Abstract
Keywords
Allium atroviolaceum Extract Antibacterial Streptococcus viridans Groups
1. Background
Oral and dental problems continue to pose a significant health concern in Indonesia. According to Indonesia's National Basic Health Research (RISKESDAS), the national prevalence of these issues was 23.4% in 2007 and increased to 25.9% in 2013 (1). This indicates a persistently high prevalence of caries, which can elevate the risk of pulp disease. The prevalence of pulp disease in Indonesia is classified as high, as indicated by the 2011 Indonesia Health Profile, which ranked pulp and periapical disease as the 7th most common outpatient condition in 2010 (2).
Pulp necrosis, or dead pulp, can occur due to bacterial infections, trauma, or exposure to chemical irritants. It is important to note that root canal infections and pulp diseases are often caused by multiple types of bacteria (3). Among these bacteria, facultative anaerobes are commonly found in pulp and root canal infections (4). In necrotic root canals, facultative anaerobic bacteria account for 57.14%, while aerobic bacteria comprise 42.86% (5). Streptococcus viridans, a gram-positive bacterium, is a predominant and pathogenic bacteria species associated with root canal infections in the oral cavity (6). This bacterium, which constitutes approximately 63% of the facultative anaerobic bacteria inhabiting the root canals, is known to cause various oral infectious diseases such as caries, periodontitis, dental abscess, bacteremia, and endocarditis (7, 8). Consequently, bacterial infections leading to pulp necrosis indicate the need for root canal treatment.
Root canal treatment involves the removal of non-vital or necrotic pulp from the root canal, followed by its replacement with a suitable filling material (9). Successful root canal treatment relies on effectively eliminating bacteria and their organic substrates, which can be achieved by employing antibacterial root canal filling materials (10). During the preparation phase, a root canal irrigant removes necrotic tissue and dentin debris while moistening the tooth's root canal to facilitate the preparation process. This step helps reduce the number of microorganisms in the root canal, enabling the remaining bacteria to be targeted with antibacterial agents (10, 11).
Root canal irrigation plays a pivotal role in supporting the success of root canal treatment (11). An ideal root canal irrigant should possess potent antibacterial properties with minimal toxicity (12). Furthermore, it should disinfect and penetrate dentin tubules, lubricate endodontic devices, provide long-term antibacterial effects, remove the smear layer, dissolve necrotic tissue, and be cost-effective, non-toxic, and non-carcinogenic (13). Although various irrigants are available today, they still exhibit certain limitations. For instance, when used at high concentrations, sodium hypochlorite (NaOCl), a commonly used synthetic irrigant, can induce periapical inflammation. In contrast, low concentrations may be ineffective in completely eradicating bacteria or removing the smear layer (14). Therefore, exploring natural alternatives with maximum antibacterial efficacy and minimal toxicity for root canal irrigants is imperative.
Allium, a prominent genus within the Amaryllidaceae family, comprises over 780 species, predominantly perennial plants with underground storage organs (15). Notable species within this genus include onions, garlic, leeks, and shallots (16). Allium atroviolaceum, commonly known as Persian shallot or dark purple onion, is a flowering plant species belonging to the Alliaceae family. Indigenous to Iran and neighboring Middle Eastern countries, Allium atroviolaceum, has been the subject of numerous studies highlighting its antibacterial properties. Essential oil derived from Allium atroviolaceum has been tested against various bacterial strains, including Escherichia coli, Staphylococcus aureus, and Pseudomonas aeruginosa.
2. Objectives
This study aims to investigate the antibacterial effects of hydroalcoholic extracts from Allium atroviolaceum on oral bacteria of the Streptococcus viridans groups. Furthermore, the antibacterial effectiveness of this extract will be compared with that of chlorhexidine, a commonly used compound in dental applications.
3. Methods
3.1. Extraction
This study utilized the ethanolic extract of Allium atroviolaceum, commonly known as the tassel plant, due to its potent antimicrobial effects. The bulbs of the tassel plant were obtained from the mountains of Gavazang, located at the northern heights of Zanjan (Herbarium code: P1008773). These bulbs were carefully collected and assigned a specific code before being stored in the herbarium of the Faculty of Pharmacy at Zanjan University of Medical Sciences (Figure 1). To prepare the extract, the collected onions were thoroughly cleaned and dried in the shade at room temperature. Once dried, the underground pods were crushed and ground. The extraction process was carried out using the percolation method with 70% ethanol as the solvent. The percolation method was employed as follows: Initially, 100 gr of tassel plant bulbs were placed inside the percolator. Subsequently, 750 mL of a 70% ethanol solution was added to the percolator. The device was then securely positioned within a closed chamber under a hood. After 72 h, the resulting solution was drained into a Petri dish. For the second extraction step, 675 mL of 70% ethanol solution was added to the percolator, followed by another 72 hours for extraction. The resulting extract from the Petri dish was drained once again.
