Abstract
Background:
Alzheimer's disease (AD) represents the most prevalent form of dementia, characterized by memory loss, and is a complex, progressive neurodegenerative condition.Objectives:
This study aimed to explore the impact of aerobic interval training (AIT) combined with Ginkgo biloba (GB) supplementation on passive avoidance memory function and the expression of the glial cell-derived neurotrophic factor (GDNF) gene in rats afflicted with AD.Methods:
A total of forty-four male Wistar rats, each weighing approximately 250 ± 20 g, were maintained in a controlled environment at a specified temperature. Over 8 weeks (5 days per week), they underwent AIT and received GB supplements at a dosage of 100 mg/kg. The rats were randomly assigned into six groups: Healthy control (HC) with 7 rats, AD control (AC) with 8 rats, amyloid-β (Aβ) injection plus GB supplement (Aβ + GB) with 8 rats, Aβ injection plus GB supplement plus AIT (Aβ + GB + AIT) with 8 rats, Aβ injection plus AIT (Aβ + AIT) with 8 rats, and sham surgery (SS) with 5 rats. The shuttlebox apparatus was utilized to assess the effect of Aβ on passive avoidance memory and to confirm disease induction. GDNF gene expression was measured using the qRT-PCR method.Results:
The study found significant improvements in passive avoidance memory in the Aβ + AIT and Aβ + GB + AIT groups compared to the AC group after 8 weeks of AIT and GB supplementation (P = 0.02 and P = 0.000, respectively). Long-term memory performance was notably enhanced in the Aβ + GB + AIT group (P = 0.000). Additionally, GDNF expression significantly increased in the Aβ + GB + AIT and Aβ + GB groups in comparison to the AC group (P = 0.000 and P = 0.01, respectively).Conclusions:
A synergistic effect of AIT and GB supplementation was observed, leading to an increase in GDNF gene expression and improvements in both passive avoidance memory and long-term memory in male rats with AD.Keywords
Aerobic Interval Training Ginkgo biloba Passive Avoidance Memory GDNF Alzheimer’s Disease, Rats
1. Background
Alzheimer's disease (AD), the predominant form of dementia, manifests through symptoms like memory loss, problem-solving challenges, and spatial and temporal disorientation. The development of AD is attributed to various factors, including the accumulation of the Aβ peptide, disturbances in glutamatergic neurotransmission, the cholinergic hypothesis, alterations in tau protein, and the impact of oxidative stress and calcium imbalance (1). Glial cell-derived neurotrophic factor (GDNF), a neurotrophic factor most commonly found in the human brain, is mainly produced by glial cells such as microglia, astrocytes, and neurons (2). A reduction in GDNF levels has been linked to the emergence of AD symptoms and pathologies, indicating its potential as a therapeutic target for neurodegenerative diseases. Furthermore, dopaminergic neurons in the substantia nigra, which generate GDNF, play a protective role for various neuronal types, including those dopaminergic neurons themselves (3). The degeneration of dopaminergic neurons is intricately connected to AD, with treatment strategies targeting the dopaminergic system and synaptic dysfunction to improve memory issues in patients (4). Remarkably, the enhanced expression of GDNF in genetically modified mice (Gdnfwt/hyper) has been shown to counteract the progressive decline in cholinergic transmission, a hallmark of dementia (5). In contemporary times, physical activity extends beyond leisure and is recognized as essential for overall health and cognitive function (6). Aerobic interval training (AIT) has demonstrated potential in altering the formation, breakdown, and clearance of AD's characteristic pathologies, such as Aβ and hyperphosphorylated tau, in animal studies (7). Although there are drugs available to mitigate AD complications, their efficacy is often limited, and they may entail side effects. Hence, the exploration of medicinal herbs for their neuroprotective properties, especially in blocking acetylcholinesterase (AChE) or reducing oxidative stress, has gained traction among researchers (8).
Ginkgo biloba (GB), an herb traditionally used to treat high blood pressure, ischemic myocardium, and cerebral ischemia (9), is derived from ancient Chinese medicinal practices known for their therapeutic advantages in managing AD (10). Notably, extracts from GB leaves have been identified as protective against neuronal damage in animal models (11). A study by Shahidi et al. assessed the impact of vitamin E and GB on the electrophysiology in a rat model of AD, revealing that GB augmented the activity of endogenous antioxidant enzymes and AChE in rats (12). Furthermore, research focusing on depressed elderly men examined the effects of combining exercise with GB supplementation on brain-derived neurotrophic factor (BDNF) levels. Findings indicated a significant elevation in BDNF levels, suggesting that exercise paired with GB intake could enhance brain function in older adults (13).
