Abstract
Background:
Many studies have revealed various aspects of the relationship between the stomach and the brain, but there has been no systematic study of the role of gut microbiota in drug addiction to date. This investigation is based on the novel concept that gut dysbiosis plays an important role in addiction disorders. Probiotic supplements (PBS) are thought to be a useful therapy method for opiate addiction and substance misuse. Therefore, it is postulated that changes in gut flora can play a significant part in the addiction process.Objectives:
The aim of this study was to observe whether PBS, through the gut-brain axis in drug addiction, can decrease morphine (Mor) tendency in rats by affecting oxidative stress (OS) markers, using a conditioned place preference (CPP) paradigm.Methods:
Forty-two rats (weight 180 - 200 g) were divided into six groups (n = 7 in each). The effective dose of morphine (Mor) was 7.5 mg/kg. Two probiotic supplements were used: Prodigest (Prd) containing Lactobacillus acidophilus, Bifidobacterium bifidum, and Bifidobacterium longum, and Prokid (Prk) containing Lactobacillus acidophilus, Bifidobacterium lactis, Lactobacillus rhamnosus, and Bifidobacterium bifidum. These were administered via gavage for 14 days. The animals were given PBS after each conditioning session in the conditioned place preference (CPP) model. The animals' locomotor activity was then evaluated using an open field apparatus. The brain was tested for malondialdehyde (MDA), nitric oxide (NO), catalase (CAT), superoxide dismutase (SOD), total antioxidant capacity (TAC), and thiol levels.Results:
Only the Prd group, but not the Prk group, significantly increased the time spent in the Mor chamber (the location preference index) during the acquisition phase (P < 0.05). The anti-oxidation/oxidation profiles (MDA, NO, CAT, SOD, TAC, and thiol) were altered by PBS.Conclusions:
Given the elevated location preference index generated by Mor, it may be argued that PBS influences mood and behavior via antioxidative/oxidative signaling, thereby potentiating the effect of Mor.Keywords
Conditioned Place Preference Morphine Probiotic Antioxidation/Oxidation Profiles Rat
1. Background
Addiction, in general, accounts for a high annual mortality rate worldwide, surpassing even chronic diseases (1). Despite extensive efforts, there is no treatment that is completely effective and appropriate for this phenomenon (2).
The term "microbiome" refers to a group of microbes, including eubacteria, archaebacteria, fungi, and viruses, that inhabit specific niches together. The study of the microbiome-host relationship has shed light on treating some gastrointestinal disorders (3).
A significant body of research has revealed facts about the connection between the gut and brain, known as the gut-brain axis, which may have a direct effect on brain metabolism (4-6). There is evidence to suggest that the gut-brain axis plays a role in dopamine function. These findings demonstrate the connection between gut microbiota and dopamine function, which could also be considered in the case of addiction (7-9).
Opioid drugs, such as morphine (Mor), may contribute to the development of oxidative stress (OS). They can either promote the formation of free radicals, including reactive oxygen species (ROS) and reactive nitrogen species (RNS), or reduce the activity of various components of antioxidant systems in brain cells (10, 11).
There is evidence that probiotic use can affect metabolic profiles, inflammatory biomarkers, and oxidative stress by producing short-chain fatty acids (SCFA) (12). For example, it has been reported that probiotics can prevent tissue damage by increasing antioxidant activity and decreasing the production of free radicals (13, 14).
Understanding the host-microbiome relationship and nervous system diseases has revolutionized the physiopathological basis of such diseases (15, 16)). A hypothesis states that “changes in the gastrointestinal microbiomes can play a key role in the addiction process” (17). For instance, research by Ning et al. reported that methamphetamine administration altered the gastrointestinal microbial population (18). Therefore, gastrointestinal microbiomes may be considered a new method to address addiction.
2. Objectives
The goal of the present study was to determine whether probiotic supplements (PBS) can decrease morphine (Mor) tendency in rats through the gut-brain axis by affecting oxidative stress (OS) markers, using a conditioned place preference (CPP) paradigm.