Allium atroviolaceum, as A, tassel plant and; B, bulbs plant. C & D, Allium atroviolaceum extraction via percolation method.
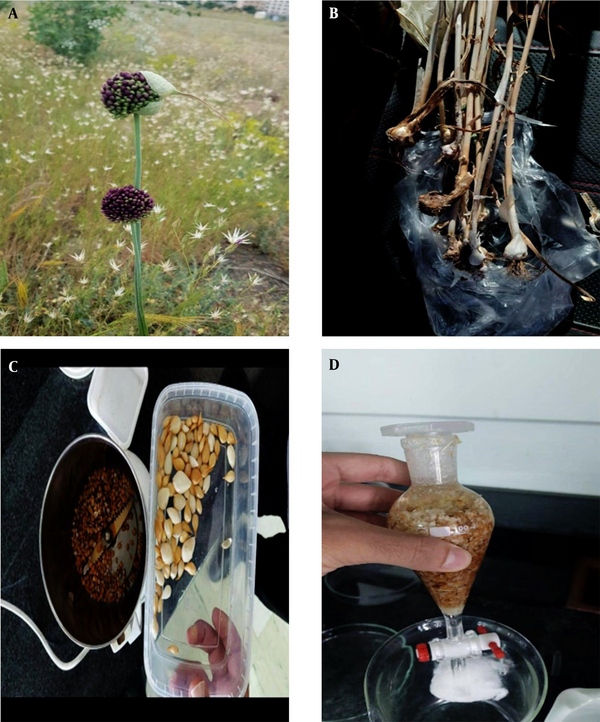
Lastly, for the third and final extraction step, 550 mL of 70% ethanol solution was added to the percolator, and after 72 h, the extract in the Petri dish was drained for the last time. This completed the extraction process. The subsequent removal of the solvent was accomplished using a rotary evaporator. The resulting solvent-free extract was stored in a dark, sealed container at 4°C until experimentation (17, 18).
3.2. McFarland Standard Preparation
To prepare the McFarland standard solution, 0.5 mL of a 1.75% barium chloride solution was added to 99.5 mL of 1% sulfuric acid. This solution can be stored in a dark environment for up to 6 months. The McFarland standard solution serves as a reference for determining the concentration of bacterial suspensions. The turbidity of the McFarland standard solution corresponds to a bacterial concentration of approximately 1.5 × 108 cfu/mL. Fresh and pure bacterial colonies are inoculated into a tube containing sterile physiological serum to create a bacterial suspension equivalent to the McFarland standard. By comparing the turbidity of the resulting bacterial suspension to that of the McFarland half standard solution, a suspension containing approximately 1.5 x 108 cfu/mL of bacteria can be prepared.
3.3. Streptococcus viridans Preparation
Lyophilized forms of standard Streptococcus strains, including Streptococcus mitis (ATCC 6249), Streptococcus mutans (PTCC 16836), Streptococcus sanguinis (PTCC 1449), and Streptococcus salivarius (PTCC 1448), were obtained from the Iran Scientific and Industrial Research Organization. To initiate the bacterial preparation process, these strains were cultured on Brain Heart Infusion (BHI) blood agar medium and subsequently incubated for 24 hours at 37°C under microaerophilic conditions. After incubation, the bacterial culture was adjusted to a 0.5 McFarland standard, corresponding to a bacterial concentration of approximately 1.5 × 108 cfu/mL. The turbidity of the bacterial culture was visually compared to that of the McFarland standard by holding both samples side by side against a white background featuring black horizontal lines. This ensured a consistent matching of turbidity between the culture and the standard, facilitating accurate standardization of the bacterial suspension.