2. Objectives
The aim of this study was to explore the impact of eight weeks of AIT and GB intake on passive avoidance memory and the expression of GDNF in rats with AD.
3. Methods
3.1. Animals
Forty-four male Wistar rats, each weighing approximately 250 ± 20 g, were accommodated in standard transparent cages, with two rats per cage, in an environment maintained at 22 ± 2°C. They were subjected to a 12-hour light-dark cycle and had free access to standard food and tap water.
3.2. Study Design
The 44 rats were randomly allocated into six groups: HC (7 rats), AC (8 rats), Aβ + GB (8 rats), Aβ + GB + AIT (8 rats), Aβ + AIT (8 rats), and SS (5 rats).
A total of 48 male Wistar rats were acquired from the Animal Research Center at Zahedan University of Medical Sciences and were distributed into six groups of eight. During the course of the study protocol and disease induction, three rats died, and one sample was removed from the study during the tissue isolation and gene expression phase. The HC group did not receive any treatment.
The sham surgery, involving sterile saline injection, was conducted to assess the impact of surgical stress.
There were two exercise groups: AIT was carried out on a treadmill five days a week for eight weeks.
Two groups were designated as supplement consumption groups: They were administered GB extract (100 mg/kg/day) five days a week for eight weeks.
3.3. Surgery and Injection of Aβ
After acquisition and an initial weighing, the rats were housed in a cage for two weeks to acclimate to their new environment. The surgical procedure for the AD groups was then executed over four consecutive days. To initiate the disease, sterile saline was mixed with 5 μg/μL of Aβ peptide (Aβ1-42, GenScript Company, America) and incubated at 37°C for one week to encourage fibrillation and peptide aggregation. Sterile saline was added to compensate for any volume loss, and the solution was subsequently stored at -20°C until needed (14).
For the AD model induction, rats were anesthetized with intraperitoneal injections of ketamine (100 mg/kg) and xylazine (8 mg/kg) and secured in a stereotaxic apparatus. Sterile scissors were used to make a vertical incision along the median longitudinal calvaria on the head skin. The bregma was located, and two 1 mm diameter holes were drilled about 2 mm posterior (P) and 1.4 mm lateral (L) to the anterodorsal thalamic nucleus for the Aβ (1-42) peptide injection. The skull surface was meticulously cleaned with sterile dry cotton between steps (15).
The injection of 2.0 μL of Aβ1-42 peptide into each lateral ventricle was performed using a 5 μL microsyringe (Hamilton Laboratory Products, Reno, NV, USA) over 5 minutes (15). Four weeks post-surgery, the shuttle test was carried out to confirm the induction of AD (16). Subsequently, the treatments, including an exercise protocol and GB supplement consumption, commenced for eight weeks.
The shuttle box device was utilized to assess the impact of amyloid on disease induction and to evaluate passive avoidance memory.
3.4. Exercise Protocol
The AIT groups engaged in treadmill running five days a week for eight weeks. For the initial two weeks, the animals underwent two 15-minute running stages at a speed of 10 m/s. During the third and fourth weeks, the duration increased to three and four stages, respectively, at a speed of 12 m/min. The fifth and sixth weeks involved four stages of running at 14 m/min, and in the final two weeks, the animals ran four stages at 16 m/min. Each session included a five-minute warm-up and cool-down at a speed of 5 m/min (Table 1) (14).
Exercise Program for Exercise Groups
Training (Five Days a Week) | A Familiarization Stage (Two Weeks Before Starting the Training Protocol) | Week | |||||||
---|---|---|---|---|---|---|---|---|---|
1 | 2 | 3 | 4 | 5 | 6 | 7 | 8 | ||
Time in sets, min | 15 | 15 | 15 | 15 | 15 | 15 | 15 | 15 | 15 |
Number of stages per day | 1 | 2 | 2 | 3 | 4 | 4 | 4 | 4 | 4 |
Active rest 5 minutes | - | 1 | 1 | 2 | 3 | 3 | 3 | 3 | 3 |
Training speed, m/m | 5 - 8 | 10 | 10 | 12 | 12 | 14 | 14 | 16 | 16 |
VO2max, % | 40 | 45 | 45 | 49 | 49 | 53 | 53 | 55 | 55 |
3.5. Preparation and Administration of GB
Following the confirmation of disease induction, GB supplementation began for the designated groups, in parallel with the start of the exercise protocol. This regimen was maintained five days a week for eight weeks.
