Abstract
Background:
Sodium arsenite (NaAsO2) is a common mineral contaminant in drinking water in numerous parts of the world. It has been shown to have cardiovascular, metabolic, neuroendocrine, and urinary effects on the body. There is abundant scientific evidence that establishes a strong correlation between arsenic exposure and kidney-related disorders.Objectives:
The present study aimed to investigate the potential protective effect of citicoline against NaAsO2-induced nephrotoxicity.Methods:
The groups included a control group, a group treated with NaAsO2 at a concentration of 50 ppm, a group treated with Cit at a dosage of 1000 mg/kg, and three groups of NaAsO2 (50 ppm) co-treated with Cit at doses of 250, 500, and 1000 mg/kg, respectively.Results:
Citicoline decreased the level of blood urea nitrogen (P < 0.001), creatinine levels (P < 0.001), thiobarbituric acid reactive substances (P < 0.001), nitric oxide (P < 0.001), inflammatory factors tumor necrosis factor-α (P < 0.001) and interleukin-6 (P < 0.001 and P < 0.001). Furthermore, Cit increased total thiol (P < 0.001) and activity levels of catalase (P < 0.05 and P < 0.001), superoxide dismutase (P < 0.01 and P < 0.001), and glutathione peroxidase (P < 0.001). Therefore, Cit reduced the harmful effects caused by the imbalance in oxidative and antioxidant systems and histopathological damage in NaAsO2-intoxicated mice, improving the damage caused by oxidative stress and inflammation.Conclusions:
Our research shows that Cit can protect the kidneys against the damaging effects of NaAsO2 by using its antioxidant and anti-inflammatory properties.Keywords
Sodium Arsenite Oxidative Stress Inflammation Citicoline Nephroprotective Mice
1. Background
Sodium arsenite (NaAsO2) is a common mineral contaminant in drinking water in numerous parts of the world. Abundant scientific evidence establishes a strong correlation between arsenic exposure and kidney-related ailments. The World Health Organization has set a permissible limit for arsenic concentration, capping it at 10 parts per billion (ppb) (1, 2). Studies indicate that millions of people worldwide are exposed to high arsenic concentrations through drinking water (3, 4). Available literature suggests that both acute and chronic arsenic exposure are strongly linked to various diseases, including kidney problems (5, 6). The precise molecular mechanism of arsenic's nephrotoxicity remains incompletely understood. Several reports have described the relationship between arsenic metabolism and its adverse health effects. Additionally, some studies have indicated that methylated arsenic are less toxic and more easily excreted through urine. However, these studies have also supported the role of oxidative stress and inflammation in tissue damage caused by arsenic exposure (7). The primary hypothesis proposed to understand NaAsO2 toxicity revolves around oxidative stress induced by arsenic (8). NaAsO2-induced oxidative stress primarily stems from reactive oxygen species (ROS) production and interaction with sulfhydryl groups of proteins/enzymes, potentially disrupting various cell signaling pathways that may contribute to NaAsO2-induced diseases. Given the association between oxidative stress and arsenic toxicity, researchers are exploring the utilization of antioxidant properties found in compounds and extracts of various plants to mitigate complications caused by NaAsO2 (9).
Citicoline (Cit), also known as cytidine diphosphate-choline (CDP-choline), is a naturally occurring compound found in the cells of both plants and animals. It serves as an essential intermediate in the synthesis of phospholipids, crucial components of cell membranes. In nature, citicoline can be obtained from dietary sources rich in choline, such as eggs, milk, liver, and certain vegetables. Upon consumption, the body can enzymatically convert choline into Cit (10, 11). Citicoline is an endogenous nucleotide comprising cytidine and choline. Studies have demonstrated that Cit exerts cardiovascular, metabolic, neuroendocrine, and urinary effects in the body (12). Various research findings indicate that this substance prevents the elevation of thiobarbituric acid reactive substances (TBARS) levels and may also decrease levels of tumor necrosis factor-alpha (TNF-α) and interleukin-6 (IL-6) in individuals exposed to NaAsO2 poisoning (7). Citicoline's impact on parameters related to oxidative stress appears to be central to its protective effect against nephrotoxicity.