3.4. An Antimicrobial Assay by Well Diffusion Method
The antimicrobial activity of the hydroalcoholic extract of Allium atroviolaceum and chlorhexidine was assessed using the well-diffusion qualitative method. BHI blood agar culture medium was employed to evaluate the antimicrobial effects. To initiate the assay, a bacterial suspension equivalent to half of the McFarland standard was prepared from the strains of Streptococcus mitis, Streptococcus mutans, Streptococcus sanguinis, and Streptococcus salivarius. Using a sterile swab, the bacterial suspension was uniformly inoculated onto the surface of the BHI blood agar medium, ensuring even distribution. Subsequently, the plates were incubated under microaerophilic conditions at 37°C, allowing the bacterial culture to grow. Next, wells were carefully created on the agar plate using a sterile Pasteur pipette. Within these wells, 50 microliters of the hydroalcoholic extract of Allium atroviolaceum, with a concentration of 50 mg/mL, PBS (Phosphate Buffer saline used as negative control), and 0.2% chlorhexidine (used as a positive control) were added. This facilitated the diffusion of the respective antimicrobial substances into the surrounding agar. After adding the extracts and control, the plates were incubated for 24 hours at 37°C, maintaining the microaerophilic conditions. After incubation, the diameter of the non-growth zone around each well (clear zone where bacterial growth was inhibited) was measured using a ruler or caliper, providing a quantitative assessment of the antimicrobial activity.
By measuring the diameter of the non-growth zone, the inhibitory effect of the hydroalcoholic extract of Allium atroviolaceum and chlorhexidine against the tested bacterial strains can be evaluated.
3.5. An antimicrobial Assay by Minimum Inhibitory Concentration and Minimum Bactericidal Concentration
The minimum inhibitory concentration (MIC) of the hydroalcoholic extract of Allium atroviolaceum and chlorhexidine was determined using the micro-broth dilution quantitative method. A 96-well microplate was utilized for this purpose. Initially, 150 μL of BHI broth culture medium was added to all plate wells. Starting with the first well, 150 μL of the hydroalcoholic extract of Allium atroviolaceum with a concentration of 50 mg/mL was added. This resulted in a 25 mg/mL concentration in the first well. The extract and culture medium were thoroughly mixed by gently pipetting up and down. Then 150 μL of this mixture was transferred to the next well (well No. 2). The process was repeated for subsequent wells, with 150 μL of the mixture being transferred and diluted from one well to the next, following the same up-and-down mixing technique. This serial dilution continued until well No. 9, with 150 μL being discarded from this well, maintaining a final volume of 150 μL in all wells. Two control wells were included in the plate. No. 11 served as the negative control, containing only culture medium without extract or bacteria, ensuring contamination-free conditions. No. 12 was the positive control, containing culture medium and bacteria without adding the extract. After diluting the extract, 15 μL of bacterial suspension equivalent to 1.5 × 106 cfu/mL was added to each well. The microplate was then incubated at 37°C under microaerophilic conditions for 18 - 24 hours. The lowest dilution of the hydroalcoholic extract that did not exhibit visual turbidity was considered the minimum inhibitory concentration (MIC), indicating the concentration at which bacterial growth was inhibited.
To determine the minimum bactericidal concentration (MBC) of the hydroalcoholic extract of Allium atroviolaceum, wells without bacterial growth (no turbidity) were selected. From these wells, 50 μL of the contents were cultured on a BHI blood agar medium. The agar plates were subsequently incubated at 37°C for 18 - 24 hours. After incubation, the plates were examined for bacterial growth, and the agar plate with the lowest extract concentration that completely inhibited bacterial growth was considered the minimum lethal concentration or MBC. Similarly, the minimum inhibitory and lethal concentrations for 0.2% chlorhexidine were also determined following the same procedure.
3.6. Data Analysis
The data obtained from the research, in the form of colony counts, was subjected to statistical analysis. A one-way analysis of variance (ANOVA) test was conducted to determine if there were any significant differences among the sample groups. Additionally, a least significant difference (LSD) post hoc test was performed to identify specific significant differences between the groups. These tests were employed to analyze and interpret the data, providing valuable insights into the comparative outcomes of the different sample groups.