In the Aβ + GB + AIT group, ginkgo consumption took place immediately after each exercise session. This study utilized a 6% solution of powdered and dried GB leaves (code: 1400-H079-369) sourced from Dineh, Tehran, administered at a dosage of 100 mg/kg. The vehicle for the plant powder was composed of distilled water, sorbitol, and 99% alcohol, in the proportions of 80:5:15, respectively. For the preparation of the solution, the leaves were pulverized, and 2 grams of GB powder were mixed with 5 ml of alcohol and 1.6 ml of sorbitol. Distilled water was then added to achieve a total solution volume of 26.6 ml. This solution was prepared eight times over the course of the training period and was kept refrigerated at 4°C. The solution was administered to the rats via gavage daily between 9 and 11 in the morning. The dosage was determined by multiplying the weight of each rat by 0.017, ensuring each rat received 100 mg per kg of body weight. The weights of the rats were recorded on a weekly basis (17).
3.6. The Shuttle Box
The shuttle box apparatus is divided into two sections: A lighted compartment and a dark compartment, separated by a guillotine door at the bottom. The dark compartment features steel rods on its floor capable of delivering an electric shock of adjustable intensity and frequency. After acclimatizing to the laboratory environment for at least 30 minutes, the rats were initially placed in the lighted compartment. Five seconds later, the guillotine door was opened, enabling the rat to move to the dark compartment. The rat was considered to have entered the dark compartment once its hind legs crossed the threshold. Upon entry, the door was closed, and the rat was returned to its cage after 10 seconds. This procedure was repeated after a 30-minute interval. If the rat did not move into the dark compartment within 15 - 20 seconds, the test was concluded, and the rat was placed back in its cage. Two minutes later, the rat was placed in the lighted compartment again for the third attempt. If the rat failed to enter the dark compartment within two minutes, the test was stopped, and the rat was returned to its cage. Twenty-four hours later, a memory test was conducted. Each rat was placed in the lighted section, and after 20 seconds, the guillotine door was opened. The duration taken by the rat to enter the dark section, known as the STL, was recorded as an indicator of memory. If the rat exhibited memory by not entering the dark compartment, the test ended after 5 minutes (18). This test was performed initially before the injection, again four weeks post-injection, and after the completion of all treatment procedures.
3.7. Quantitative Real-time PCR
The quantitative real-time PCR (qRT-PCR) method was utilized to assess GDNF gene expression. Total RNA extraction from hippocampal tissue was conducted using an RNX-Plus™ kit (Cinnagen, Iran), following the manufacturer's instructions. The quality and concentration of RNA were verified using a NanoDrop 2000c (Eppendorf, Germany). RNA samples treated with DNase underwent cDNA synthesis with a cDNA synthesis kit (Pars Tos, Iran). PCR primers for amplifying GDNF (Gene ID: 25453) and ACTB (β-actin) (Gene ID: 81822) genes were designed using the Primer-BLAST tool on NCBI (National Center for Biotechnology Information) (Table 2). The qRT-PCR was conducted using Master Mix and SYBR® Green (Pars Tus, Iran) in a StepOne™ Applied Biosystems thermocycler. The thermocycler conditions were set to an initial hold at 95°C for 5 minutes, followed by 35 cycles of denaturation at 94°C for 20 seconds, annealing of primers at 60°C for 40 seconds, and extension at 72°C for 30 seconds. Data normalization was carried out using β-actin as the housekeeping gene. The efficiency of the GDNF and β-actin genes was determined using LinRegPCR software version 2013. x. The relative expression of the GDNF gene in hippocampal tissue was calculated using Pfaffl's method (14).
