Abstract
Background:
The Artemisia genus is one of the largest genera of the Asteraceae family, with various traditional and modern reported uses. Within this group, Artemisia ciniformis (Dermane Talaei) is an endemic plant of Iran with antioxidant, antimalarial, anticancer, cytoprotective, and neuroprotective properties. Due to these potential benefits, further phytochemical research needs to be conducted to understand its chemotaxonomic and pharmaceutical effects.Objectives:
The main purpose of this study is to investigate the dichloromethane fraction of A. ciniformis from Iran for phytochemical and chemotaxonomic aspects.Methods:
Artemisia ciniformis aerial parts were collected, dried, and extracted by the maceration method using dichloromethane after defatting. The prepared extract was subjected to chromatographic techniques, including column chromatography, vacuum liquid chromatography, and HPLC, to isolate purified compounds, which were identified using spectroscopic analyses such as NMR and EI-MS.Results:
Two tetrahydrofuran-type sesquiterpenoids: (1) hydroxydavanone and (2) arteincultone; two acetophenone derivatives; (3) xanthoxylin and (4) 2,4-dihydroxy-6-methoxy acetophenone; and a flavonoid, (5) jaceosidin, were isolated. Additionally, a polymethoxylated flavone was obtained in small amounts, and its structure was tentatively assigned as (6) 5,6-dihydroxy-2-(3-hydroxy-4,5-dimethoxyphenyl)-7-methoxy-4H-chromen-4-one (Ciniformon).Conclusions:
Artemisia ciniformis is a rich source of phytochemicals from various categories, such as sesquiterpenes and phenolic compounds, that can be used in systematic studies and medicinal research to treat human disorders by discovering new biologically active as well as lead compounds.Keywords
Acetophenone Derivative Artemisia ciniformis Chemotaxonomy Flavonoid NMR Sesquiterpenoid
1. Background
The genus Artemisia L., as one of the largest genera in the family Asteraceae, consists of more than 500 species distributed generally in the northern temperate zone of the world (1, 2). In Iran, 34 Artemisia species have been reported and considered as dominant species of this region, which grow in various geographic areas from high mountains to deserts (3, 4). Various species of this genus have been used since ancient times in folk remedies for the treatment of different diseases including upper respiratory infections, coughs, and colds, urogenital issues like diuresis. It also acts as a digestive aid with carminative, laxative, and antispasmodic properties, helps in liver dysfunctions as a choleretic, treats gynecological problems like abnormal menstruation, and aids in difficult labor, central nervous system diseases including epilepsy and insomnia, and dermal problems such as wounds, allergic rashes, and dermatitis (1, 5-7). Among them, Artemisia ciniformis Krasch. & Popov ex Poljakov (Asteraceae, in Persian: Dermane Talaei) is a plant that grows in central Asia and the Northeast of Iran (8). Scientific research has shown that extracts and essential oil of Artemisia ciniformis have biological effects including antioxidant, antimalarial, antibacterial, anticancer, cytoprotective, and neuroprotective activities (9-15). In more detailed studies performed with the fractionation of total extracts with different solvents, there are several reports of more significant efficacy of the dichloromethane fraction in comparison with others (9-12, 14, 16). However, the identity of the dichloromethane fraction containing compounds has been clarified only by thin-layer chromatography (TLC) that has shown the probable presence of terpenoids and steroids (16). The presence of high biological activity in the dichloromethane fraction necessitates its purification and structure elucidation of phytochemicals present in it. This can lead to the discovery of new biologically active constituents and lead compounds, expanding the existing chemotaxonomic information.
2. Objectives
This study was conducted to investigate the dichloromethane fraction of A. ciniformis from Iran for phytochemical and chemotaxonomical characteristics.
3. Methods
3.1. General
Extraction procedure solvents were obtained from Dr. Mojallali Company, I.R. Iran. High-performance liquid chromatography (HPLC)-graded solvents, TLC plates (silica gel GF254 plates, 20 × 20 cm, 0.5 mm), and column chromatography stationary phase (Silica gel 60, 0.063-0.200 µm) were purchased from Merck, Germany. A Young Lin apparatus, equipped with a YL 9111S pump and YL 9160 PDA detector, was used for HPLC procedures. Also, a Vertica Reverse phase column (RP18 250 × 30 mm) or KNAUER normal phase column (250 × 20 mm) was applied as necessary. Recording NMR spectra was performed by a 500 MHz Bruker spectrometer using deuterated chloroform (CDCl3) as solvent.
3.2. Plant Material
In September 2011, aerial parts of Artemisia ciniformis were collected from populations growing in Tandoureh National Park, Khorasan Razavi Province, I.R. Iran. The plant material was identified by Dr. Valiollah Mozaffarian, a botanist at the Research Institute of Forests and Rangelands, and voucher number (12569) was deposited in the herbarium of the Faculty of Pharmacy, Mashhad University of Medical Sciences, Mashhad, I.R. Iran.
3.3. Extraction and Isolation
Plant material was dried in shade and ground to prepare for extraction. Fatty compounds were removed by petroleum ether (bp 40 - 60°C) (2.4 L × 3) extraction of 240 g of powdered plant material, resulting in 12.76 g (5.31 % w/w) extract after solvent evaporation. Successively, the residual plant material was exposed to dichloromethane (DCM) (2.4 L × 3) to yield 27.86 g (11.60 % w/w) extract. Solvent evaporation in both extraction procedures was performed at 45°C under vacuum conditions using a rotary evaporator. The DCM extract was fractionated by vacuum liquid chromatography (VLC) using a normal column of silica gel 60 and a mixture of organic solvents (heptane: Ethyl acetate, 10→100) to obtain 11 fractions, A - K, based on TLC evaluation. These fractions were then injected into HPLC in the following order to isolate pure compounds
For HPLC, either normal phase or reverse phase columns were used, employing n-hexane (n-Hex): Ethyl acetate (EtOAc) in the former and water: Methanol (MeOH) in the latter as the gradient mobile phase.
Fraction B was further divided into 10 subfractions, B1 - B10, using a normal phase column. Based on purity investigations by TLC, subfraction B10 was chosen for further inspection, resulting in the identification of compound 3: Xanthoxylin.