4. Results
4.1. Well Diffusion Method
The present study aimed to evaluate the antimicrobial effects of the hydroalcoholic extract of Allium atroviolaceum and 0.2% chlorhexidine mouthwash on standard strains of Streptococcus mitis (ATCC 6249), Streptococcus mutans (PTCC 16836), Streptococcus sanguinis (PTCC 1449), and Streptococcus salivarius (PTCC 1448) under laboratory conditions. Using the well diffusion method, the diameter of the growth inhibition zone was measured for the hydroalcoholic extract of Allium atroviolaceum at a concentration of 50 mg/mL against the four Streptococcus viridans group bacteria. The exact measurement was performed for chlorhexidine as a positive control. The results are presented in Figure 2A and B, showing the values of the growth inhibition zone in millimeters. As illustrated in Figure 2A, the hydroalcoholic extract of Allium atroviolaceum at a concentration of 50 mg/mL exhibited the highest inhibitory effect against Streptococcus salivarius, with a growth inhibition zone diameter of 17.00 ± 2.00 mm. On the other hand, it showed the lowest inhibitory effect against Streptococcus mutans, with a growth inhibition zone diameter of approximately 12.00 ± 1.00 mm. The diameter of the growth inhibition zone for chlorhexidine against the tested streptococci ranged between 19.00 ± 0.50 and 22.00 ± 1.00 mm. These results provide valuable insights into the antimicrobial activity of the hydroalcoholic extract of Allium atroviolaceum and its potential as an alternative to chlorhexidine for combating oral bacterial infections.
A, Inhibition zone diameter induced by Allium atroviolaceum extract, chlorhexidine, and PBS (phosphate buffer saline) on all investigated microorganisms. B, Inhibition Zone via well diffusion assay.

4.2. Minimum Inhibitory Concentration and Minimum Bactericidal Concentration
In this study, the antimicrobial activity of the hydroalcoholic extract of Allium atroviolaceum was also evaluated using the MIC (minimum inhibitory concentration) and MBC (minimum bactericidal concentration) methods against Streptococcus mitis, Streptococcus mutans, Streptococcus sanguinis, and Streptococcus salivarius. The results were compared with 0.2% chlorhexidine, a widely used antimicrobial agent. Table 1 presents the MIC and MBC values of the hydroalcoholic extract of Allium atroviolaceum and chlorhexidine against the tested streptococci. Serial dilutions of the extract and chlorhexidine were performed, starting with concentrations of 25 mg/mL and 0.1%, respectively. MIC and MBC values of the extract for Streptococcus sanguinis were found to be approximately 3.12 mg/mL, whereas the MIC and MBC values for other strains (Streptococcus mitis, Streptococcus mutans, Streptococcus sanguinis, and Streptococcus salivarius) were about 6.25 mg/mL. Comparatively, chlorhexidine exhibited lower MIC and MBC values of 0.0003%. These findings indicate that the hydroalcoholic extract of Allium atroviolaceum possesses moderate antimicrobial activity against the tested streptococci, with comparable effectiveness to chlorhexidine. Figure 3A displays the image of the 96-well microplate used to determine the MIC of the tassel onion extract. The absence of visible growth in certain wells indicates the inhibitory effect of the extract at specific concentrations. Figure 3B illustrates the plates containing BHI blood agar used to determine the MBC of the extract. The absence of bacterial growth on the plates indicates the extract's lethal effect at specific concentrations.
MIC and MBC Values for Allium atroviolaceum Extract, and Chlorhexidine Against all Investigated Microorganisms
Microorganisms | Allium atroviolaceum (mg/mL) | Chlorhexidine (0.2%) | ||
---|---|---|---|---|
MBC | MIC | MIC (%) | MBC (%) | |
Streptococcus mitis | 6.25 | 6.25 | 0.0003 | 0.0003 |
Streptococcus mutans | 6.25 | 6.25 | 0.0003 | 0.0003 |
Streptococcus sanguinis | 3.12 | 3.12 | 0.0003 | 0.0003 |
Streptococcus salivarius | 6.25 | 6.25 | 0.0003 | 0.0003 |
A, 96-well microplate to determine the MIC of the Allium atroviolaceum extraction and chlorhexidine against all investigated microorganisms; B, Blood agar plates to MBC microorganism’s assay.