The qRT-PCR Primer Sequences
Gene | Primer |
---|---|
GDNF | |
Forward | CTGTTCTCCTCTCCTGGCTGTTC |
Reverse | CTCGGGGCTTTCCTCGTC |
β-actin | |
Forward | GATGGTGGGTATGGGTCAG |
Reverse | GCTCATTGTAGAAAGTGTGG |
3.8. Statistical Analysis
Data were presented as mean ± standard error of the mean (SEM). The scores from the passive avoidance test were analyzed using repeated measures analysis. Significance was established through one-way ANOVA with LSD’s post-hoc test (SPSS, version 23), considering a value of P < 0.05 as significant.
4. Results
4.1. Passive Avoidance Memory Results
The passive avoidance memory test revealed that the AC (Alzheimer's disease control) group exhibited a significantly shorter STL time compared to other groups, confirming the successful induction of AD in this group. Treatment with AIT and GB supplementation extended the STL duration in comparison to the AC group, thereby enhancing passive avoidance memory. In inter-group comparisons, a notable difference was observed between the Aβ + AIT group (186.5 ± 122.9) and the Aβ + GB + AIT group (300.0 ± 0.0), AC (74.4 ± 55.9), SS (300.0 ± 0.0), and HC (300.0 ± 0.0). Additionally, the Aβ + GB + AIT group significantly differed from the Aβ + GB (162.2 ± 120.6) and AC groups. The Aβ + GB group also differed significantly from the SS and HC groups. Beyond these comparisons, the AC group was significantly different from the SS and HC groups (P < 0.05) (Figure 1).
Between-group results of the passive avoidance test. The latency time (in seconds) to enter the dark chamber before receiving a foot shock was significantly higher in the Aβ + GB + AIT, SS, and HC groups compared to the AC group, indicating an improvement in step-through latency (STL). The level of significance was set at (P < 0.05). Beta-amyloid injection (Aβ), healthy control (HC), Alzheimer's disease control (AC), Ginkgo biloba (GB), aerobic interval training (AIT), and sham surgery (SS).
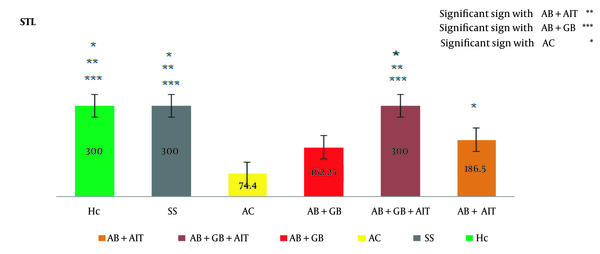
4.2. Results of the Passive Avoidance Test for Disease Induction and Long-term Memory Performance
The passive avoidance test results, aimed at assessing disease induction and long-term memory performance, indicated that prior to surgery, animals in all groups did not enter the dark section, showing a uniform STL of (300.0 ± 0.0). A comparison of STL times before and 4 weeks after surgery in the groups receiving Aβ injections highlighted the induction of AD and the impact of Aβ aggregation, with a noticeable difference indicating AD presence in these animals. The Aβ + AIT and Aβ + GB groups demonstrated a significant difference in STL before surgery and after 8 weeks (186.5 ± 122.9 and 162.2 ± 120.6, respectively), yet no difference was noted when comparing times 4 weeks (214.1 ± 99.1 and 220.3 ± 62.0, respectively) and 8 weeks post-surgery. In contrast, the Aβ + GB + AIT group showed consistent STL times before surgery and after 8 weeks (300.0 ± 0.0), with a notable difference observed between immediately post-surgery (225.8 ± 86.1) and 8 weeks later, suggesting that AIT combined with GB supplementation enhances long-term memory in rats with AD. The AC group's results indicated a progression in Aβ degradation and a significant decline in long-term memory function at 4 and 8 weeks post-surgery (217.8 ± 64.01 and 74.4 ± 55.9, respectively). Moreover, between the SS and HC groups, no significant differences were observed across the three test phases, implying that the sham surgery did not significantly impact long-term memory performance (P < 0.05) (Figure 2).
The passive avoidance test was conducted to assess the results within each group. The experiment consisted of three parts: before surgery, 4 weeks after surgery, and 8 weeks after surgery. Statistically significant differences were found between the pre-operative and post-operative stages in groups that received Aβ injections. In the AC group, differences were noticeable before and 8 weeks post-surgery. The Aβ + GB + AIT group exhibited significant improvements post-surgery and following AIT and GB supplementation. The significance level was set at (P < 0.05). beta-amyloid injection (Aβ), healthy control (HC), Alzheimer's disease control (AC), Ginkgo biloba (GB), aerobic interval training (AIT), and sham surgery (SS).
