 were added to the cells cultured in Neurobasal-A medium (Sigma, USA) for 14 days. The differentiation medium was changed every 3 days. After 14 days, the cells were depolymerized with ethylenediaminetetraacetie acid (EDTA) and prepared for flow cytometry and gene expression analysis.
3.4. Flow Cytometry
After treatment, the cells were detached with trypsin/EDTA solution (Sigma, Germany) and suspended after washing with PBS. The cells were permeabilized with Triton X-100 (Sigma, Germany) and incubated with a blocking buffer (10% normal goat serum [NGS]) (Sigma, Germany). Primary and secondary antibodies (Sigma, Germany) were added to the cells, mixed, and incubated for 30 min at room temperature in the dark. The samples were washed twice with PBS, the supernatant was discarded after centrifugation at 200 × g for 5 min at room temperature, and the cells were resuspended in PBS containing 1% paraformaldehyde (Sigma, Germany) to fix the cells. Finally, 300 µl of PBS was added. The assay used a FACStarPLUS flow cytometer (BD Biosciences, Franklin Lakes, NJ, USA). Flow cytometry data were analyzed using FlowJo analysis software (Tree Star Inc., Ashland, OR, USA).
3.5. Gene Expression Assay
According to the instructions, total ribonucleic acid (RNA) was extracted using the RNA extraction kit (Takara, Shiga, Japan). The obtained RNA was used to synthesize complementary deoxyribose nucleic acid (cDNA) using the cDNA synthesis kit (Takara, Shiga, Japan). Real-time polymerase chain reaction (RT-PCR) was performed using SYBR Premix Ex Taq technology (Takara Bio Inc., Japan) on the Applied Biosystems StepOne RT-PCR System (Life Technologies, USA). The human glyceraldehyde-3-phosphate dehydrogenase (GAPDH) gene was analyzed synchronously as an endogenous control. The relative expression of the target genes was then quantified using the 2-ΔΔCt method. Primer sequences are listed in Table 1.
Primer Sequences
Genes | Sequences (5’ - 3’) |
---|---|
Nestin | |
F | TCAAGATGTCCCTCAGCCTGGA |
R | AAGCTGAGGGAAGTCTTGGAGC |
MAP-2 | |
F | AGGCTGTAGCAGTCCTGAAAGG |
R | CTTCCTCCACTGTGACAGTCTG |
Β-tubulin ІІІ | |
F | GTCCGCCTGCCTCTTCGTCTCTA |
R | GGCCCCTATCTGGTTGCCGCACT |
GAPDH | |
F | TCCCTGAGCTGAACGGGAAG |
R | GGAGGAGTGGGTGTCGCTGT |
3.6. Statistical Analysis
Data were analyzed by one-way analysis of variance followed by post hoc Tukey's test using SPSS software version 16.0 (Chicago, USA). All values were mean ± standard error of the mean and were representative of three independent experiments, and P < 0.05 was considered a statistically significant difference.
4. Results
4.1. Effects of Harmine and Alginate Hydrogel on the Survival of Dental Pulp Stem Cells
The effects of treatment with 0, 0.1, 1, 5, 10, 20, 40, 80, and 100 µM were evaluated by the MTT method (Figure 1). Concentrations of 0.1 and 80 µM harmine had no significant effect on cell proliferation and survival (P < 0.05), while concentrations of 5 and 10 μM harmine significantly increased cell proliferation (P < 0.05). At 10 μM harmine, cell viability was significantly decreased (P < 0.05). The highest survival and proliferation rates were observed at 5 μM harmine. Due to the lack of toxicity at 5 and 10 μM harmine, these concentrations were used for further studies. The alginate hydrogel also showed no cytotoxic effect on the viability of the encapsulated cells.
The effects of different concentrations of harmine on dental pulp stem cells. Cell proliferation was determined by the 3-(4,5-dimethylthiazol-2-yl)-2,5-diphenyl-2H-tetrazolium bromide assay. Data are expressed as a percentage of control cells as means ± standard deviation. **; P < 0.01, and ***; P < 0.01 compared to control.