2. Objectives
As there is no existing study on the effect of Cit on NaAsO2-induced nephrotoxicity, the present study aims to investigate Cit's potential protective effect against NaAsO2-induced nephrotoxicity.
3. Methods
3.1. Chemicals
Sodium arsenite, citicoline, thiobarbituric acid (TBA), Ellman's Reagent, phosphate buffered saline, trichloroacetic acid, phosphoric acid, and Bradford solution reagent, were purchased from Sigma Aldrich Company. The kits utilized for glutathione peroxidase (GPx) and superoxide dismutase (SOD), nitric oxide (NO), IL-6, and TNF-α tests were obtained from ZellBio Company. Additionally, kits for measuring serum factors were purchased from Pars Azmoon Company.
3.2. Animals
Adult male NMRI mice, weighing between 23 to 27 g and aged 8 to 12 weeks, were sourced from the research center and animal house at Ahvaz Jundishapur University of Medical Sciences (AJUMS). Mice were provided unlimited access to food and water and were housed in controlled conditions for one week. These conditions included a 12:12-hour light/dark cycle, relative humidity of 50 - 60%, and a temperature range of 22 - 25°C. The study received approval from the Ethical Committee of Ahvaz Jundishapur University of Medical Sciences, in accordance with NIH protocols for the care and use of laboratory animals.
3.3. Ethical Considerations
The procedures for animal experiments and euthanasia were approved by the AJUMS ethics committee, following standard methods of animal care and use. (Ethics approval ID: IR.AJUMS.ABHC.REC.1400.090).
3.4. Experimental Method
The mice were divided into six experimental groups, each consisting of 8 animals. These groups comprised a control group, a group treated with NaAsO2 at a concentration of 50 ppm, a group treated with Cit at a dosage of 1000 mg/kg, and three groups treated with NaAsO2 50 ppm co-administered with Cit at doses of 250, 500, and 1000 mg/kg, respectively (13). Over the course of an eight-week study, mice were exposed to NaAsO2 at a concentration of 50 ppm in their drinking water (14). Meanwhile, the control group received a vehicle solution in their drinking water. In the final two weeks of the study, the mice received purified citicoline orally to achieve the desired dosage. Fresh drinking water containing NaAsO2 was prepared daily throughout the experiment.
Following ethical guidelines for working with laboratory animals and under the observation of designated observers from AJUMS, the mice were administered intraperitoneal anesthesia using a combination of ketamine and xylazine at a dosage of 90/10 mg/kg. Blood samples were obtained by puncture, and the resulting sera were used to measure the activity of kidney enzymes such as blood urea nitrogen (BUN) and creatinine (Cr). Kidney tissue was also collected. Right kidneys were preserved in a solution called phosphate-buffered formalin for histological analysis by a histologist, while left kidneys were frozen at -70°C for later evaluation of kidney markers.
The protein concentration of the samples was determined using the Bradford method. The samples were then homogenized in phosphate-buffered saline at a specific ratio and speed, followed by centrifugation to separate the tissues and collect the supernatants in microtubes. Precautions such as working on ice and using a protease inhibitor were taken throughout the process to ensure sample integrity and prevent damage.
3.5. Biochemical Assays
3.5.1. Kidney Serum Biomarkers
Once the serum samples were collected, the levels of BUN and Cr were evaluated following the instructions provided by the manufacturer's kit protocols.
3.5.2. Kidney Oxidative Stress
The measurement of total thiol (TT) level (15), TBARS level (16), and catalase (CAT) activity (17) were performed according to the studies mentioned.
3.5.3. Kidney Cytokine
To measure IL-6 and TNF-α in the kidney supernatant samples, ZellBio kit methods were utilized.
3.6. Histological Procedure
For histopathological examination, kidney tissue was preserved in a formalin solution and then embedded in paraffin. The samples were embedded in paraffin and were cut into 4 μm thick sections. These sections were mounted on glass slides and subjected to H&E staining. Three slides were prepared for each animal tissue, and five random microscopic fields were evaluated and scored.
3.7. Statistical Method
The data are presented as the mean ± SD. The dataset of this study was analyzed using a one-way analysis of variance (ANOVA) with Tukey's multiple comparisons as a post hoc test. Statistical significance was determined by P-values less than 0.05.