3. Methods
3.1. Animals
All studies were conducted on male Wistar rats weighing 180 - 200 g at the start of the experiment, procured from Kashan University of Medical Sciences. The rats were housed in an animal facility on a regular 12-hour light/dark cycle, with free access to food and water. The laboratory temperature was maintained between 22 - 25°C with 40 - 50% humidity. Seven rats were utilized in each study group.
3.2. Drugs
The Mor sulfate was supplied by Temad Co. (Tehran, Iran). The Mor (7.5 mg/kg) was prepared for subcutaneous injection by dissolving it in 0.9% saline (Sal). The 7.5 mg/kg dose elicited CPP effectively, while other doses (2.5, 5, and 10 mg/kg) had a weaker effect. Therefore, we selected 7.5 mg/kg as the effective dose (19).
3.3. Apparatus
The CPP apparatus (Maze Router, Tabriz, Iran) consists of three rooms (A, B, and C) divided by guillotine doors. The two larger conditioning chambers (A and B) are the same size but have different colors and patterns. Chamber A has black and white walls and a corresponding floor, while Chamber B has white walls. Chamber C is smaller than the other chambers and connects to both Chambers A and B through the guillotine doors.
3.4. Behavioral Testing
The CPP protocol was followed for 16 days and comprised three separate phases (18): Pre-conditioning, conditioning, and post-conditioning. All animals were allowed to become accustomed to the device a week before the experiment began. After habituation, animals were placed in the center chamber for 15 minutes while the guillotine doors were raised to provide access to the entire apparatus during the pre-conditioning phase (day 1). The amount of time spent in each chamber was recorded separately during this phase.
During the conditioning phase (days 2 to 15), each rat received 14 days of Mor (7.5 mg/kg, i.p.) or Sal (2 mL/kg, i.p.) injections. Specifically, rats were given Mor on days 2, 4, 6, 8, 10, 12, and 14, and Sal on days 3, 5, 7, 9, 11, 13, and 15. In the 'unbiased' technique, rats were restricted to the morphine compartment for 45 minutes following Mor delivery and to the Sal compartment after Sal injection.
A CPP test was administered during the post-conditioning phase (day 16). Animals were placed in the middle chamber while the guillotine doors were removed, giving them unrestricted access to the entire apparatus for 15 minutes, just like on day one. The time spent in each chamber was recorded at this phase as well. The preference shift was determined as the difference (in seconds) between the times spent in the Mor-receiving chamber on days 16 and 1 (20).
3.5. Preparation and Administration of the Probiotics
In this study, the PBS contained a mixture of bacteria in the form of two products: (1) prodigest (Prd), which contains Lactobacillus acidophilus, Bifidobacterium bifidum, and Bifidobacterium longum (109 CFU/mL each); and (2) prokid (Prk), which contains Lactobacillus acidophilus and Bifidobacterium lactis. Each capsule included 500 mg of the bacterial mixture, totaling 109 CFU. The probiotics were dissolved in water and delivered intragastrically.
3.6. Experimental Design
In this study, a total of 42 rats (n = 7 per group) were randomly assigned to six groups (Sal, Mor, two treatment, and two vehicle groups) and orally gavaged as follows:
- Group I (Sal) received Sal (days 2 - 15) for each conditioning session for 14 consecutive days.
- Group II (Mor) received Mor (days 2, 4, 6, 8, 10, 12, and 14) and Sal (days 3, 5, 7, 9, 11, 13, and 15) for each conditioning session.
- Group III (Mor+Prd) received Mor (days 2, 4, 6, 8, 10, 12, and 14) and Sal (days 3, 5, 7, 9, 11, 13, and 15) for each conditioning session and was given the Prd product following each conditioning session.
- Group IV (Sal+Prd) received Sal (days 2 - 15) for each conditioning session and was given the Prd product following each conditioning session.
- Group V (Mor+Prk) received Mor (days 2, 4, 6, 8, 10, 12, and 14) and Sal (days 3, 5, 7, 9, 11, 13, and 15) for each conditioning session and was given the Prk product following each conditioning session.
- Group VI (Sal+Prk) received Sal (days 2-15) for each conditioning session and was given the Prk product following each conditioning session.