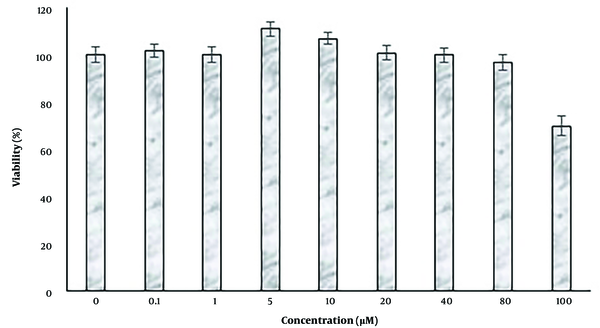
4.2. Effects of Harmine on Nestin, Microtubule-Associated Protein, and β-tubulin III Protein Expression Levels in Dental Pulp Stem Cells-Derived Neural Cells in Two-Dimensional Cultures
The expression levels of the neural cell markers of nestin, MAP-2, and β-tubulin III in DPSCs cells were evaluated by flow cytometry assay. The nestin expression level in the control cells was 6.14%, while the cells treated with 5 and 10 µM harmine showed 34.9% and 56% nestin expression levels, respectively, showing a significant increase (Figure 2A). The MAP-2 expression level in the control group was 9.49%, while the cells treated with 5 and 10 µM harmine showed 38% and 43.5% expression levels, respectively. Thus, the expression level of MAP-2 was significantly increased by harmine treatment (Figure 2B). Similarly, the expression levels of β-tubulin III were 13.4%, 47.9%, and 51.9% in cells treated with 0, 5, and 10 µM harmine, respectively. Thus, the expression level of β-tubulin ІІІ was significantly increased by harmine treatment (Figure 2C).
The effect of harmine on dental pulp stem cells in two-dimensional cultures. Neural cell marker proteins of (A) nestin, (B) microtubule-associated protein, and (C) β-tubulin III proteins were determined by flow cytometry assay.
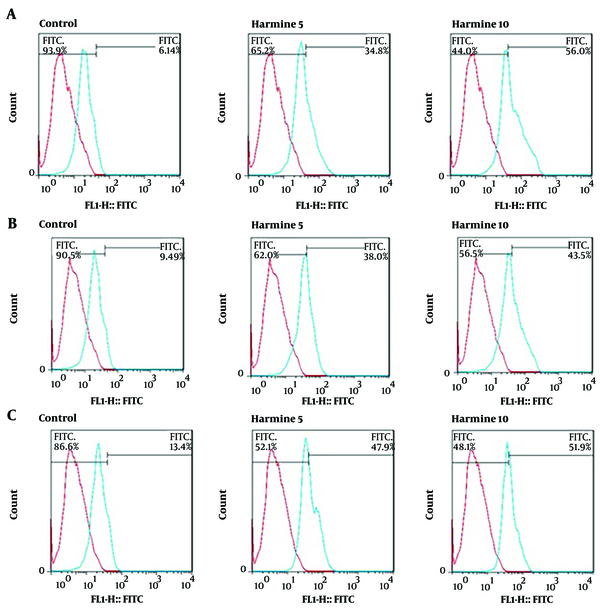
4.3. Effects of Harmine on Nestin, Microtubule-Associated Protein, and β-tubulin III Protein Expression Levels in Dental Pulp Stem Cells-Derived Neural Cells in Tree-Dimensional Cultures
The expression levels of the neural cell marker proteins nestin, MAP-2, and β-tubulin III in DPSCs-derived neural cells cultured in three-dimensional culture were evaluated by flow cytometry assay. At day 14 of differentiation, the mean nestin expression in cells in alginate hydrogel was 28.3%, and the cells treated with 5 and 10 µM harmine showed 60.6% and 71.5% nestin expression levels, respectively. So, nestin expression was significantly increased by harmine treatment (Figure 3A). The mean MAP-2 expression level in DPSCs-derived neural cells in alginate hydrogel was 35.1%, and the cells treated with 5 and 10 µM harmine showed 57.6% and 73.5% expression levels, respectively. Thus, the expression level of MAP-2 was significantly increased by harmine treatment (Figure 3B). Similarly, the β-tubulin III expression levels were 35.9%, 58.5%, and 73.2% in cells treated with 0, 5, and 10 µM harmine, respectively. Thus, the β-tubulin ІІІ expression level was significantly increased by harmine treatment (Figure 3C).