4. Results
4.1. Citicoline Effects on Serum Biomarkers
The NaAsO2 group showed significantly higher levels of BUN and Cr activities compared to the control group (P < 0.001). However, in the NaAsO2 co-treated Cit groups (250, 500, and 1000 mg/kg), there was a significant decrease in serum activity levels compared to the NaAsO2 group (P < 0.001), as depicted in Figure 1.
The effects of citicoline (Cit) on A, BUN and B, Cr in NaAsO2-induced nephrotoxicity in mice. *** P < 0.001 compared to the control group and ### P < 0.001 compared to the NaAsO2 group.
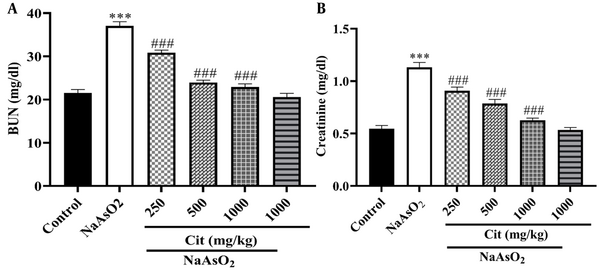
4.2. Citicoline Effects on Oxidative Stress Factors
The NaAsO2 group exhibited higher levels of TBARS and NO compared to the control group (P < 0.001). According to Figure 2, administration of NaAsO2 significantly decreased TT levels and the activity of GPx, SOD, and CAT enzymes compared to the control group (P < 0.001). However, when the NaAsO2 group was co-treated with Cit (250, 500, and 1000 mg/kg), there was a significant reduction in NO and TBARS levels and an increase in the activity of antioxidant enzymes GPx, SOD, CAT, and TT levels compared to the NaAsO2 -administered group.
A, the effects of citicoline (Cit) on the level of nitric oxide (NO); B, thiobarbituric acid reactive substances (TBARS); C, total thiol (TT); D, glutathione peroxidase (GPx); E, superoxide dismutase (SOD); and F, catalase (CAT) in NaAsO2-induced kidney injury in mice. *** P < 0.001 compared to the control group and # P < 0.05, ## P < 0.01 and ### P < 0.001 compared to the NaAsO2 group.
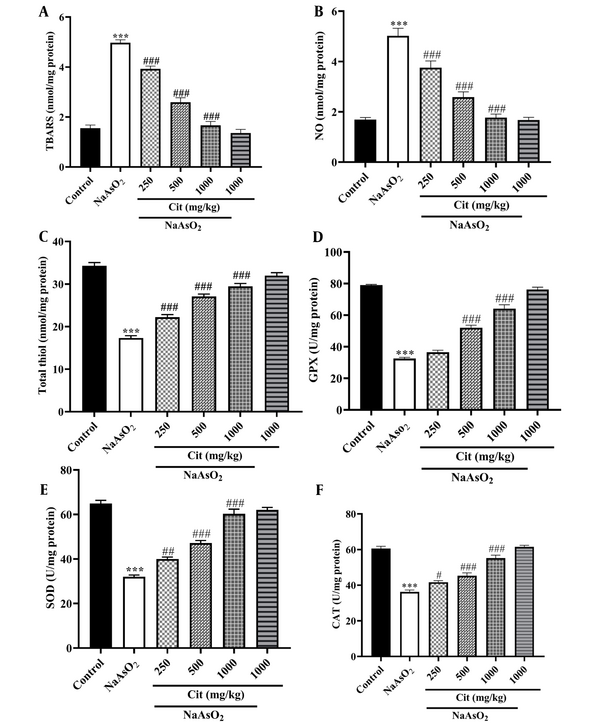
4.3. Citicoline Effects on Inflammatory Factors
TNF-α and IL-6 levels were significantly increased in the NaAsO2 group compared to the control group (P < 0.001). In experimental Cit pretreated groups, a significant decrease in TNF-α and IL-6 cytokines was observed compared to the NaAsO2 group (Figure 3).