Each group received their respective treatments for 14 consecutive days (Figure 1).
Experimental procedure
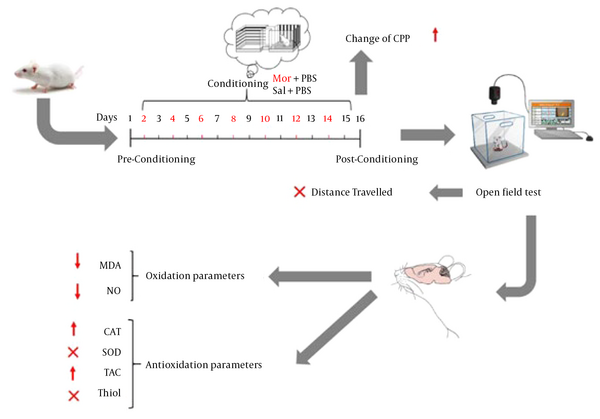
3.7. Oxidation Profiles
Malondialdehyde (MDA) levels in brain tissue were determined using the method developed by Ohkawa et al. (21) to evaluate lipid peroxidation (LPO) in terms of nmol/mg protein (19). The nitric oxide (NO) level in brain tissue was evaluated in nmol/mg protein by detecting supernatant metabolites with the Griess reagent, as proposed by Moshage et al. (22).
3.8. Antioxidant Profiles
The activities of catalase (CAT), superoxide dismutase (SOD), total antioxidant capacity (TAC), and thiol were evaluated using ELISA kits (Kiazist Co., Iran).
3.9. Total Protein
The Bradford method was used to measure total protein levels in brain tissue, utilizing concentrated Coomassie blue reagent and bovine serum albumin as the standard (23).
3.10. Statistical Analysis
The results are presented as mean ± SEM. To analyze the data, one-way ANOVA with a Tukey post-test was utilized. Calculations were performed using the Prism statistical program version 9.
4. Results
Figure 2A depicts the effect of different Mor dosages on CPP in all groups. Mor dosages of 2.5, 5, 7.5, and 10 mg/kg were employed in the current investigation. The results showed a substantial increase in CPP at dosages of 5, 7.5, and 10 mg/kg (particularly 7.5 mg/kg) compared to the Sal group (ANOVA, Tukey's: P < 0.01). As a result, 7.5 mg/kg of Mor was deemed effective in this trial.
Figure 2B depicts the effect of different PBS treatments on CPP in all groups. The (Mor+Prd) group showed a substantial increase in the change of CPP (Sec.) compared to the Mor group (P < 0.01).
Figure 2C shows that there is no significant influence on locomotor activity between the groups (P > 0.05).
The effects of different doses of Mor. administration on CPP for determining the effective dose of Mor (A), the effect of treatment with probiotic supplement (Prodijest and Prokid products) on the CPP in all groups (B), the effect of treatment with probiotic supplement (Prodijest and Prokid products) on locomotor activity in all groups (C). Comparisons between different groups were made using one-way analysis of variance (ANOVA) followed by Tukey’s post hoc test. Data are expressed as mean ± SEM (n=7 in each group). * P < 0.05, ** P < 0.01, and *** P < 0.001, compared to Sal group. ++ P < 0.01 compared to Mor group. Sal, Saline; Mor, Morphine; Prd, Prodijest; Prk, Prokid; CPP, Conditioned place preference.