Similarly, a portion of fraction E was divided into 6 subfractions, E1 - E6. Subfraction E3 was further divided into 7 secondary subfractions (E3a-E3g) after passing through an HPLC normal column. The first subfraction was identified as 2,4-dihydroxy-6-methoxy acetophenone, compound 4. Another portion was split into two parts using an HPLC reverse column, with the second part identified as arteincultone (2).
Following this isolation process, fraction F was run over an HPLC reverse column, resulting in the isolation of compound 1: Hydroxydavanone.
Fraction H underwent inspection using an HPLC reverse column, yielding subfractions H1, H2, and H3. Further investigations using spectroscopic techniques identified H1 as jaceosidin (5) and H2 as a new flavone derivative compound tentatively named ciniformon (6).
3.4. UV Analysis
The UV visible spectrophotometer (Shimadzu, UVmini-1240) was used to record the absorption from pure compounds dissolved in methanol. Additionally, shift reagents including sodium methoxide (CH3ONa), sodium acetate (CH₃COONa), boric acid (B(OH)3), aluminum chloride (AlCl3), and hydrochloric acid (HCl) solutions were used to measure the bathochromic shift (17).
4. Results
Compound (1): hydroxydavanone; C15H24O3 (Figure 1); MW 252.35 g/mol. 1H-NMR and 13C-NMR spectroscopic data are presented in Appendix 1.
Isolated compounds from DCM extract of Artemisia ciniformis
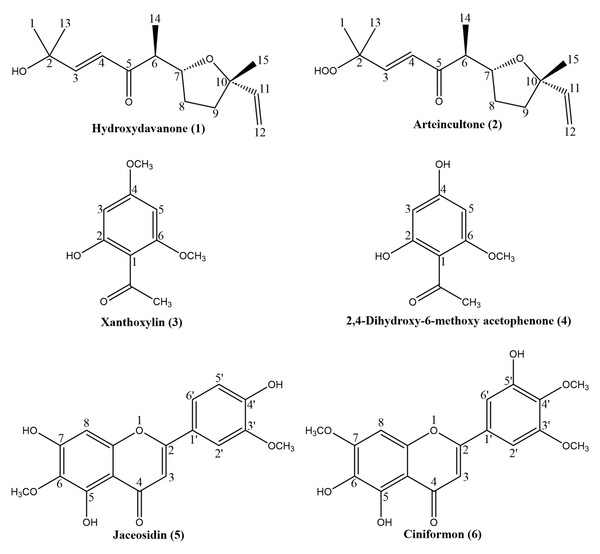
Compound (2): Arteincultone; C15H24O4 (Figure 1); MW 268.35 g/mol. 1H-NMR and 13C-NMR spectroscopic data are presented in Appendix 1.
Compound (3): Xanthoxylin; C10H12O4 (Figure 1); MW 196.20 g/mol. 1H-NMR and 13C-NMR spectroscopic data are presented in Appendix 1.
Compound (4): 2,4-dihydroxy-6-methoxyacetophenone; C9H10O4 (Figure 1); MW 182.18 g/mol. 1H-NMR and 13C-NMR spectroscopic data are presented in Appendix 1.
Compound (5): Jaceosidin; C17H14O7 (Figure 1); MW 330.29 g/mol. 1H-NMR and 13C-NMR spectroscopic data are presented in Appendix 1.
Compound (6): Ciniformon; C18H16O8 (Figure 1); MW 360.32 g/mol. 1H-NMR, EI-MS, and UV spectroscopic data are presented in Appendix 1.
5. Discussion
5.1. Structure Elucidation of Isolated Compounds
Compound 1's decoupled 13C-NMR spectrum showed 15 carbon atoms, which, considering the DCM solvent extraction and abundance of sesquiterpenes in the Asteraceae family, was supposed to be a sesquiterpenoid. Inspection of the broad bond (BB) 1H-NMR peaks and their integrities revealed 4 methyl groups (δH 1.04, 1.26 & 1.39). Meanwhile, a couple of pointed methyls that made a sharp singlet peak (δH 1.39) with an integrity of 6 protons were joined to an oxygenated quaternary carbon according to prepared HMBC. In this way, the presence of an isopropanoyl moiety in the purified compound was assumed. Furthermore, one of the four methyl groups depicted a doublet (δH 1.04, J = 7), which, upon recorded HMBC, proved its connection with a methine (δH 2.91) group. Moreover, the 13C-NMR determined 4 carbon atoms that were involved in an olefinic system, which the COSY spectrum connected two by two (δC 152.6 & 125.2, 144.6 & 111.4). Among the defined double bonds, one pair admitted attachment with the previously recognized isopropanoyl by a carbon that resonated at δC 152.6. The 13C-NMR displayed a carbonyl group (δC 203.0) that had no resonance with protons in HSQC, administrating a ketone functional group. HMBC exhibited the described ketone located between a carbon atom contributed to a double bond (δC 125.2, C-H, δH 6.43) and a methine group (δC 49.8) that was discussed above. Taking known parts together and HMBC correlations, it was defined that the isopropanoyl group (δC 29.2, 70.9, 29.3), one of the double bond systems (δC 152.6 & 125.2), ketone functional group (δC 203.0), methine group (δC 49.8), and one of the methyls (δC 13.0) linked to each other respectively [(H3C)2COH-CH=CH-CO-CH-CH3]. Without mentioned groups and parts, there existed two oxygenated carbon, including a methine (δC 80.4) and a quaternary carbon (δC 82.9), as well as two methylenes (δC 29.3 & 37.5), which the COSY spectrum clarified their neighboring, and totally their order was determined using HMBC investigation as -CH-CH2-CH2-C- in which terminal carbons (δC 80.4, 82.9) are connected to oxygen. This feature points out a 5-member oxygen-containing heterocyclic fragment like tetrahydrofuran, which is found in davanone derivatives of sesquiterpenes. Further studies on HMBC demonstrated that the identified tetrahydrofuran linked to the previously recognized methine (δC 49.8) by means of cycle-participating methine (δC 80.4) from one side, and on the other side, tetrahydrofuran quaternary carbon jointed to a methyl (δC 26.5) and methylene (δC 144.6). Finally, the foregoing characteristics, as well as published papers (18), determined that compound 1 was hydroxydavanone.