The effects of citicoline (Cit) on the level of A, tumor necrosis factor-alpha-α (TNF-α) and B, interleukin-6 (IL-6) in NaAsO2-induced hepatotoxicity in mice. *** P < 0.001 compared to the control group and ## P < 0.01 and ### P < 0.001 compared to NaAsO2 group.
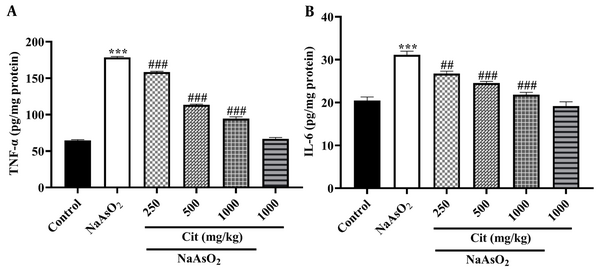
4.4. Histopathologic Findings
Slides of kidney sections taken from control animals showed normal structure, conversely, in the group exposed to as normal tissue patterns were disrupted. The NaAsO2 + Cit 1000 mg/kg group significantly inhibited NaAsO2-induced histological damage, such as inflammatory cell infiltration and congestion of blood vessels. Histological criteria were classified into four categories and given specific grades: Normal (-), weak (+), moderate (++), or intense (+++). The recorded ratings for each criterion were then averaged to obtain overall evaluations (Figure 4 and Table 1).
The effects of citicoline (Cit) on histopathological changes and lesions induced by sodium arsenite (NaAsO2) in kidney tissues of mice. The effects of Cit are evident in H&E staining. Green arrows: Proximal tube damage, yellow arrows: Inflammation, and black arrows: Congestion of blood vessels. Magnification: × 100.
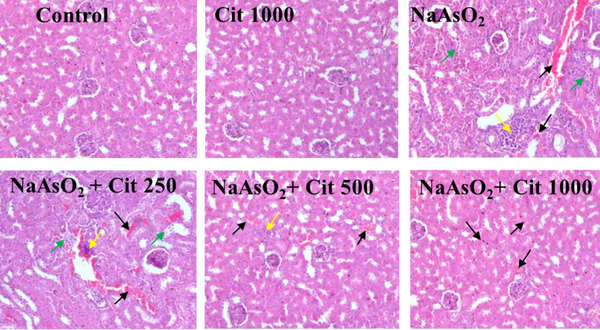
Histology Assessments in the Control and Experimental Groups
Groups | Congestion of Blood Vessels | Inflammation | Proximal Tube Damage |
---|---|---|---|
Control | - | - | - |
Citicoline (1000 mg/kg) | - | - | - |
NaAsO2 | (++) to (+++) | (+++) | (+) to (++) |
NaAsO2 + citicoline (250 mg/kg) | (++) to (+++) | (++) to (+++) | (+) to (++) |
NaAsO2 + citicoline (500 mg/kg) | (+) to (++ | (+) | (+) |
NaAsO2 + citicoline (1000 mg/kg) | (-) to (+) | (-) to (+) | (-) to (+) |
5. Discussion
Due to the increased use of agricultural pesticides and metals, environmental levels of arsenic are continuously rising, attributing chronic and long-term toxic effects to NaAsO2. Long-term exposure to NaAsO2 has detrimental effects on the nervous system, heart, reproductive organs, liver, and kidneys, leading to various health complications (18-20). Researchers have recently focused on finding safe and effective ways to treat and prevent heavy metal poisoning. A suggested approach is to use chelating agents to bind to antioxidant compounds. Another effective mechanism to prevent arsenic poisoning is to increase the concentration of intracellular antioxidants (21).
Elevated levels of BUN and serum Cr are recognized as crucial indicators of renal impairment (22). Numerous studies have documented that exposure to arsenic can lead to an increase in BUN and Cr levels (23, 24). In our research, kidney injury induced by arsenic led to elevated levels of urea nitrogen and serum Cr. However, the administration of Cit demonstrated substantial alleviation of these adverse effects, consistent with the results of the Al-Hafyan et al. study (25).