The effect of harmine on dental pulp stem cells in three-dimensional cultures. Neural cell marker proteins of (A) nestin, (B) MAP-2, and (C) β-tubulin III were determined by flow cytometry assay.
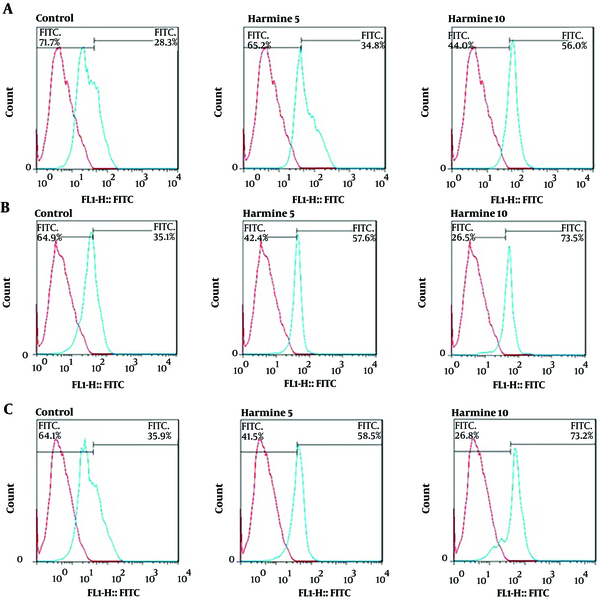
4.4. Effects of Harmine on Nestin, Microtubule-Associated Protein, and β-tubulin III mRNA Expression Levels in Dental Pulp Stem Cells-Derived Neural Cells in Two-Dimensional Cultures
The expression levels of the neural differentiation marker genes, including nestin, MAP-2, and β-tubulin III, were examined by RT-PCR (Figure 4). Concentrations of 5 and 10 μM harmine significantly increased the expression levels of these markers (P < 0.05). The expression levels of all three markers increased after 14 days when the cells were exposed to the differentiation medium.
The effect of harmine on dental pulp stem cells in two-dimensional cultures. The expression levels of neural cell marker genes, including nestin, MAP-2, and β-tubulin III, were determined by real-time polymerase chain reaction assay. Data are expressed as a percentage of control cells as mean ± standard deviation ***; P < 0.01 compared to control.
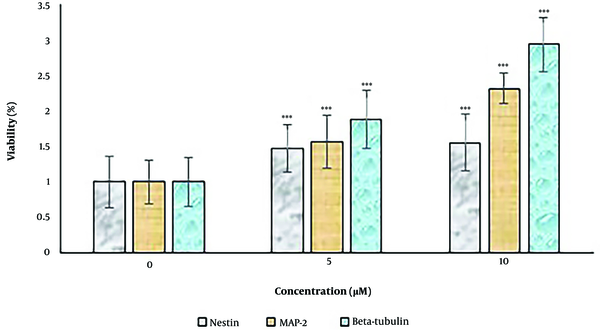
4.5. Effects of Harmine on Nestin, Microtubule-Associated Protein, and β-tubulin III mRNA Expression Levels in Dental Pulp Stem Cells-Derived Neural Cells in Three-Dimensional Cultures
The expression levels of the neural differentiation marker genes, including nestin, MAP-2, and β-tubulin III, were examined by RT-PCR (Figure 5). Concentrations of 5 and 10 μM harmine significantly increased the expression levels of these markers (P < 0.05). The expression levels of all three markers were increased in the alginate hydrogel.