Investigation of compound 2's BB 1H-NMR showed a principal backbone similarity with compound 1, which literature review and analyzing references (19, 20) concluded that compound 2 is a sesquiterpenoid molecule called arteincultone.
Compound 3 exhibited aromatic and aliphatic structures in BB 1H-NMR. The aromatic structure was shown in the downfield by a couple of protons (δH 5.92 & 6.06), and their identical coupling constant (J = 2.4) indicated long-range splitting, which can be a sign of a meta position between protons. Also, the aliphatic 1H-NMR field showed the presence of two methoxy groups (δH 3.82 & 3.85) which related to aromatic carbons (δC 90.2 & 92.7) respectively. Another methyl group (δH 2.61) was identified that was joined to a carbonyl (δC 203.2) functional group and confirmed an acetyl connection to the aromatic 6-membered ring, which pointed out to be an acetophenone derivative structure with two methoxyl substituents. Noted features and literature review (21-24) lead to the identification of the isolated molecule as xanthoxylin.
Comparison of 1H-NMR and 13C-NMR spectra of compound 4 with identified xanthoxylin confirmed the acetophenone skeleton of compound 4. Without acetyl and phenyl contributing peaks, a singlet peak with an integration of 3 was detected in the upfield that pointed to a methoxyl substituent on the phenyl group. Also, two other oxygenated aromatic carbons remained that implied a hydroxyl group on the phenyl. Eventually, taking these features into consideration and reviewing literature (24, 25), compound 4 was determined to be 2,4-dihydroxy-6-methoxy acetophenone.
Observing peaks with the loss of small molecules and/or radicals from the parent ion in the mass spectrum, such as those of 13 (CH3), 18 (H2O), 28 (CO), and 48 (CH2CO) a.u.m, indicated phenolic hydroxyl and methyl groups, suggesting a flavonoid structure that is not glycosylated (26, 27). The 1H-NMR spectrum presented 5 peaks with an integrity of one, showing aromatic protons related to the A and B rings of a probable flavonoid. Additionally, 2 sharp singlet peaks with an integrity of three were seen in the BB, indicating 2 methyl groups and suggesting a methoxyflavonoid. Considering the basic backbone of flavonoids, along with having 2 methyl groups and 5 aromatic protons and no aliphatic proton, it was demonstrated that the purified compound is a methoxylated flavone with three hydroxyl groups. Along with this and literature review (28), compound 5 was identified as a known flavonoid, jaceosidin.
The mass spectrum of compound 6 presented a 360 m/z peak [M]+ as a molecular ion with losses of 18 Da (H2O), 15 Da (CH3), and 28 Da (CO) fragments, indicating the existence of phenolic hydroxyl and methyl groups of a non-glycosylated flavonoid (26, 27). Comparison of the 1H-NMR spectrum with the identified compound 5 determined the H3 and H8 positions with δH of 6.67 (s) and 6.61 (s) respectively. Additionally, detected singlet peaks with an integrity of 3 protons at δH 3.89, 3.91, and 3.96 confirmed the presence of three methoxyl functions in the molecule. Regarding the fragmentation pattern of various classes of flavonoids and the existence of 153 m/z and 179 m/z peaks in EI-MS indicated that the purified compound has a flavone structure with a methoxy group on ring A and two ones on ring B (27). UV spectroscopy of the methanolic solvent of the pure compound showed maximum absorption at λ 335 and 275 nm. Measurement of these peaks with bathochromic shifts by adding different chemicals, including CH3ONa, AlCl3, AlCl3/HCl, CH3COONa, and CH3COONa/B(OH)3, and stability of caused changes indicated the position of methoxy and hydroxyl groups. In this way, methoxy groups were assigned on 7, 3ʹ, and 4ʹ, and free hydroxyls were suggested for 5, 6, and 5ʹ positions (17). Also, the two remaining aromatic protons δH 7.15 (d, J = 2, 1H) and δH 7.12 (d, J = 2, 1H) of 1H-NMR were assigned to the 2ʹ and 6ʹ locations of the B ring. It should be noted that compound 6 was isolated in a very small amount, and for this reason, all the spectroscopic investigations were not possible with 1H-NMR, EI-MS, and UV analysis. However, the experiments carried out so far indicated a new flavone derivative which the authors have tentatively named ciniformon (3ʹ,5,6-trihydroxy-4ʹ,5ʹ,7-trimethoxyflavone). To the best of our knowledge, it has not been reported previously.
5.2. Chemotaxonomic Significance
Plants have played an important role in the treatment of human diseases since ancient times, serving as major contributors of natural products. Traditionally, these compounds have been used as a part of foods or folk medicines, and recently, after isolation, as pharmaceutically pure compounds or lead compounds. Moreover, natural products, especially secondary metabolites, form the basis of chemotaxonomy. This method of classification studies the distribution of chemical compounds or groups of chemicals produced by living organisms, particularly in the plant kingdom, among different plant species that are related or potentially related. Secondary metabolites and their biosynthetic pathways are often limited to a specific taxon, suggesting them as proper markers for classification. This kind of classification shows which species are phytochemically closer to each other, and so the targeted compounds can be predicted to be located in them. Thus, chemotaxonomic studies direct plant selection for pharmacological investigations, taking into account their metabolites as possible biologically active ingredients (29).