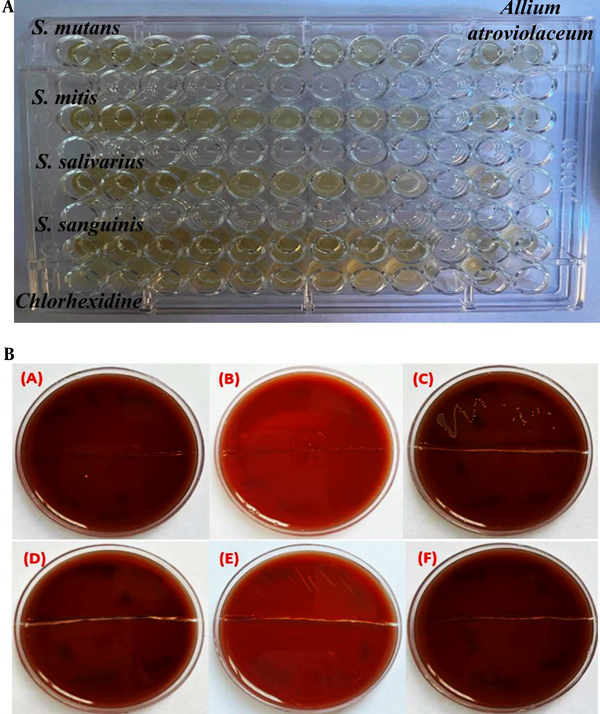
5. Discussion
The results of the antibacterial assay using the well diffusion, MIC, and MBC methods were obtained for the hydroalcoholic extract of Allium atroviolaceum and 0.2% chlorhexidine against Streptococcus sanguinis, Streptococcus mitis, Streptococcus mutans, Streptococcus sanguinis, and Streptococcus salivarius. To better understand the significance of these findings, a comparison with existing literature is necessary. The well diffusion method demonstrated the inhibitory effect of the hydroalcoholic extract of tassel onion on the growth of the tested streptococci. The diameter of the growth inhibition halo was observed, with the extract showing varying degrees of effectiveness against different strains. The results demonstrated in Figure 1 indicates that the extract exhibited the highest inhibitory effect against Streptococcus salivarius, with a diameter of approximately 17.00 ± 2.00 mm. In contrast, the lowest inhibitory effect was observed against Streptococcus mutans, with a diameter of approximately 12.00 ± 1.00 mm. Chlorhexidine, the positive control, displayed inhibition halos ranging from 19.00 ± 0.50 to 22.00 ± 1.00 mm, demonstrating its potent antimicrobial activity against the tested streptococci. In examining the well diffusion results, varying inhibitory effectiveness against different streptococcal strains suggests a nuanced interaction between the extract and specific bacterial species. Notably, the extract's highest inhibitory effect against Streptococcus salivariusaligns with previous research on Allium species, hinting at strain-specific responses.
In the MIC and MBC analysis, the hydroalcoholic extract of Allium atroviolaceum and chlorhexidine were subjected to serial dilution to determine the lowest concentration at which bacterial growth was inhibited (MIC) and the lowest concentration that resulted in bacterial death (MBC). In comparison, chlorhexidine displayed significantly lower MIC and MBC values of 0.0003%, indicating its greater potency in inhibiting bacterial growth and causing bacterial death. When comparing these results with the existing literature, it is essential to consider variations in extraction methods, sample sources, and bacterial strains used in different studies. However, several studies have investigated the antimicrobial activity of Allium species, including tassel onion, against various bacterial pathogens. For instance, research conducted by Lahiri D et al. reported the antimicrobial potential of Allium cepa (onion) extract against Streptococcus mutans and Streptococcus sanguinis, corroborating our findings (19). Additionally, studies on the antimicrobial activity of chlorhexidine have consistently highlighted its effectiveness against a wide range of bacteria, including streptococci (20, 21). Although the antimicrobial activity of the hydroalcoholic extract of Allium atroviolaceum was less potent than chlorhexidine, it still exhibited significant inhibitory effects. This suggests that the extract could serve as a potential alternative or complementary treatment option, particularly in cases where synthetic antimicrobial agents may not be suitable or desired. However, a more detailed exploration of known bioactive compounds within Allium atroviolaceum could be integrated to bolster the discussion. For instance, Allium species contain organosulfur compounds, flavonoids, and polyphenols, each with antimicrobial properties. Investigating the interplay of these compounds with the bacterial strains tested could provide a more nuanced understanding of the extract's inhibitory potential. While acknowledging the limitations of the present study in identifying specific bioactive compounds, it is crucial to highlight the potential implications of such identification. Future research endeavors could focus on isolating and characterizing these compounds, unraveling the molecular mechanisms underpinning the extract's antimicrobial activity. This, in turn, may contribute to developing targeted and potent antimicrobial agents derived from Allium atroviolaceum. In summary, augmenting the discussion with a more thorough exploration of the chemical constituents of Allium atroviolaceum and their implications for antimicrobial activity would enrich the understanding of the extract's therapeutic potential.