The effect of harmine on dental pulp stem cells in three-dimensional cultures. The expression levels of neural cell marker genes, including (A) nestin, (B) microtubule-associated protein, and (C) β-tubulin III, were determined by real-time polymerase chain reaction assay. Data are expressed as a percentage of control cells as mean ± standard deviation ***; P < 0.01 compared to control.
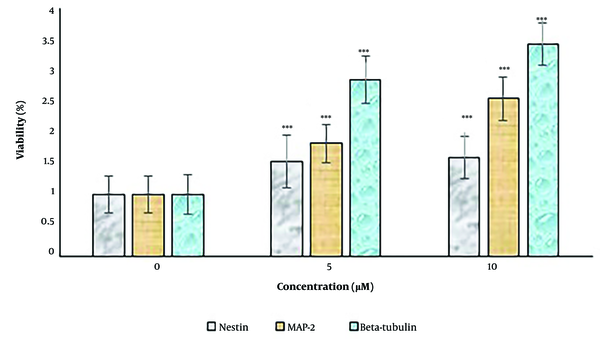
5. Discussion
The present study demonstrated an accelerating effect of harmine on DPSCs differentiation into neurons in two-dimensional and three-dimensional culture systems. The data indicated that alginate hydrogel provided a suitable three-dimensional environment for the DPSCs culture and neural differentiation.
Alginate hydrogel at 1.2% w/v did not affect the viability or proliferation rates of the cells. In the present study, DPSCs were transferred to 1.2% alginate hydrogel and cultured in a neural differentiation medium. Ashton et al. cultured rat neural progenitor cells in 1% alginate hydrogel with poly lactic-co-glycolic acid-aluminum (PLGA-Al) for 7 days and observed a significant increase in the proliferation rate of these cells (30). In Ashton et al.’s study, the presence of PLGA-Al together with alginate hydrogel may have increased the proliferation rate of rat neural progenitor cells by controlling the degradation of this hydrogel; the type of cells and the concentration of alginate used in the two studies are also different. The behavior of different cells in alginate hydrogels has been studied, and different results have been obtained (30). These results may be related to the specific cell type or the method used to encapsulate the cells (31). Since alginate does not interfere with cell function, cells cannot adhere to the alginate network. Cell adhesion is necessary for most cells’ survival, proliferation, differentiation, and gene expression (32). The non-adhesive nature of the alginate hydrogel promotes cell-cell interaction, which is important for maintaining cell survival and enhancing the functional properties of cells (33).
In this study, it was found that 5 and 10 µM harmine increased the proliferation of DPSCs. In Title et al.’s study, 4 days of harmine treatment (1, 3.3, 5, and 10 µM) increased pancreatic β-cell proliferation. Although the duration of treatment in the two studies is different, the results are completely consistent (34). In contrast to our study, one study showed that concentrations of 1, 3, and 10 μM harmine had no effect on osteoblast cell proliferation. The reason for this contradiction is the possibility of a difference in cell type, showing that harmine does not have a general effect (35).
It was found in this study that 5 and 10 μM harmine increased and accelerated stem cell differentiation. A previous study showed that harmine increased osteoblast differentiation (35).
Flow cytometry test indicated that the mean percentage of nestin, MAP-2, and β-tubulin ІІІ positive cells increased in cells treated with harmine compared to the control cells; also, according to the results of the RT-PCR test, the expression levels of all three genes, including nestin, MAP-2, and β-tubulin ІІІ, increased in cells treated with harmine compared to the control cells. This study is the first research to report the accelerating effect of harmine on DPSCs differentiation into neurons. A previous study showed that harmine could increase osteoblast differentiation by inducing bone morphogenetic protein (BMP) expression and activating the BMP and Runx2 signaling pathways (35).