The Artemisia genus is the largest group of the Anthemideae tribe of the Asteraceae family, comprising about 500 species found mostly in the northern hemisphere (30). Various investigations have been conducted on the Artemisia genus, revealing the presence of different categories of metabolites, mainly phenolics (including glycosylated and unglycosylated flavonoids, tannins, and phenolic acids), terpenoids (such as monoterpenes, sesquiterpenes, and diterpenes), and alkaloids (31). Additionally, other compounds like coumarins, caffeoylquinic acids, sterols, polyketides, fatty acids, carbohydrates, proteins, and acetylenes have also been reported (1, 31, 32). Given that Artemisia belongs to the Asteraceae family, it is assumed that A. ciniformis is also rich in essential oils. Therefore, the majority of phytochemical investigations on A. ciniformis have focused on these essential oil components (15, 33-37). The essential oil, mostly extracted by distillation, mainly consists of camphor, 1,8-cineole, trans-pinocarveol, myrcene, and linalool as major monoterpenoid constituents of the aerial parts oil (35, 37). In addition to the monoterpenoid content, a high amount of davanone, categorized as a sesquiterpenoid, has been reported (37). However, in the distillation method, some lipophilic compounds may not be detectable, and the process may subject the compounds to heat and humidity, potentially inducing chemical reactions. Meanwhile, analyzing lipophilic phytochemicals using extraction methods provides a better understanding of the low-polarity phytochemicals of A. ciniformis. On the other hand, in bioactivity evaluations conducted on A. ciniformis, fractions prepared using a low-polarity solvent, dichloromethane, have shown more potent activities than others (9-12, 14, 16). Hence, this study investigated the phytochemical profile of the defatted dichloromethane extract of A. ciniformis, resulting in the isolation of two known sesquiterpenoids, two acetophenone derivatives, and two flavonoid constituents.
Compound 1, hydroxydavanone, is found in other members of the Asteraceae family, including the Tanacetum and Pyrethrum genera (18). Additionally, hydroxydavanone has been isolated from various Artemisia species, such as A. maritima, A. inculta, A. pallens, A. laciniata, A. herba-alba, A. reptans, A. lobelia ssp. canescens, A. lobelii ssp. biasolettiana, and A. alba (18). However, this is the first instance of hydroxydavanone being isolated from A. ciniformis, which could serve as a candidate for evaluating the phylogenetic relationship between this species and other members of the Asteraceae family. Additionally, another davanone derivative, arteincultone (Compound 2), previously reported from Tanacetum vulgare and Artemisia abrotanum, was isolated and identified from A. ciniformis for the first time (19, 20). Moreover, the biosynthesis of davanone derivatives by both Artemisia and Tanacetum species suggests their chemotaxonomic proximity, which warrants further investigation.
There are several reports of the anti-cancer activity of davanone derivatives from different Artemisia species against cancerous cell lines (18, 38-43). The mechanism of action of davanone derivatives against cancer cells involves apoptosis induction through a caspase-dependent process, loss of mitochondrial membrane potential (MMP), inhibition of cell migration and invasion, and targeting of the PI3K/AKT/MAPK signaling pathway (18, 38-41). Additionally, other biological activities such as antifungal and antiosteoclastogenic activities have been reported for davanone derivatives (44, 45).
Previously known acetophenone derivatives, considered as phenolic compounds, were isolated and their structures elucidated based on spectroscopic analyses. In earlier investigations, xanthoxylin (Compound 3) was reported in various plants from different families, including Annonaceae (Annonacrassiflora), Amaryllidaceae (Ungernia severtzovii), Euphorbiaceae (Euphorbia fidjiana, Sapium sebiferum, Croton nepetifolius, Croton hieronymi), Piperaceae (Peperomia glabella), Rutaceae (Citrus limon, Zanthoxylum americanum, Zanthoxylum alatum, Euodia borbonica, Zanthoxylum budrunga, Zanthoxylum bungeanum, Acradenia frankliniae) (21, 22, 46-55). Additionally, there are reports of xanthoxylin identification in Asteraceae family members such as Blumea balsamifera, Matricaria recutita, and some Artemisia species like A. santolina, A. scotina, and A. coerulescens (56-58). In recent decades, several biological activities, including neurotransmitter-mediated contractions in nonvascular smooth muscles, antifungal, antispasmodic, antiedema, inhibition of prostaglandin synthetase and 5-lipoxygenase, and melanogenesis induction by activating tyrosinase, have been reported for xanthoxylin, warranting further investigation (52). Furthermore, 2,4-dihydroxy-6-methoxyacetophenone (Compound 4) was extracted from A. ciniformis for the first time, while it had been previously reported from Euphorbiaceae (Euphorbia fischeriana) and Asteraceae (Tanacetum Sonbolii, Artemisia annua, Artemisia iwayomogi) (25, 59). Antioxidant, antiprotozoal (against Trypanosoma brucei rhodesiense), and antibacterial (against Clostridium perfringens) activities of 2,4-dihydroxy-6-methoxyacetophenone have been reported (25, 60, 61).
The third type of compounds identified in A. ciniformis belongs to flavonoids, which constitute the major class of phenolic natural products with various basic structures, including flavanones, flavones, isoflavones, flavonols, flavanols, and anthocyanins. They exhibit a wide range of biological properties such as neuroprotective, anti-inflammatory, cardioprotective, antioxidant, anticancer, antimicrobial, and antiviral effects (62). Among flavonoids, a few compounds have methoxylated phenolic hydroxyls, leading to O-methylated or methoxylated flavonoids. Methoxylation of flavonoids increases their hydrophobicity, facilitating cell entry, preventing degradation, and enhancing their chemopreventive potency compared to unmethoxylated flavonoids. Additionally, methylation protects dietary flavonoids from rapid hepatic metabolism. These characteristics suggest that methoxylated flavonoids have higher bioavailability and bioactivity than unmethoxylated ones. Moreover, since methoxylated flavonoids are less common than flavonoids or flavonoid glycosides, they are suitable to be used as chemotaxonomic markers (63).
Jaceosidin (Compound 5), a methoxylated flavonoid with a flavone scaffold, has been detected in various taxonomic branches, including Rubiaceae (Scyphiphora hydrophyllacea, Psychotria serpens), Scrophulariaceae (Eremophila glabra), Eriocaulaceae (Eriocaulon buergerianum), and predominantly in asters (64-67). In the Asteraceae family, jaceosidin is distributed among several genera such as Eupatorium (E. lindleyanum), Saussurea (S. medusa), and particularly Artemisia (A. argyi, A. frigida, A. asiatica, A. princeps, A. sacrorum, A. iwayomogi, A. princeps, A. juncea, A. alba, A. monosperma, A. frigida, A. santolina, A. californica, A. baldshuanica) (68-73). Thus, this compound can be proposed as a probable Artemisia phytochemical marker within the Asteraceae genera. Recent research has confirmed the biological activities of jaceosidin, including antioxidant, anti-inflammatory, anti-allergic, antibacterial, and anticancer activities, which have also been reported in various Artemisia species (42, 74).