5.1. Conclusion
In this investigation, we probed the antimicrobial prowess of the hydroalcoholic extract of Allium atroviolaceum alongside the widely used 0.2% chlorhexidine, targeting Streptococcus sanguinis, Streptococcus mutans, Streptococcus salivarius, and Streptococcus mitis through comprehensive well diffusion, MIC, and MBC assessments. Our findings distinctly showcase the inhibitory impact of Allium atroviolaceum on the tested streptococcal strains, manifesting as discernible growth inhibition zones in the well diffusion assay. Notably, the extract exerted its most potent inhibitory effect against Streptococcus salivarius, underscoring its strain-specific efficacy, albeit with comparatively lower effectiveness against Streptococcus mutans. Delving deeper through MIC and MBC analyses, the hydroalcoholic extract revealed MIC and MBC values of 3.12 mg/mL against Streptococcus sanguinis and 6.25 mg/mL against the remaining strains. While these values position Allium atroviolaceum as a viable antimicrobial contender, chlorhexidine emerged as a more potent adversary, displaying significantly lower MIC and MBC values of 0.0003%. This emphasizes chlorhexidine's robust capability in inhibiting bacterial growth and inducing bacterial death. In a broader context, our study adds substantial weight to the expanding body of evidence affirming the antimicrobial properties of Allium atroviolaceum. However, we recognize the imperative for continued exploration. Unraveling the specific bioactive compounds responsible for its antimicrobial activity is a priority. Future investigations should pivot toward isolating and characterizing these compounds, shedding light on their mechanisms of action, safety profiles, and plausible clinical applications.
Our work signals a critical juncture in understanding the antimicrobial potential of Allium atroviolaceum extract, illuminating promising avenues for the development of natural antimicrobial agents. This research transcends the laboratory, envisioning novel therapeutic alternatives for combatting bacterial infections. As we navigate the evolving landscape of antimicrobial research, Allium atroviolaceum emerges as a beacon of possibility, especially where synthetic antimicrobial agents may prove less suitable or preferred.
References
-
1.
Sudikno S, Syarief H, Dwiriani CM, Riyadi H. Faktor Risiko Obesitas Sentral Pada Orang Dewasa Umur 25-65 Tahun Di Indonesia (Analisis Data Riset Kesehatan Dasar 2013). Penelitian Gizi dan Makanan (The Journal of Nutrition and Food Research). 2016;38(2). https://doi.org/10.22435/pgm.v38i2.5540.111-120.
-
2.
Indonesia KR. Profil data kesehatan Indonesia tahun 2011. Jakarta: Kementerian Kesehatan Republik Indonesia; 2012.
-
3.
Walton RE, Torabinejad M, Bakland LK. Endodontics. In: 10th, editor. Principles and Practice. Louis, Missouri: Elsevier; 2009.
-
4.
Sakko M, Tjaderhane L, Rautemaa-Richardson R. Microbiology of Root Canal Infections. Prim Dent J. 2016;5(2):84-9. [PubMed ID: 28826437]. https://doi.org/10.1308/205016816819304231.
-
5.
Yamin IF, Natsir N. Bakteri dominan di dalamsaluran akar gigi nekrosis (Dominant bacteria in root canal of necrotic teeth). Journal of Dentomaxillofacial Science. 2014;13(2). https://doi.org/10.15562/jdmfs.v13i2.399.
-
6.
de Lima Guimarães NLS, Otoch HM, de Andrade LC, Ferreira CM, Rocha MMDNP, de Almeida Gomes F. Microbiological evaluation of infected root canals and their correlation with pain. RSBO Revista Sul-Brasileira de Odontologia. 2012;9(1):31-7.
-
7.
Pujiastuti P, Lestari S. Perbedaan Efektifitas Antibakteri Ekstrak Daun Sirih Merah (Piper crocatum) pada Porphyromonas gingivalis dan Streptococcus viridans. STOMATOGNATIC-Jurnal Kedokteran Gigi. 2015;12(1):1-4.
-
8.
Samaranayake L. 4th, editor. Essential microbiology for dentistry. 2012. Churchil Livingstone, Elsevier; 2012.
-
9.