Encapsulated cells in alginate hydrogel differentiated into neuron-like cells more than cells in two-dimensional cultures, with an increase in the mean percentage of nestin, MAP-2, and β-tubulin ІІІ positive cells compared to the control cells. Also, according to the results of the RT-PCR test, the expression levels of nestin, MAP-2, and β-tubulin ІІІ genes increased in encapsulated cells compared to the control cells. This effect has been reported in previous studies. Banerjee et al. cultured mature neural stem cells isolated from rat hippocampus in alginate at a concentration of 0.25% or 1%. Their experiments showed that when examining the neural differentiation of these cells, the expression level of the β-tubulin Ш marker (neural differentiation marker) was higher in cells cultured in alginate at a concentration of 0.25% (36). These results were consistent with the results of our recent investigation. Xei et al. also cultured stem cells isolated from rat adipose tissue in peptide hydrogel and neuro-induction medium. Ten days after neural differentiation, the immunocytochemical analysis showed that these cells expressed nestin and bromodeoxyuridine (BrdU) (cell proliferation marker) in the center of the formed cell masses (37). The results of the present study showed a significant increase in nestin expression in cells encapsulated in alginate hydrogel after 14 days of neural differentiation. Although the type of scaffold used in the two studies and the type of cells are different, it is possible that the hydrophilic state created by both scaffolds contributes to similar results. Read et al. showed that the rat glioma BT4C cell line encapsulated in 1.5% alginate hydrogel, cultured in vivo proliferated, and survived 4 months after culture (9). In contrast, in more recent experiments, DPSCs encapsulated in 1.2% alginate hydrogel and cultured in vitro showed no proliferation. The difference in cell type and concentration of alginate used, and also the in vivo conditions in Read et al.’s study, may explain the different results in the two studies. Purcell et al. cultured mouse cortical neural stem cells in 1% alginate and observed increased survival and proliferation of these cells from day 7 to day 21, in addition to larger proliferating cell masses expressing nestin (38). In contrast to these results, the proliferation of DPSCs encapsulated in alginate hydrogel did not change in the present study. In our study, the expression of nestin increased. Likely, the different cell types and concentrations of alginate used in the two studies led to different results. Matyash et al. cultured human and rat neural stem cells on a 1% or 0.2% alginate hydrogel neural medium. These cells had longer neurites than the control group. Moreover, 60 - 70% of the cells were positive for MAP-2 or β-tubulin Ш markers (39). The results of this research showed that DPSCs encapsulated in 1.2% alginate hydrogel were positive for nestin, β-tubulin ІІІ, and MAP-2.
5.1. Conclusions
The study presented harmine as a neural differentiator of pulp stem cells, which also improved cell proliferation and viability of these cells. Furthermore, alginate hydrogel is suitable for setting up a three-dimensional culture system for differentiating pulp stem cells into neurons. The use of alginate in this three-dimensional system is more effective than a two-dimensional culture system and may be based on more similarity to natural cell attachments and cell-cell interactions.
Acknowledgements
References
-
1.
Hunt NC, Smith AM, Gbureck U, Shelton RM, Grover LM. Encapsulation of fibroblasts causes accelerated alginate hydrogel degradation. Acta Biomater. 2010;6(9):3649-56. [PubMed ID: 20307693]. https://doi.org/10.1016/j.actbio.2010.03.026.
-
2.
Moroni L, de Wijn JR, van Blitterswijk CA. 3D fiber-deposited scaffolds for tissue engineering: influence of pores geometry and architecture on dynamic mechanical properties. Biomaterials. 2006;27(7):974-85. [PubMed ID: 16055183]. https://doi.org/10.1016/j.biomaterials.2005.07.023.
-
3.
Dutta RC, Dutta AK. Cell-interactive 3D-scaffold; advances and applications. Biotechnol Adv. 2009;27(4):334-9. [PubMed ID: 19232387]. https://doi.org/10.1016/j.biotechadv.2009.02.002.