One of the most important and fundamental damages is related to oxidative stress, wherein NaAsO2 acts as a toxic agent against the body's antioxidant system. Kidney cells have specialized antioxidant enzyme systems like SOD, CAT, GPx, and TT to combat oxidative stress. SOD converts superoxide, a byproduct of oxidative stress, into hydrogen peroxide (H2O2). CAT breaks down H2O2 into oxygen and water, and GPx helps neutralize harmful lipid peroxides and H2O2 in the cells. Together, these enzymes defend kidney cells against oxidative stress (25). In the present study, the antioxidant system, including CAT, GPx, SOD, and TT, was reduced in exposure to NaAsO2, and Cit, as an antioxidant, was able to reduce the toxicity caused by NaAsO2, consistent with many studies (19, 26). Oxidative stress increases pro-inflammatory cytokines, which ultimately cause widespread inflammation. TNF-α and IL-6 are two important pro-inflammatory cytokines in the process of inflammation, which cause the activation of inflammatory cells at the site of injury. Also, by stimulating inducible nitric oxide synthase (iNOS), TNF-α can increase the amount of NO, which is one of the harmful factors (27).
In a study conducted by our research team, NaAsO2 was found to elevate the levels of pro-inflammatory factors TNF-α and IL-6. However, the administration of Cit demonstrated its potential to decrease these pro-inflammatory factors and mitigate the associated complications. This outcome closely aligns with the findings of Zhang et al. and Al-Brakati et al. studies (24, 28). Additionally, in a study conducted by Nikravesh et al., Cit decreased factors such as TNF-α and IL-6 (7). Clearly, excessive production of TBARS and NO contributes to the damage caused by NaAsO2. In this study, according to the obtained results, Cit was able to reduce the toxicity caused by NaAsO2 by decreasing these harmful factors. Remarkably, these findings align with a prior study conducted by Al-Brakati et al. (24).
Overall, Cit exhibited the potential to mitigate the harmful effects of NaAsO2 by targeting TBARS and NO production. According to the histological examination, Cit effectively improved the kidney's pathological conditions caused by NaAsO2 toxicity. On the other hand, NaAsO2 caused significant damage to the renal tissue, including congestion of blood vessels, proximal tube damage, and inflammation. Based on the functional and biochemical findings, it can be concluded that Cit exerted its protective effects by acting as an antioxidant and anti-inflammatory agent.
5.1. Conclusions
Citicoline, known for its strong antioxidant properties, has shown promising results in reducing the damage caused by NaAsO2. The results of our research indicate that citicoline was able to reduce the damage caused by NaAsO2, suggesting that Cit can be used to control and prevent chronic poisoning caused by NaAsO2.
References
-
1.
Khosravi-Darani K, Rehman Y, Katsoyiannis I, Kokkinos E, Zouboulis A. Arsenic Exposure via Contaminated Water and Food Sources. Water. 2022;14(12). https://doi.org/10.3390/w14121884.
-
2.
Gora RH, Kerketta P, Baxla SL, Toppo R, Prasad R, Patra PH, et al. Ameliorative Effect of Tephrosia Purpurea in Arsenic-induced Nephrotoxicity in Rats. Toxicol Int. 2014;21(1):78-83. [PubMed ID: 24748739]. [PubMed Central ID: PMC3989919]. https://doi.org/10.4103/0971-6580.128807.
-
3.
Argos M, Kalra T, Rathouz PJ, Chen Y, Pierce B, Parvez F, et al. Arsenic exposure from drinking water, and all-cause and chronic-disease mortalities in Bangladesh (HEALS): a prospective cohort study. Lancet. 2010;376(9737):252-8. [PubMed ID: 20646756]. [PubMed Central ID: PMC3951449]. https://doi.org/10.1016/S0140-6736(10)60481-3.
-
4.
Steinmaus CM, Ferreccio C, Romo JA, Yuan Y, Cortes S, Marshall G, et al. Drinking water arsenic in northern chile: high cancer risks 40 years after exposure cessation. Cancer Epidemiol Biomarkers Prev. 2013;22(4):623-30. [PubMed ID: 23355602]. [PubMed Central ID: PMC3848421]. https://doi.org/10.1158/1055-9965.EPI-12-1190.
-
5.