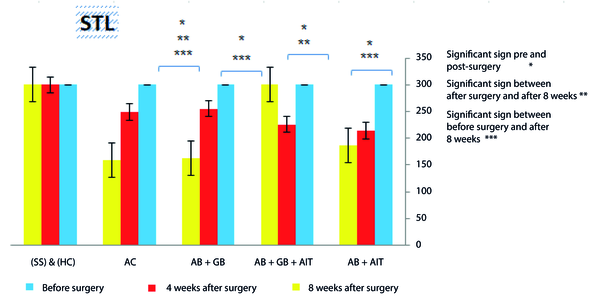
4.3. GDNF Gene Expression Analysis
The qRT-PCR method was employed to evaluate changes in the relative expression of the GDNF gene across the experimental groups. A notable difference in expression was observed between the Aβ + AIT group (0.83 ± 0.42) and both the Aβ + GB + AIT (1.93 ± 0.99) and Aβ + GB (1.74 ± 1.49) groups. When comparing the Aβ + GB + AIT group to other groups, a significant increase was noted in comparison to the AC (0.75 ± 0.16) and SS (0.98 ± 0.67) groups. Similarly, the Aβ+GB group's expression levels were significantly higher than those of the AC group. However, no significant difference in GDNF gene expression was detected between the HC (1.28 ± 0.18) group and the other groups (P < 0.05) (Figure 3).
The levels of relative GDNF gene expression. The Aβ + GB + AIT and Aβ + GB groups showed significantly higher expression levels than the AC group. The significance level was set at (P < 0.05). beta-amyloid injection (Aβ), health control (HC), Alzheimer's disease control (AC), Ginkgo biloba (GB), aerobic interval training (AIT), and sham surgery (SS).
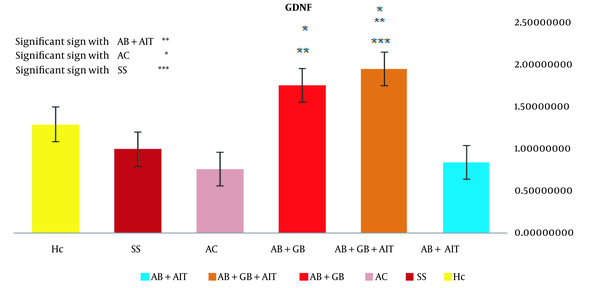
5. Discussion
The findings suggest that AIT mitigates the impact of Aβ on passive avoidance memory in rats. Moreover, combining AIT with GB supplementation led to improvements in both passive avoidance memory and long-term memory. The study also revealed a decline in long-term memory over time in the AC group. The Aβ + AIT + GB group demonstrated stable memory performance from before surgery through after 8 weeks of AIT and GB treatment, indicating that AIT and GB together may ameliorate Aβ's adverse effects on memory and result in significant enhancements. This implies that the synergy between AIT and GB could be pivotal in AD prevention. While the Aβ + GB group showed improvement, no marked difference in memory performance was found. These outcomes are consistent with prior research that examined the effects of combining physical exercise and working memory training on reducing cognitive decline in older adults diagnosed with mild clinical AD (19). Moreover, combining magnesium supplementation with treadmill exercise yielded consistent results, enhancing neurogenesis and plasticity in the hippocampus, which in turn improved memory deficits in aged mice. These findings suggest that combined treatment is more effective than either treatment alone, highlighting the synergistic role of magnesium and physical exercise in mitigating age-related memory deficits (20). Regular physical activity is widely recognized for its beneficial effects on brain function. Exercise is thought to reduce the risk of cerebrovascular diseases, diminish inflammation, improve brain structure through the stimulation of growth factors, and lessen amyloid accumulation. Biologically, the improvement of insulin resistance is linked to better synaptic function and increased brain activity, contributing to cognitive function enhancement (21). Eight weeks of endurance training showed an increase in periodic behaviors, delayed entry into the dark room, and reduced time spent in the dark room, which collectively enhanced the passive avoidance memory in AD rats treated with trimethyltin (8). Furthermore, studies have shown that GB improves memory performance over time, and the molecular networks involved in GB's proliferation may regulate the expression of proteins related to cell metabolism, sustaining high activity and neuroprotection (22). Our results indicate that AIT combined with GB supplementation elevates GDNF gene expression more than exercise alone. Additionally, GB supplementation alone raised GDNF gene expression. GDNF contributes to differentiation, proliferation, and myelin formation in axons following nerve injury. Moreover, activating the GDNF signaling pathway may increase the transcription of genes involved in the repair, proliferation, or replacement of tissues or nerves damaged by hyperglycemia, facilitating normal axon regeneration in neurons (23). Aerobic exercise, as a potent factor, can aid in cell proliferation of nutritional factors and inhibit factors accelerating the process of neuron degradation, thereby improving memory and learning (24).