A new methoxylated flavonoid with a flavone skeleton was tentatively identified as 5,6-dihydroxy-2-(3-hydroxy-4,5-dimethoxyphenyl)-7-methoxy-4H-chromen-4-one (Compound 6). This flavone has not been reported previously, and we have named it ciniformon. Further spectral analysis is required, and if its structure can be elucidated further, it could serve as a chemotaxonomic indicator for future studies. Considering the proposed structure of ciniformon, similar to other methylated flavonoids, biological activities such as antioxidant, anticancer, and immunomodulatory effects can be anticipated (63).
5.3. Conclusions
In conclusion, the Artemisia genus is a rich source of phytochemicals, among which A. ciniformis is relatively understudied. This phytochemical investigation yielded several compounds from various categories, including tetrahydrofuran-type sesquiterpenoids: (1) hydroxydavanone and (2) arteincultone; acetophenone derivatives; (3) xanthoxylin and (4) 2,4-dihydroxy-6-methoxy acetophenone; and flavonoids; (5) jaceosidin and a tentatively identified polymethoxylated flavone (6) 5,6-dihydroxy-2-(3-hydroxy-4,5-dimethoxyphenyl)-7-methoxy-4H-chromen-4-one (Ciniformon). This study demonstrated, as predicted, that A. ciniformis is a rich source of sesquiterpenes and phenolic compounds that can be utilized in medicinal research to treat human ailments and diseases by discovering new biologically active compounds and lead compounds.
Acknowledgements
References
-
1.
Bora KS, Sharma A. The genus Artemisia: a comprehensive review. Pharm Biol. 2011;49(1):101-9. [PubMed ID: 20681755]. https://doi.org/10.3109/13880209.2010.497815.
-
2.
Hayat MQ, Ashraf M, Khan MA, Mahmood T, Ahmad M, Jabeen S. Phylogeny of Artemisia L.: recent developments. African Journal of Biotechnology. 2009;8(11).
-
3.
Heshmati GA. Vegetation characteristics of four ecological zones of Iran. International Journal of plant production. 2007;1(2):25-224.
-
4.
Mozaffarian V. A dictionary of Iranian plant names. Tehran: Farhang Moaser. 1996;396(2):396-8.
-
5.
Abad MJ, Bedoya LM, Apaza L, Bermejo P. The artemisia L. Genus: a review of bioactive essential oils. Molecules. 2012;17(3):2542-66. [PubMed ID: 22388966]. [PubMed Central ID: PMC6268508]. https://doi.org/10.3390/molecules17032542.
-
6.
Obolskiy D, Pischel I, Feistel B, Glotov N, Heinrich M. Artemisia dracunculus L. (tarragon): a critical review of its traditional use, chemical composition, pharmacology, and safety. J Agric Food Chem. 2011;59(21):11367-84. [PubMed ID: 21942448]. https://doi.org/10.1021/jf202277w.
-
7.
Abad MJ, Bedoya LM, Bermejo P. Essential Oils from the Asteraceae Family Active against Multidrug-Resistant Bacteria. Fighting Multidrug Resistance with Herbal Extracts, Essential Oils and Their Components. 2013. p. 205-21. https://doi.org/10.1016/b978-0-12-398539-2.00014-8.
-
8.
Mozaffarian V. 'Compositae':'Anthemideae'&'Echinopeae'. Research Institute of Forests and Rangelands; 2008.
-
9.
Mojarrab M, Naderi R, Heshmati Afshar F. Screening of different extracts from artemisia species for their potential antimalarial activity. Iran J Pharm Res. 2015;14(2):603-8. [PubMed ID: 25901169]. [PubMed Central ID: PMC4403078].
-
10.
Emami A, Zamani Taghizadeh Rabe S, Ahi A, Mahmoudi M. Study ontoxic effects of Artemisisa spp. fractions from Iran on human cancer cell lines. Journal of Advances in Medical and Biomedical Research. 2010;18(70):58-67.
-
11.
Taghizadeh Rabe SZ, Mahmoudi M, Ahi A, Emami SA. Antiproliferative effects of extracts from Iranian Artemisia species on cancer cell lines. Pharm Biol. 2011;49(9):962-9. [PubMed ID: 21592012]. https://doi.org/10.3109/13880209.2011.559251.
-
12.
Tayarani-Najaran Z, Hajian Z, Mojarrab M, Emami SA. Cytotoxic and apoptotic effects of extracts of Artemisia ciniformis Krasch. and Popov ex Poljakov on K562 and HL-60 cell lines. Asian Pac J Cancer Prev. 2014;15(17):7055-9. [PubMed ID: 25227790]. https://doi.org/10.7314/apjcp.2014.15.17.7055.
-
13.
Mojarrab M, Jamshidi M, Ahmadi F, Alizadeh E, Hosseinzadeh L. Extracts of Artemisia ciniformis Protect Cytotoxicity Induced by Hydrogen Peroxide in H9c2 Cardiac Muscle Cells through the Inhibition of Reactive Oxygen Species. Adv Pharmacol Sci. 2013;2013:141683. [PubMed ID: 24381586]. [PubMed Central ID: PMC3867950]. https://doi.org/10.1155/2013/141683.
-
14.
Hosseinzadeh L, Mirzaei S, Hajialyani M, Ahmadi F, Emami SA, Mojarrab M. The protective effect of different extracts of aerial parts of Artemisia ciniformis against H2O2-induced oxidative stress and apoptosis in PC12 pheochromocytoma cells. J Appl Pharm Sci. 2019;9(4):16-23. https://doi.org/10.7324/japs.2019.90403.
-
15.
Taherkhani M. Chemical investigation and protective effects of bioactive phytochemicals from Artemisia ciniformis. Iran J Chem Chem Eng. 2016;35(2):15-26.
-
16.