S. Herath HMA, Kawakami T, Nagasawa S, Serikawa Y, Motoyama A, Chaminda GGT, et al. Arsenic, cadmium, lead, and chromium in well water, rice, and human urine in Sri Lanka in relation to chronic kidney disease of unknown etiology. J Water Health. 2018;16(2):212-22. [PubMed ID: 29676757]. https://doi.org/10.2166/wh.2018.070.
-
6.
Saint-Jacques N, Brown P, Nauta L, Boxall J, Parker L, Dummer TJB. Estimating the risk of bladder and kidney cancer from exposure to low-levels of arsenic in drinking water, Nova Scotia, Canada. Environ Int. 2018;110:95-104. [PubMed ID: 29089168]. https://doi.org/10.1016/j.envint.2017.10.014.
-
7.
Nikravesh M, Mahdavinia M, Neisi N, Khorsandi L, Khodayar MJ. Citicoline ameliorates arsenic-induced hepatotoxicity and diabetes in mice by overexpression of VAMP2, PPAR-gamma, As3MT, and SIRT3. Pestic Biochem Physiol. 2023;192:105391. [PubMed ID: 37105618]. https://doi.org/10.1016/j.pestbp.2023.105391.
-
8.
Tam LM, Wang Y. Arsenic Exposure and Compromised Protein Quality Control. Chem Res Toxicol. 2020;33(7):1594-604. [PubMed ID: 32410444]. [PubMed Central ID: PMC7373670]. https://doi.org/10.1021/acs.chemrestox.0c00107.
-
9.
Barai M, Ahsan N, Paul N, Hossain K, Rashid MA, Kato M, et al. Amelioration of arsenic-induced toxic effects in mice by dietary supplementation of Syzygium cumini leaf extract. Nagoya journal of medical science. 2017;79(2):167.
-
10.
Jambou R, El-Assaad F, Combes V, Grau GE. Citicoline (CDP-choline): What role in the treatment of complications of infectious diseases. Int J Biochem Cell Biol. 2009;41(7):1467-70. [PubMed ID: 19401146]. https://doi.org/10.1016/j.biocel.2009.02.011.
-
11.
EFSA Panel on Dietetic Products N. Scientific Opinion on the safety of “citicoline” as a Novel Food ingredient. EFSA J. 2013;11(10). https://doi.org/10.2903/j.efsa.2013.3421.
-
12.
Cakir A, Ocalan B, Cansu C, Suyen GG, Cansev M, Kahveci N. Effects of citicoline administration on synaptic proteins in rapid eye movement sleep-deprived rats. Iran J Basic Med Sci. 2022;25(5):562.
-
13.
Safavi M, Hosseini-Sharifabad A, Seyed-Yousefi Y, Rabbani M. Protective Effects of Citicoline and Benfotiamine Each Alone and in Combination on Streptozotocin-induced Memory Impairment in Mice. Clin Psychopharmacol Neurosci. 2020;18(1):81-92. [PubMed ID: 31958909]. [PubMed Central ID: PMC7006985]. https://doi.org/10.9758/cpn.2020.18.1.81.
-
14.
Paul DS, Hernandez-Zavala A, Walton FS, Adair BM, Dedina J, Matousek T, et al. Examination of the effects of arsenic on glucose homeostasis in cell culture and animal studies: development of a mouse model for arsenic-induced diabetes. Toxicol Appl Pharmacol. 2007;222(3):305-14. [PubMed ID: 17336358]. [PubMed Central ID: PMC2680915]. https://doi.org/10.1016/j.taap.2007.01.010.
-
15.
Ellman GL. Tissue sulfhydryl groups. Arch Biochem Biophys. 1959;82(1):70-7. [PubMed ID: 13650640]. https://doi.org/10.1016/0003-9861(59)90090-6.
-
16.
Mihara M, Uchiyama M. Determination of malonaldehyde precursor in tissues by thiobarbituric acid test. Anal Biochem. 1978;86(1):271-8. [PubMed ID: 655387]. https://doi.org/10.1016/0003-2697(78)90342-1.
-
17.
Shangari N, O'Brien PJ. Catalase activity assays. Curr Protoc Toxicol. 2006;Chapter 7:Unit 7 7 1-15. [PubMed ID: 20954160]. https://doi.org/10.1002/0471140856.tx0707s27.