Ghasemi et al. demonstrated that aerobic exercise could enhance learning and increase the expression of neurotrophic factor genes, notably GDNF, in the hippocampus of rats with AD. It also reduces inflammatory markers, contributing to improved memory and learning capabilities (25). The antioxidant properties of GB are largely attributed to its effects on cerebral blood flow (CBF), the neurotransmitter system, cellular redox balance, and nitric oxide (NO) levels (26). GB has been shown to protect neurons from β-amyloid toxicity, which can lead to mitochondrial dysfunction and apoptosis (27). This study also revealed a significant increase in GDNF expression levels in the group receiving GB supplementation. Research involving patients with multiple sclerosis (MS) found that GB treatment facilitated myelin sheath repair and increased the expression of BDNF and GDNF in astrocytes, suggesting that GB prompts astrocytes to produce neurotrophic factors. These factors potentially promote the formation and differentiation of oligodendrocytes in demyelinated areas (28). Surgical stress had a minimal impact on memory and learning in the SS group, where animals only underwent surgery at the study's outset. However, a significant influence was observed on GDNF gene expression, highlighting the need for further research in this area and investigation into the molecular mechanisms involved.
5.1. Conclusions
The findings indicate that the synergistic application of AIT and GB supplementation significantly boosted passive avoidance memory and long-term memory, as well as GDNF gene expression in rats with AD. The modest enhancement observed with GB supplementation alone might be attributed to the administration's duration and dosage. Additionally, the type, intensity, and duration of the exercise regimen could influence the observed lack of AIT effects on GDNF gene expression.
Acknowledgements
References
-
1.
Bagheri S, Rashno M, Salehi I, Karimi SA, Raoufi S, Komaki A. Geraniol improves passive avoidance memory and hippocampal synaptic plasticity deficits in a rat model of Alzheimer's disease. Eur J Pharmacol. 2023;951:175714. [PubMed ID: 37054939]. https://doi.org/10.1016/j.ejphar.2023.175714.
-
2.
Chan HH, Leong YQ, Voon SM, Pan ML, Leong CO, Lim CL, et al. Effects of Amyloid Precursor Protein Overexpression on NF-kappaB, Rho-GTPase and Pro-Apoptosis Bcl-2 Pathways in Neuronal Cells. Rep Biochem Mol Biol. 2021;9(4):417-25. [PubMed ID: 33969135]. [PubMed Central ID: PMC8068452]. https://doi.org/10.52547/rbmb.9.4.417.
-
3.
Numakawa T, Kajihara R. Neurotrophins and Other Growth Factors in the Pathogenesis of Alzheimer's Disease. Life (Basel). 2023;13(3). [PubMed ID: 36983803]. [PubMed Central ID: PMC10051261]. https://doi.org/10.3390/life13030647.
-
4.
Krashia P, Nobili A, D'Amelio M. Unifying Hypothesis of Dopamine Neuron Loss in Neurodegenerative Diseases: Focusing on Alzheimer's Disease. Front Mol Neurosci. 2019;12:123. [PubMed ID: 31156387]. [PubMed Central ID: PMC6534044]. https://doi.org/10.3389/fnmol.2019.00123.
-
5.
Mitra S, Turconi G, Darreh-Shori T, Matlik K, Aquilino M, Eriksdotter M, et al. Increased Endogenous GDNF in Mice Protects Against Age-Related Decline in Neuronal Cholinergic Markers. Front Aging Neurosci. 2021;13:714186. [PubMed ID: 34475820]. [PubMed Central ID: PMC8406776]. https://doi.org/10.3389/fnagi.2021.714186.