Ramazani E, Tayarani-Najaran Z, Shokoohinia Y, Mojarrab M. Comparison of the cytotoxic effects of different fractions of Artemisia ciniformis and Artemisia biennis on B16/F10, PC3 and MCF7 Cells. Res Pharm Sci. 2020;15(3):273-80. [PubMed ID: 33088327]. [PubMed Central ID: PMC7540818]. https://doi.org/10.4103/1735-5362.288434.
-
17.
Markham KR. Techniques of flavonoid identification. 1982.
-
18.
Maggio A, Rosselli S, Brancazio CL, Spadaro V, Raimondo FM, Bruno M. Metabolites from the aerial parts of the Sicilian population of Artemisia alba. Nat Prod Commun. 2013;8(3):283-6. [PubMed ID: 23678791].
-
19.
Appendino G, Gariboldi P, Nano GM, Tétényi P. Tetrahydrofuran-type terpenoids from Tanacetum vulgare. Phytochemistry. 1984;23(11):2545-51. https://doi.org/10.1016/s0031-9422(00)84096-0.
-
20.
Rücker G, Manns D, Wilbert S. Peroxide als Pflanzeninhaltsstoffe, 12. Mitt.: Peroxide vom Davanon‐Typ aus der Eberraute (Artemisia abrotanum) und ihre Darstellung. Archiv der Pharmazie. 2006;326(8):457-60. https://doi.org/10.1002/ardp.19933260806.
-
21.
Cambie RC, Lal AR, Rutledge PS, Woodgate PD. Ent-14[S],16β,17-trihydroxyatisan-3-one and further constituents from Euphorbia fidjiana. Phytochemistry. 1991;30(1):287-92. https://doi.org/10.1016/0031-9422(91)84139-j.
-
22.
Valenciennes E, Smadja J, Conan JY. Screening for biological activity and chemical composition of Euodia borbonica var. borbonica (Rutaceae), a medicinal plant in Reunion Island. J Ethnopharmacol. 1999;64(3):283-8. [PubMed ID: 10363846]. https://doi.org/10.1016/s0378-8741(98)00137-8.
-
23.
Wang Y, Shi L, Wang A, Tian H, Wang H, Zou C. Preparation of High-Purity (–)-Borneol and Xanthoxylin from Leaves ofBlumea balsamifera(L.) DC. Sep Sci Technol. 2014;49(10):1535-40. https://doi.org/10.1080/01496395.2014.891613.
-
24.
Brown GD. Two New Compounds from Artemisia annua. J Natural Products. 2004;55(12):1756-60. https://doi.org/10.1021/np50090a006.
-
25.
Tabatabaei SM, Farimani MM, Nejad-Ebrahimi S, Salehi P. Phytochemical study of Tanacetum sonbolii aerial parts and the antiprotozoal activity of its components. Iran J Pharm Res. 2020;19(1):77.
-
26.
Bai Y, Zheng Y, Pang W, Peng W, Wu H, Yao H, et al. Identification and Comparison of Constituents of Aurantii Fructus and Aurantii Fructus Immaturus by UFLC-DAD-Triple TOF-MS/MS. Molecules. 2018;23(4). [PubMed ID: 29601542]. [PubMed Central ID: PMC6017871]. https://doi.org/10.3390/molecules23040803.
-
27.
Tsimogiannis D, Samiotaki M, Panayotou G, Oreopoulou V. Characterization of flavonoid subgroups and hydroxy substitution by HPLC-MS/MS. Molecules. 2007;12(3):593-606. [PubMed ID: 17851414]. [PubMed Central ID: PMC6149353]. https://doi.org/10.3390/12030593.
-
28.
Martínez V, Barberá O, Sánchez-Parareda J, Alberto Marco J. Phenolic and acetylenic metabolites from Artemisia assoana. Phytochemistry. 1987;26(9):2619-24. https://doi.org/10.1016/s0031-9422(00)83891-1.
-
29.
Singh R; Geetanjali. Chemotaxonomy of Medicinal Plants. Natural Products and Drug Discovery. 2018. p. 119-36. https://doi.org/10.1016/b978-0-08-102081-4.00006-x.
-
30.
Haghighi AR, Belduz AO, Vahed MM, Coskuncelebi K, Terzioglu S. Phylogenetic relationships among Artemisia species based on nuclear ITS and chloroplast psbA-trnH DNA markers. Biologia. 2014;69(7):834-9. https://doi.org/10.2478/s11756-014-0379-3.
-
31.
Turi CE, Shipley PR, Murch SJ. North American Artemisia species from the subgenus Tridentatae (Sagebrush): a phytochemical, botanical and pharmacological review. Phytochemistry. 2014;98:9-26. [PubMed ID: 24359634]. https://doi.org/10.1016/j.phytochem.2013.11.016.
-
32.
Nasseri S, Emami SA, Mojarrab M. Dicaffeoylquinic Acids from the Aerial Parts of Artemisia ciniformis Krasch. & Popov ex Poljakov. Pharm Sci. 2019;25(2):171-5. https://doi.org/10.15171/ps.2019.25.
-
33.
Jafarian T, Roghani K, Mohammadi A. Chemical constituents and antioxidant properties of Artemisia ciniformis essential oils at pre and flowering stages from Northeast of Iran. Res J Chem Environ. 2019;23(6):1-6.
-
34.
Taherkhani M. Chemical Constituents, Antimicrobial, Cytotoxicity, Mutagenic and Antimutagenic Effects of Artemisia ciniformis. Iran J Pharm Res. 2016;15(3):471-81. [PubMed ID: 27980582]. [PubMed Central ID: PMC5149034].
-
35.
Taherkhani M, Rustaiyan A, Taherkhani T. Composition of the Leaf Essential Oils ofArtemisia ciniformisKrasch. et M. Pop. ex Poljak,Artemisia oliverianaJ. Gay ex Bess. in DC. andArtemisia turanicaKrasch., Three Asteraceae Herbs Growing Wild in Iran. J Essent. 2012;15(6):1006-12. https://doi.org/10.1080/0972060x.2012.10662605.
-
36.
Firouzni A, Vahedi H, Sabbaghi F, Bigdeli M. Composition of the essential oil of Artemisia ciniformis, A. kopetdaghensis, and A. khorasanica in Iran. Chem Nat Compd. 2009;44(6):804-6. https://doi.org/10.1007/s10600-009-9212-6.