-
18.
Sharma AK, Kaur J, Kaur T, Singh B, Yadav HN, Pathak D, et al. Ameliorative role of bosentan, an endothelin receptor antagonist, against sodium arsenite-induced renal dysfunction in rats. Environ Sci Pollut Res Int. 2021;28(6):7180-90. [PubMed ID: 33026618]. https://doi.org/10.1007/s11356-020-11035-0.
-
19.
Mehrzadi S, Goudarzi M, Fatemi I, Basir Z, Malayeri A, Khalili H. Chrysin attenuates sodium arsenite-induced nephrotoxicity in rats by suppressing oxidative stress and inflammation. Tissue Cell. 2021;73:101657. [PubMed ID: 34628213]. https://doi.org/10.1016/j.tice.2021.101657.
-
20.
Fatemi I, Khalili H, Mehrzadi S, Basir Z, Malayeri A, Goudarzi M. Mechanisms involved in the possible protective effect of chrysin against sodium arsenite-induced liver toxicity in rats. Life Sci. 2021;267:118965. [PubMed ID: 33383050]. https://doi.org/10.1016/j.lfs.2020.118965.
-
21.
Rajak C, Singh N, Parashar P. Metal toxicity and natural antidotes: prevention is better than cure. Environ Sci Pollut Res Int. 2020;27(35):43582-98. [PubMed ID: 32951168]. https://doi.org/10.1007/s11356-020-10783-3.
-
22.
Kandemir FM, Yildirim S, Kucukler S, Caglayan C, Mahamadu A, Dortbudak MB. Therapeutic efficacy of zingerone against vancomycin-induced oxidative stress, inflammation, apoptosis and aquaporin 1 permeability in rat kidney. Biomed Pharmacother. 2018;105:981-91. [PubMed ID: 30021393]. https://doi.org/10.1016/j.biopha.2018.06.048.
-
23.
Akaras N, Gur C, Kucukler S, Kandemir FM. Zingerone reduces sodium arsenite-induced nephrotoxicity by regulating oxidative stress, inflammation, apoptosis and histopathological changes. Chem Biol Interact. 2023;374:110410. [PubMed ID: 36822304]. https://doi.org/10.1016/j.cbi.2023.110410.
-
24.
Al-Brakati AY, Kassab RB, Lokman MS, Elmahallawy EK, Amin HK, Abdel Moneim AE. Role of thymoquinone and ebselen in the prevention of sodium arsenite-induced nephrotoxicity in female rats. Hum Exp Toxicol. 2019;38(4):482-93. [PubMed ID: 30558456]. https://doi.org/10.1177/0960327118818246.
-
25.
Al-Hafyan S, Asoodeh A, Baghshani H, Salari LE. Ameliorative potential of betaine against arsenite-induced hepatotoxicity and nephrotoxicity. Comparative Clinical Pathology. 2023;33(1):155-62. https://doi.org/10.1007/s00580-023-03535-5.
-
26.
Turk E, Kandemir FM, Yildirim S, Caglayan C, Kucukler S, Kuzu M. Protective Effect of Hesperidin on Sodium Arsenite-Induced Nephrotoxicity and Hepatotoxicity in Rats. Biol Trace Elem Res. 2019;189(1):95-108. [PubMed ID: 30066062]. https://doi.org/10.1007/s12011-018-1443-6.
-
27.
Satoh M, Nakamura M, Tamura G, Makita S, Segawa I, Tashiro A, et al. Inducible nitric oxide synthase and tumor necrosis factor-alpha in myocardium in human dilated cardiomyopathy. J Am Coll Cardiol. 1997;29(4):716-24. [PubMed ID: 9091515]. https://doi.org/10.1016/s0735-1097(96)00567-0.
-
28.
Zhang J, Pan X, Li N, Li X, Wang Y, Liu X, et al. Grape seed extract attenuates arsenic-induced nephrotoxicity in rats. Exp Ther Med. 2014;7(1):260-6. [PubMed ID: 24348802]. [PubMed Central ID: PMC3861237]. https://doi.org/10.3892/etm.2013.1381.