-
4.
Lee KY, Mooney DJ. Hydrogels for tissue engineering. Chem Rev. 2001;101(7):1869-79. [PubMed ID: 11710233]. https://doi.org/10.1021/cr000108x.
-
5.
Nicodemus GD, Bryant SJ. Cell encapsulation in biodegradable hydrogels for tissue engineering applications. Tissue Eng Part B Rev. 2008;14(2):149-65. [PubMed ID: 18498217]. [PubMed Central ID: PMC2962861]. https://doi.org/10.1089/ten.teb.2007.0332.
-
6.
Lee KY, Alsberg E, Mooney DJ. Degradable and injectable poly (aldehyde guluronate) hydrogels for bone tissue engineering. J Biomed Mater Res. 2001;56(2):228-33. [PubMed ID: 11340593].
-
7.
Peter SJ, Miller MJ, Yasko AW, Yaszemski MJ, Mikos AG. Polymer concepts in tissue engineering. J Biomed Mater Res. 1998;43(4):422-7.
-
8.
Li X, Liu T, Song K, Yao L, Ge D, Bao C, et al. Culture of neural stem cells in calcium alginate beads. Biotechnol Prog. 2006;22(6):1683-9. [PubMed ID: 17137318]. https://doi.org/10.1021/bp060185z.
-
9.
Read TA, Stensvaag V, Vindenes H, Ulvestad E, Bjerkvig R, Thorsen F. Cells encapsulated in alginate: A potential system for delivery of recombinant proteins to malignant brain tumours. Int J Dev Neurosci. 1999;17(5-6):653-63. [PubMed ID: 10571425]. https://doi.org/10.1016/s0736-5748(99)00052-0.
-
10.
Rowley JA, Madlambayan G, Mooney DJ. Alginate hydrogels as synthetic extracellular matrix materials. Biomaterials. 1999;20(1):45-53. [PubMed ID: 9916770]. https://doi.org/10.1016/s0142-9612(98)00107-0.
-
11.
Augst AD, Kong HJ, Mooney DJ. Alginate hydrogels as biomaterials. Macromol Biosci. 2006;6(8):623-33. [PubMed ID: 16881042]. https://doi.org/10.1002/mabi.200600069.
-
12.
Drury JL, Mooney DJ. Hydrogels for tissue engineering: scaffold design variables and applications. Biomaterials. 2003;24(24):4337-51. [PubMed ID: 12922147]. https://doi.org/10.1016/s0142-9612(03)00340-5.
-
13.
Li T, Shi XW, Du YM, Tang YF. Quaternized chitosan/alginate nanoparticles for protein delivery. J Biomed Mater Res A. 2007;83(2):383-90. [PubMed ID: 17450586]. https://doi.org/10.1002/jbm.a.31322.
-
14.
Kemp MR, Fryer PJ. Enhancement of diffusion through foods using alternating electric fields. Innov Food Sci Emerg Technol. 2007;8(1):143-53. https://doi.org/10.1016/j.ifset.2006.09.001.
-
15.
Prang P, Muller R, Eljaouhari A, Heckmann K, Kunz W, Weber T, et al. The promotion of oriented axonal regrowth in the injured spinal cord by alginate-based anisotropic capillary hydrogels. Biomaterials. 2006;27(19):3560-9. [PubMed ID: 16500703]. https://doi.org/10.1016/j.biomaterials.2006.01.053.
-
16.
Petruzzo P, Cappai A, Ruiu G, Dessy E, Rescigno A, Brotzu G. Development of biocompatible barium alginate microcapsules. Transplant Proc. 1997;29(4):2129-30. [PubMed ID: 9193556]. https://doi.org/10.1016/s0041-1345(97)00290-x.
-
17.