-
37.
Rustaiyan A, Masoudi S, Kazemi M. Volatile Oils Constituents from Different Parts ofArtemisia ciniformisKrasch. Et M. Pop. ex Poljak andArtemisia incana(L.) Druce. from Iran. Journal of Essential Oil Research. 2007;19(6):548-51. https://doi.org/10.1080/10412905.2007.9699328.
-
38.
Mohamed TA, Albadry HA, Elshamy AI, Younes SH, Shahat AA, El‐wassimy MT, et al. A new Tetrahydrofuran sesquiterpene skeleton from Artemisia sieberi. J Chin Chem Soc. 2020;68(2):338-42. https://doi.org/10.1002/jccs.202000198.
-
39.
Hosseinzadeh L, Shokoohinia Y, Arab M, Allahyari E, Mojarrab M. Cytotoxic and Apoptogenic Sesquiterpenoids from the Petroleum Ether Extract of Artemisia aucheri Aerial Parts. Iran J Pharm Res. 2019;18(1):391-9. [PubMed ID: 31089373]. [PubMed Central ID: PMC6487405].
-
40.
Cai J, Zhong YY, Tian S. Naturally occurring davanone terpenoid exhibits anticancer potential against ovarian cancer cells by inducing programmed cell death, by inducing caspase-dependent apoptosis, loss of mitochondrial membrane potential, inhibition of cell migration and invasion and targeting PI3K/AKT/MAPK signaling pathway. J BUON. 2020;25(5):2301-7. [PubMed ID: 33277849].
-
41.
Xiao Y, Deng T, Wang D. Davanone terpenoid inhibits cisplatin-resistant acute myeloid leukemia cancer cell growth by inducing caspase-dependent apoptosis, loss of mitochondrial membrane potential, inhibition of cell migration and invasion and targeting PI3K/AKT/MAPK signalling pathway. J BUON. 2020;25(3):1607-13. [PubMed ID: 32862611].
-
42.
Bisht D, Kumar D, Kumar D, Dua K, Chellappan DK. Phytochemistry and pharmacological activity of the genus artemisia. Arch Pharm Res. 2021;44(5):439-74. [PubMed ID: 33893998]. [PubMed Central ID: PMC8067791]. https://doi.org/10.1007/s12272-021-01328-4.
-
43.
Hussain M, Thakur RK, Khazir J, Ahmed S, Khan MI, Rahi P, et al. Traditional uses, phytochemistry, pharmacology, and toxicology of the genus Artemisia L. (Asteraceae): A high-value medicinal plant. Curr Top Med Chem. 2023. [PubMed ID: 37711006]. https://doi.org/10.2174/1568026623666230914104141.
-
44.
Vajs V, Trifunovic S, Janackovic P, Sokovic M, Milosavljevic S, Tesevic V. Antifungal activity of davanone-type sesquiterpenes from Artemisia lobelli var. conescens. J Serb Chem Soc. 2004;69(11):969-72. https://doi.org/10.2298/jsc0411969v.
-
45.
El-Desoky AH, Asano T, Maeyama Y, Kato H, Hitora Y, Goto E, et al. Arteperoxides A-C, tris-normonoterpene-sesquiterpene conjugates with peroxide-bridges from Artemisia judaica exhibiting antiosteoclastogenic activity. Phytochemistry. 2023;206:113548. [PubMed ID: 36481317]. https://doi.org/10.1016/j.phytochem.2022.113548.
-
46.
Roesler R, Catharino RR, Malta LG, Eberlin MN, Pastore G. Antioxidant activity of Annona crassiflora: Characterization of major components by electrospray ionization mass spectrometry. Food Chem. 2007;104(3):1048-54. https://doi.org/10.1016/j.foodchem.2007.01.017.
-
47.
Chermenskaya TD, Stepanycheva EA, Shchenikova AV, Savelieva EI, Chakaeva AS. Insecticidal effects of Ungernia severtzovii bulb extracts against the grain aphid Schizaphis graminum (Rondani). Industrial Crops and Products. 2012;36(1):122-6. https://doi.org/10.1016/j.indcrop.2011.08.010.
-
48.
Kouno I, Saishoji T, Sugiyama M, Kawano N. A xylosylglucoside of xanthoxylin from sapium sebiferum root bark. Phytochemistry. 1983;22(3):790-1. https://doi.org/10.1016/s0031-9422(00)86992-7.
-
49.
Catalan CA, de Heluani CS, Kotowicz C, Gedris TE, Herz W. A linear sesterterpene, two squalene derivatives and two peptide derivatives from Croton hieronymi. Phytochemistry. 2003;64(2):625-9. [PubMed ID: 12943786]. https://doi.org/10.1016/s0031-9422(03)00202-4.
-
50.
Soares MG, Felippe AP, Guimarães EF, Kato MJ, Ellena J, Doriguetto AC. 2-Hydroxy-4,6-dimethoxyacetophenone from leaves of Peperomia glabella. J Braz Chem Soc. 2006;17(7). https://doi.org/10.1590/s0103-50532006000700002.
-
51.
Hartmann G, Nienhaus F. The Isolation of Xanthoxylin from the Bark of Pbytophthora- and Hendersonula-infected Citrus limon and its Fungitoxic Effect. Journal of Phytopathology. 1974;81(2):97-113. https://doi.org/10.1111/j.1439-0434.1974.tb02784.x.
-
52.
Wu H, Niu C, Aisa HA. Research progress in small molecules as anti-vitiligo agents. Current Medicinal Chemistry. 2024;31(8):995-1035.
-
53.
Simonsen HT, Adsersen A, Bremner P, Heinrich M, Wagner Smitt U, Jaroszewski JW. Antifungal constituents of Melicope borbonica. Phytother Res. 2004;18(7):542-5. [PubMed ID: 15305313]. https://doi.org/10.1002/ptr.1482.
-
54.
Ruangrungsi N, Tantivatana P, P. Borris R, A. Cordell G. Traditional medicinal plants of Thailand. III. Constituents of Zanthoxylum budrunga (Rutaceae). J Sci Soc Thailand. 1981;7(3). https://doi.org/10.2306/scienceasia1513-1874.1981.07.123.