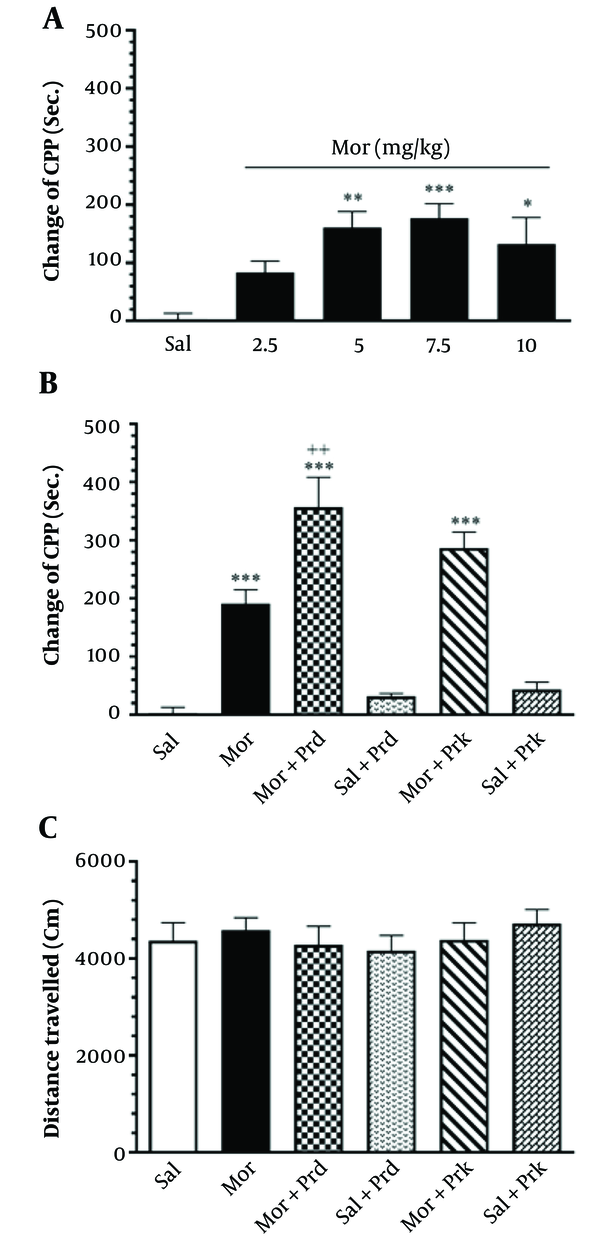
Figure 3A depicts the effect of different PBS treatments on LPO in all experimental groups. The MDA level in brain tissue increased significantly in the Mor group compared to the Sal group (P < 0.001). The MDA levels in brain tissue were significantly lower in the (Mor+Prd) and (Mor+Prk) groups compared to the Mor group (P < 0.05).
Figure 3B depicts the effect of different PBS treatments on NO in all experimental groups. The Mor group showed a significant rise in NO levels in brain tissue compared to the Sal group (P < 0.001). The (Mor+Prd) group had a significant decrease in NO levels in brain tissue compared to the Mor group (P < 0.05).
The level of MDA (nmol/mg protein) (A) and NO (nmol/mg protein) (B) in brain tissue after treatment with probiotic supplement in all groups. Comparisons between different groups were made using one-way analysis of variance (ANOVA) followed by Tukey’s post hoc test. Data are expressed as mean ± SEM (n = 7 in each group). ** P < 0.01, and *** P < 0.001 compared to Sal group. + P < 0.05, ++ P < 0.01, and +++ P < 0.001 compared to Mor group. Sal, Saline; Mor, Morphine; Prd, Prodigest; Prk, Prokid; MDA, malondialdehyde; NO, nitric oxide.