Shapiro L, Cohen S. Novel alginate sponges for cell culture and transplantation. Biomaterials. 1997;18(8):583-90. [PubMed ID: 9134157]. https://doi.org/10.1016/s0142-9612(96)00181-0.
-
18.
Coviello T, Matricardi P, Marianecci C, Alhaique F. Polysaccharide hydrogels for modified release formulations. J Control Release. 2007;119(1):5-24. [PubMed ID: 17382422]. https://doi.org/10.1016/j.jconrel.2007.01.004.
-
19.
Simmons CA, Alsberg E, Hsiong S, Kim WJ, Mooney DJ. Dual growth factor delivery and controlled scaffold degradation enhance in vivo bone formation by transplanted bone marrow stromal cells. Bone. 2004;35(2):562-9. [PubMed ID: 15268909]. https://doi.org/10.1016/j.bone.2004.02.027.
-
20.
Kong HJ, Mooney DJ. The effects of poly (ethyleneimine) (PEI) molecular weight on reinforcement of alginate hydrogels. Cell Transplant. 2003;12(7):779-85. [PubMed ID: 14653624]. https://doi.org/10.3727/000000003108747253.
-
21.
Alsberg E, Kong HJ, Hirano Y, Smith MK, Albeiruti A, Mooney DJ. Regulating bone formation via controlled scaffold degradation. J Dent Res. 2003;82(11):903-8. [PubMed ID: 14578503]. https://doi.org/10.1177/154405910308201111.
-
22.
Alsberg E, Anderson KW, Albeiruti A, Franceschi RT, Mooney DJ. Cell-interactive alginate hydrogels for bone tissue engineering. J Dent Res. 2001;80(11):2025-9. [PubMed ID: 11759015]. https://doi.org/10.1177/00220345010800111501.
-
23.
Hsiong SX, Huebsch N, Fischbach C, Kong HJ, Mooney DJ. Integrin-adhesion ligand bond formation of preosteoblasts and stem cells in three-dimensional RGD presenting matrices. Biomacromolecules. 2008;9(7):1843-51. [PubMed ID: 18540674]. [PubMed Central ID: PMC2853778]. https://doi.org/10.1021/bm8000606.
-
24.
Huang GT, Gronthos S, Shi S. Mesenchymal stem cells derived from dental tissues vs. those from other sources: their biology and role in regenerative medicine. J Dent Res. 2009;88(9):792-806. [PubMed ID: 19767575]. [PubMed Central ID: PMC2830488]. https://doi.org/10.1177/0022034509340867.
-
25.
Chen L, Zheng L, Jiang J, Gui J, Zhang L, Huang Y, et al. Calcium Hydroxide-induced Proliferation, Migration, Osteogenic Differentiation, and Mineralization via the Mitogen-activated Protein Kinase Pathway in Human Dental Pulp Stem Cells. J Endod. 2016;42(9):1355-61. [PubMed ID: 27395474]. https://doi.org/10.1016/j.joen.2016.04.025.
-
26.
Mitsiadis TA, Feki A, Papaccio G, Caton J. Dental pulp stem cells, niches, and notch signaling in tooth injury. Adv Dent Res. 2011;23(3):275-9. [PubMed ID: 21677078]. https://doi.org/10.1177/0022034511405386.
-
27.
Patel K, Gadewar M, Tripathi R, Prasad SK, Patel DK. A review on medicinal importance, pharmacological activity and bioanalytical aspects of beta-carboline alkaloid ''Harmine''. Asian Pac J Trop Biomed. 2012;2(8):660-4. [PubMed ID: 23569990]. [PubMed Central ID: PMC3609365]. https://doi.org/10.1016/S2221-1691(12)60116-6.
-
28.
Berrougui H, Martin-Cordero C, Khalil A, Hmamouchi M, Ettaib A, Marhuenda E, et al. Vasorelaxant effects of harmine and harmaline extracted from Peganum harmala L. seeds in isolated rat aorta. Pharmacol Res. 2006;54(2):150-7. [PubMed ID: 16750635]. https://doi.org/10.1016/j.phrs.2006.04.001.