-
6.
Yazdani H, Soltani BT, Babaei P. Effects of Kiss Peptin13 on Spatial Memory in Streptozotocin-induced Alzheimer's Disease (AD) in Rats. Journal of Guilan University of Medical Sciences. 2015;24(93).
-
7.
Yu F, Vock DM, Zhang L, Salisbury D, Nelson NW, Chow LS, et al. Cognitive Effects of Aerobic Exercise in Alzheimer's Disease: A Pilot Randomized Controlled Trial. J Alzheimers Dis. 2021;80(1):233-44. [PubMed ID: 33523004]. [PubMed Central ID: PMC8075384]. https://doi.org/10.3233/JAD-201100.
-
8.
Akbari F, Moghadasi M, Farsi S, Edalatmanesh MA. The effect of eight weeks moderate-intensity endurance training with saffron intake on memory and learning in rats with trimethytin model of alzheimer’s disease. Journal of Applied Exercise Physiology. 2019;15(30):115-28.
-
9.
Abdel-Zaher AO, Farghaly HSM, El-Refaiy AEM, Abd-Eldayem AM. Protective effect of the standardized leaf extract of Ginkgo biloba (EGb761) against hypertension-induced renal injury in rats. Clin Exp Hypertens. 2018;40(8):703-14. [PubMed ID: 29351002]. https://doi.org/10.1080/10641963.2018.1425421.
-
10.
Assi AA, Farrag MMY, Badary DM, Allam EAH, Nicola MA. Protective effects of curcumin and Ginkgo biloba extract combination on a new model of Alzheimer's disease. Inflammopharmacology. 2023;31(3):1449-64. [PubMed ID: 36856916]. [PubMed Central ID: PMC10229698]. https://doi.org/10.1007/s10787-023-01164-6.
-
11.
Liu H, Ye M, Guo H. An Updated Review of Randomized Clinical Trials Testing the Improvement of Cognitive Function of Ginkgo biloba Extract in Healthy People and Alzheimer's Patients. Front Pharmacol. 2019;10:1688. [PubMed ID: 32153388]. [PubMed Central ID: PMC7047126]. https://doi.org/10.3389/fphar.2019.01688.
-
12.
Shahidi S, Ghahremanitamadon F, Soleimani Asl S, Komaki A, Afshar S, Hashemi-Firouzi N. Electrophysiological, behavioral and molecular study of vitamin E and Ginkgo biloba in a rat model of Alzheimer’s disease. Res J Pharmacogn. 2021;8(1):39-51.
-
13.
Hasanvand B, Farhadi A. Effect of Combined Exercise and Ginkgo Biloba Supplementation for 8 Weeks on Brain-derived Neurotrophic Factor Level in Depressed Older Men. Salmand. 2021;16(2):234-47. https://doi.org/10.32598/sija.16.2.2805.1.
-
14.
Shamsipour S, Sharifi G, Taghian F. Impact of interval training with probiotic (L. plantarum / Bifidobacterium bifidum) on passive avoidance test, ChAT and BDNF in the hippocampus of rats with Alzheimer's disease. Neurosci Lett. 2021;756:135949. [PubMed ID: 33974953]. https://doi.org/10.1016/j.neulet.2021.135949.
-
15.
Jadhav R, Kulkarni YA. The Combination of Baicalein and Memantine Reduces Oxidative Stress and Protects against beta-amyloid-Induced Alzheimer's Disease in Rat Model. Antioxidants (Basel). 2023;12(3). [PubMed ID: 36978955]. [PubMed Central ID: PMC10045767]. https://doi.org/10.3390/antiox12030707.
-
16.
Naghibi S, Shariatzadeh Joneydi M, Barzegari A, Davoodabadi A, Ebrahimi A, Eghdami E, et al. Treadmill exercise sex-dependently alters susceptibility to depression-like behaviour, cytokines and BDNF in the hippocampus and prefrontal cortex of rats with sporadic Alzheimer-like disease. Physiol Behav. 2021;241:113595. [PubMed ID: 34536437]. https://doi.org/10.1016/j.physbeh.2021.113595.
-
17.