-
55.
Huang X, Kakiuchi N, Che Q, Huang S, Hattori M, Namba T. Effects of extracts of Zanthoxylum fruit and their constituents on spontaneous beating rate of myocardial cell sheets in culture. Phytotherapy Research. 2006;7(1):41-8. https://doi.org/10.1002/ptr.2650070111.
-
56.
Rivero-Cruz I, Pérez-Vásquez A, Mata R. Chemical Composition of the Oil from Chamomile Grown in Mexico. Planta Med. 2013;79(10). https://doi.org/10.1055/s-0033-1348785.
-
57.
Akyev B, Yusupov MI, Kasymov SZ, Sidyakin GP. Xanthoxylin from Artemisia santolina and A. scotina. Chemistry of Natural Compounds. 1973;9(3):390. https://doi.org/10.1007/bf00565699.
-
58.
Sacco T, Frattini C, Bicchi C. Constituents of the Essential Oil of Artemisia coerulescens. Planta Med. 1983;48(7):178-80. [PubMed ID: 17404981]. https://doi.org/10.1055/s-2007-969917.
-
59.
He J, Xu JK, Zhang J, Bai HJ, Ma BZ, Cheng YC, et al. Fischeriana A, a meroterpenoid with an unusual 6/6/5/5/5/6/6 heptacyclic carbon skeleton from the roots of Euphorbia fischeriana. Org Biomol Chem. 2019;17(10):2721-4. [PubMed ID: 30775763]. https://doi.org/10.1039/c9ob00013e.
-
60.
Yan X, Ding Y, Lee SH, Li W, Sun Y, Yang SY, et al. Evaluation of the antioxidant activities of natural components of Artemisia iwayomogi. Nat Prod Sci. 2014;20(3):176-81.
-
61.
Ivarsen E, Frette XC, Christensen KB, Christensen LP, Engberg RM, Grevsen K, et al. Bioassay-Guided Chromatographic Isolation and Identification of Antibacterial Compounds from Artemisia annua L. That Inhibit Clostridium perfringens Growth. J AOAC Int. 2014;97(5):1282-90. [PubMed ID: 25902977]. https://doi.org/10.5740/jaoacint.SGEIvarsen.
-
62.
Liga S, Paul C, Peter F. Flavonoids: Overview of Biosynthesis, Biological Activity, and Current Extraction Techniques. Plants (Basel). 2023;12(14). [PubMed ID: 37514347]. [PubMed Central ID: PMC10384615]. https://doi.org/10.3390/plants12142732.
-
63.
Wen L, Jiang Y, Yang J, Zhao Y, Tian M, Yang B. Structure, bioactivity, and synthesis of methylated flavonoids. Ann N Y Acad Sci. 2017;1398(1):120-9. [PubMed ID: 28436044]. https://doi.org/10.1111/nyas.13350.
-
64.
Dai H, Mei W, Wu J. Studies on chemical constituents of mangrove plant Scyphiphora hydrophyllacea. Chin Pharm J. 2006;41(19):1452.
-
65.
Zhou BD, Zhang XL, Niu HY, Guan CY, Liu YP, Fu YH. [Chemical constituents from stems and leaves of Psychotria serpens]. China J Chinese Materia Med. 2018;43(24):4878-83. Chinese.
-
66.
Algreiby AA, Hammer KA, Durmic Z, Vercoe P, Flematti GR. Antibacterial compounds from the Australian native plant Eremophila glabra. Fitoterapia. 2018;126:45-52. [PubMed ID: 29155275]. https://doi.org/10.1016/j.fitote.2017.11.008.
-
67.
Lee IJ, Chung CP, Chang SJ, Lin YL. Morphological and chemical analyses of Eriocauli Flos sold in Taiwan markets. J Food Drug Anal. 2017;25(4):939-45. [PubMed ID: 28987371]. [PubMed Central ID: PMC9328884]. https://doi.org/10.1016/j.jfda.2017.01.002.
-
68.
Wu S, Sun Q, Chu C, Zhang J. [Chemical constituents of Eupatorium lindleyanum]. China journal of Chinese materia medica. 2012;37(7):937-40. Chinese.
-
69.
Yu ZZ, Fu CX, Han YS, Li YX, Zhao DX. Salicylic acid enhances Jaceosidin and Syringin production in cell cultures of Saussurea medusa. Biotechnol Lett. 2006;28(13):1027-31. [PubMed ID: 16786264]. https://doi.org/10.1007/s10529-006-9035-5.
-
70.
Taleghani A, Emami SA, Tayarani-Najaran Z. Artemisia: a promising plant for the treatment of cancer. Bioorg Med Chem. 2020;28(1):115180. [PubMed ID: 31784199]. https://doi.org/10.1016/j.bmc.2019.115180.
-
71.
Okhundedaev BS, Bobakulov KM, Mukhamatkhanova RF, Sham’yanov ID, Abdullaev ND. New Flavone from Artemisia juncea of the Flora of Uzbekistan. Chemistry of Natural Compounds. 2019;55(5):818-20. https://doi.org/10.1007/s10600-019-02822-4.
-
72.
Trendafilova A, Todorova M, Genova V, Peter S, Wolfram E, Danova K, et al. Phenolic Profile of Artemisia alba Turra. Chem Biodivers. 2018;15(7). e1800109. [PubMed ID: 29772115]. https://doi.org/10.1002/cbdv.201800109.
-
73.
Allison BJ, Allenby MC, Bryant SS, Min JE, Hieromnimon M, Joyner PM. Antibacterial activity of fractions from three Chumash medicinal plant extracts and in vitro inhibition of the enzyme enoyl reductase by the flavonoid jaceosidin. Nat Prod Res. 2017;31(6):707-12. [PubMed ID: 27482826]. https://doi.org/10.1080/14786419.2016.1217201.
-
74.
Nageen B, Rasul A, Hussain G, Shah MA, Anwar H, Hussain SM, et al. Jaceosidin: A Natural Flavone with Versatile Pharmacological and Biological Activities. Curr Pharm Des. 2021;27(4):456-66. [PubMed ID: 32348212]. https://doi.org/10.2174/1381612826666200429095101.