Mielczarek A, Król K. Conservative dentistry and endodontics. Oxford University Press; 2020, [cited 2023]. Available from: https://wls.wum.edu.pl/sites/wls.wum.edu.pl/files/Sylabusy%20EDD%2022-23/conserv-dent-and-endo-5-rok-edd-2022-2023_2.pdf.
-
10.
Nugroho JJ, Trilaksana AC, Natsir N, Rovani CA, Asrianti A, Hikmah N. Cytotoxicity of 5% ethanol extracts of moringa oleifera leaf as an alternative of root canal irrigant to fibroblast BHK-21 cell culture. Journal of Dentomaxillofacial Science. 2021;6(1):39-41. https://doi.org/10.15562/jdmfs.v6i1.1105.
-
11.
Wahjuningrum DA, Pramesti HD, Sihombing MR, Devi ZL, Roelianto M. Chitosan Antibacterial Activity Against Streptococcus viridans. Malaysian Journal of Medicine and Health Sciences. 2021.
-
12.
Budi HS, Juliastuti WS, Christy BR. Antimicrobial Activity of Musa paradisiaca var. sapientum on Enterococcus faecalis Viability. Malays J Med Health Sci. 2020;16(Supp 4):17-21.
-
13.
Ramachandran RA, Li W, Khande S, Shylendra A, Trivedi AR, Xie Q, et al. Advanced Electrochemical Reamer (EC-Reamer) for Root Canal Treatment. Eur Endod J. 2023;8(1):79-89. https://doi.org/10.14744/eej.2022.86094.
-
14.
Kotecha N, Shah NC, Doshi RJ, Kishan KV, Luke AM, Shetty KP, et al. Microbiological Effectiveness of Sodium Hypochlorite Gel and Aqueous Solution When Implemented for Root Canal Disinfection in Multirooted Teeth: A Randomized Clinical Study. J Funct Biomater. 2023;14(5). [PubMed ID: 37233350]. [PubMed Central ID: PMC10219193]. https://doi.org/10.3390/jfb14050240.
-
15.
Lorigooini Z, Kobarfard F, Ayatollahi SA. Anti-platelet aggregation assay and chemical composition of essential oil from Allium atroviolaceum Boiss growing in Iran. Int J Biosci. 2014;5(2):151-6.
-
16.
Lanzotti V, Barile E, Antignani V, Bonanomi G, Scala F. Antifungal saponins from bulbs of garlic, Allium sativum L. var. Voghiera. Phytochemistry. 2012;78:126-34. [PubMed ID: 22513009]. https://doi.org/10.1016/j.phytochem.2012.03.009.
-
17.
Abou-Hashem MMM, Abo-Elmatty DM, Mesbah NM, Abd El-Mawgoud AM. Induction of sub-G(0) arrest and apoptosis by seed extract of Moringa peregrina (Forssk.) Fiori in cervical and prostate cancer cell lines. J Integr Med. 2019;17(6):410-22. [PubMed ID: 31669164]. https://doi.org/10.1016/j.joim.2019.09.004.
-
18.
Gharbavi M, Mousavi M, Pour-Karim M, Tavakolizadeh M, Sharafi A. Biogenic and facile synthesis of selenium nanoparticles using Vaccinium arctostaphylos L. fruit extract and anticancer activity against in vitro model of breast cancer. Cell Biol Int. 2022;46(10):1612-24. [PubMed ID: 35819083]. https://doi.org/10.1002/cbin.11852.
-
19.
Lahiri D, Nag M, Dutta B, Dash S, Ghosh S, Ray RR. Synergistic Effect of Quercetin with Allicin from the Ethanolic Extract of Allium cepa as a Potent AntiQuorum Sensing and Anti-Biofilm Agent Against Oral Biofilm. Advances in Bioprocess Engineering and Technology. Springer; 2021. p. 69-81. https://doi.org/10.1007/978-981-15-7409-2_7.
-
20.
O'Connor M, Harrison G, Lenahan D, Moran GP. A dentifrice containing salivary enzymes and xylitol exhibits superior antimicrobial activity in vitro against adherent Streptococcus mutans compared to a chlorhexidine dentifrice. Lett Appl Microbiol. 2023;76(2). [PubMed ID: 36764670]. https://doi.org/10.1093/lambio/ovad026.
-
21.
Pillai SGP, Johnson L, Bagde H. Chlorhexidine Mouthwash in Dentistry. Journal of Nursing Research,Patient Safety and Practise. 2023;3(3):24-9. https://doi.org/10.55529/jnrpsp.33.24.29.