-
29.
Chen Q, Chao R, Chen H, Hou X, Yan H, Zhou S, et al. Antitumor and neurotoxic effects of novel harmine derivatives and structure-activity relationship analysis. Int J Cancer. 2005;114(5):675-82. [PubMed ID: 15609303]. https://doi.org/10.1002/ijc.20703.
-
30.
Ashton RS, Banerjee A, Punyani S, Schaffer DV, Kane RS. Scaffolds based on degradable alginate hydrogels and poly(lactide-co-glycolide) microspheres for stem cell culture. Biomaterials. 2007;28(36):5518-25. [PubMed ID: 17881048]. https://doi.org/10.1016/j.biomaterials.2007.08.038.
-
31.
Novikova LN, Mosahebi A, Wiberg M, Terenghi G, Kellerth JO, Novikov LN. Alginate hydrogel and matrigel as potential cell carriers for neurotransplantation. J Biomed Mater Res A. 2006;77(2):242-52. [PubMed ID: 16392134]. https://doi.org/10.1002/jbm.a.30603.
-
32.
Retta SF, Ternullo M, Tarone G. Adhesion to matrix proteins. Methods Mol Biol. 1999;96:125-30. [PubMed ID: 10098129]. https://doi.org/10.1385/1-59259-258-9:125.
-
33.
Glicklis R, Shapiro L, Agbaria R, Merchuk JC, Cohen S. Hepatocyte behavior within three-dimensional porous alginate scaffolds. Biotechnol Bioeng. 2000;67(3):344-53.
-
34.
Title AC, Karsai M, Mir-Coll J, Grining OY, Rufer C, Sonntag S, et al. Evaluation of the effects of harmine on beta-cell function and proliferation in standardized human islets using 3D high-content confocal imaging and automated analysis. Front Endocrinol (Lausanne). 2022;13:854094. [PubMed ID: 35860702]. [PubMed Central ID: PMC9289187]. https://doi.org/10.3389/fendo.2022.854094.
-
35.
Yonezawa T, Lee JW, Hibino A, Asai M, Hojo H, Cha BY, et al. Harmine promotes osteoblast differentiation through bone morphogenetic protein signaling. Biochem Biophys Res Commun. 2011;409(2):260-5. [PubMed ID: 21570953]. https://doi.org/10.1016/j.bbrc.2011.05.001.
-
36.
Banerjee A, Arha M, Choudhary S, Ashton RS, Bhatia SR, Schaffer DV, et al. The influence of hydrogel modulus on the proliferation and differentiation of encapsulated neural stem cells. Biomaterials. 2009;30(27):4695-9. [PubMed ID: 19539367]. [PubMed Central ID: PMC2743317]. https://doi.org/10.1016/j.biomaterials.2009.05.050.
-
37.
Xie X, Tang Z, Chen J, Yang J, Zeng W, Liu N, et al. Neurogenesis of adipose-derived stem cells in hydrogel. J Huazhong Univ Sci Technolog Med Sci. 2011;31(2):174-7. [PubMed ID: 21505979]. https://doi.org/10.1007/s11596-011-0246-1.
-
38.
Purcell EK, Singh A, Kipke DR. Alginate composition effects on a neural stem cell-seeded scaffold. Tissue Eng Part C Methods. 2009;15(4):541-50. [PubMed ID: 19368511]. [PubMed Central ID: PMC2819710]. https://doi.org/10.1089/ten.tec.2008.0302.
-
39.
Matyash M, Despang F, Mandal R, Fiore D, Gelinsky M, Ikonomidou C. Novel soft alginate hydrogel strongly supports neurite growth and protects neurons against oxidative stress. Tissue Eng Part A. 2012;18(1-2):55-66. [PubMed ID: 21770866]. https://doi.org/10.1089/ten.TEA.2011.0097.