Sadeghinejad M, Soltani Z, Afzalpour ME, Khaksari M, Pourranjbar M. What is the combined effect of intense intermittent exercise and Ginkgo biloba plant on the brain neurotrophic factors levels, and learning and memory in young rats? Pharmacol Rep. 2019;71(3):503-8. [PubMed ID: 31009841]. https://doi.org/10.1016/j.pharep.2019.02.006.
-
18.
Zavvari F, Karimzadeh F. A Review on the Behavioral Tests for Learning and Memory Assessments in Rat. The Neuroscience Journal of Shefaye Khatam. 2017;5(4):110-24. https://doi.org/10.18869/acadpub.shefa.5.4.110.
-
19.
Lam LCW, Chan WC, Kwok TCY, Lee JSW, Yu BML, Lee S, et al. Combined physical exercise-working memory training on slowing down cognitive decline in elders with mild clinical Alzheimer disease: a randomised controlled study (abridged secondary publication). Hong Kong Med J. 2022;28 Suppl 3(3):28-30. [PubMed ID: 35701227].
-
20.
El-Domiaty HF, El-Roghy ES, Salem HR. Combination of magnesium supplementation with treadmill exercise improves memory deficit in aged rats by enhancing hippocampal neurogenesis and plasticity: a functional and histological study. Appl Physiol Nutr Metab. 2022;47(3):296-308. [PubMed ID: 35225658]. https://doi.org/10.1139/apnm-2021-0133.
-
21.
Imaoka M, Nakao H, Nakamura M, Tazaki F, Hida M, Imai R, et al. Improvement of memory function via a combination of exercise and soy peptide supplementation in community-dwelling older adults: A randomized controlled trial. Contemp Clin Trials Commun. 2022;30:100998. [PubMed ID: 36124312]. [PubMed Central ID: PMC9482121]. https://doi.org/10.1016/j.conctc.2022.100998.
-
22.
Soliani AG, Muratori BG, dos Santos AL, Sartorelli P, Cerutti SM. Standardized extract of Ginkgo biloba enhances memory persistence over time. Phytomedicine Plus. 2023;3(2). https://doi.org/10.1016/j.phyplu.2023.100441.
-
23.
Kaki A, Karimi M. The effect of aerobic exercise with melatonin on GDNF gene expression and some indicators of oxidative stress in male rats with diabetic neuropathic pain. Daneshvar Medicine. 2021;29(3):132-46.
-
24.
Song LJ, Sui RX, Wang J, Miao Q, He Y, Yin JJ, et al. Targeting the differentiation of astrocytes by Bilobalide in the treatment of Parkinson's disease model. Int J Neurosci. 2024;134(3):274-91. [PubMed ID: 36037147]. https://doi.org/10.1080/00207454.2022.2100778.
-
25.
Ghasemi P, Gharakhanlou R, Shamsi MM, Khodadadi D. The Effect of 4 Weeks Aerobic Exercise on Gene Expression of Glial Cell_Derived Neurotrophic Factor, TNF-α and Cognition in Rat’s Hippocampus with Alzheimer's Disease Induced by Amyloid Beta. Sport Phys. 2021;13(49):98-169.
-
26.
Nowak A, Kojder K, Zielonka-Brzezicka J, Wrobel J, Bosiacki M, Fabianska M, et al. The Use of Ginkgo Biloba L. as a Neuroprotective Agent in the Alzheimer's Disease. Front Pharmacol. 2021;12:775034. [PubMed ID: 34803717]. [PubMed Central ID: PMC8599153]. https://doi.org/10.3389/fphar.2021.775034.
-
27.
Liu X, Hao W, Qin Y, Decker Y, Wang X, Burkart M, et al. Long-term treatment with Ginkgo biloba extract EGb 761 improves symptoms and pathology in a transgenic mouse model of Alzheimer's disease. Brain Behav Immun. 2015;46:121-31. [PubMed ID: 25637484]. https://doi.org/10.1016/j.bbi.2015.01.011.
-
28.
Yin JJ, He Y, An J, Miao Q, Sui RX, Wang Q, et al. Dynamic Balance of Microglia and Astrocytes Involved in the Remyelinating Effect of Ginkgolide B. Front Cell Neurosci. 2019;13:572. [PubMed ID: 31969806]. [PubMed Central ID: PMC6960131]. https://doi.org/10.3389/fncel.2019.00572.