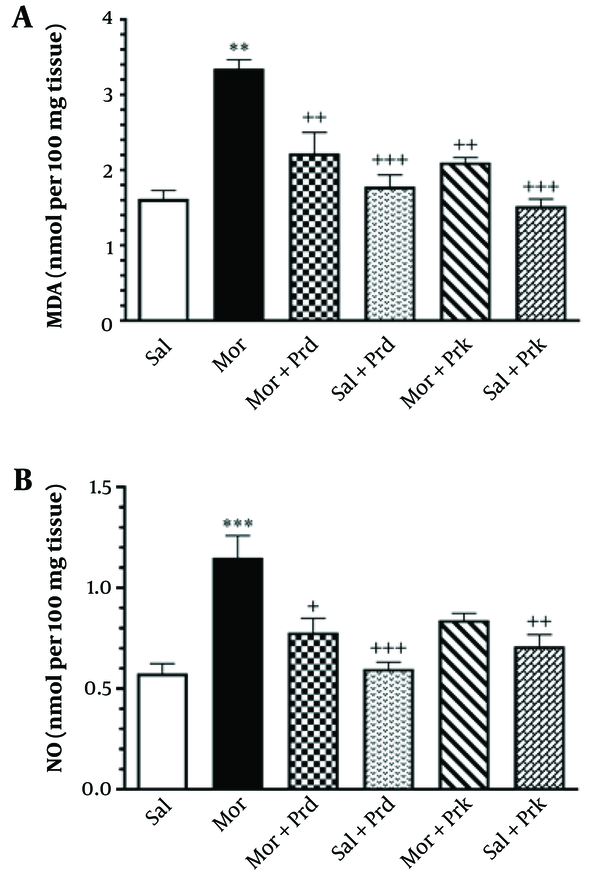
Figure 4A depicts the effect of different PBS treatments on the tissue level activity of CAT (U/M) in all groups. The Mor group showed a significant decrease in CAT activity in brain tissue compared to the Sal group (P < 0.05). Additionally, the (Mor+Prd) and (Mor+Prk) groups showed a significant increase in CAT activity in brain tissue compared to the Mor group (P < 0.001).
Figure 4B depicts the effect of different PBS treatments on the tissue activity of SOD (U/M) in all groups. The Mor group showed a significant decrease in SOD activity in brain tissue compared to the Sal group (P < 0.05). However, there was no significant difference in SOD activity in brain tissue between the (Mor+Prd) and (Mor+Prk) groups compared to the Mor group (P > 0.05).
The activity of CAT (U/M) (A) and SOD (U/M) (B) in brain tissue after treatment with probiotic supplement in all groups. Comparisons between different groups were made using one-way analysis of variance (ANOVA) followed by Tukey’s post hoc test. Data are expressed as mean ± SEM (n = 7 in each group). * P < 0.05, ** P < 0.01, and *** P < 0.001 compared to Sal group. + P < 0.05, and +++ P < 0.001 compared to Mor group. Sal, Saline; Mor, Morphine; Prd, Prodigest; Prk, Prokid; CAT, catalase; SOD, superoxide dismutase.
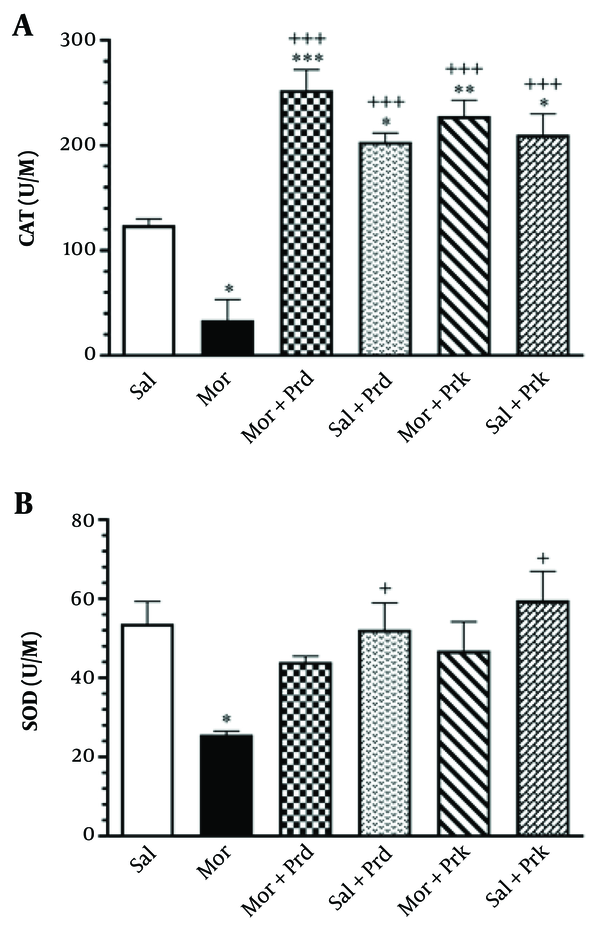
Figure 5A depicts the effect of different PBS treatments on TAC (nmol/mg protein) in all experimental groups. TAC levels in brain tissue were significantly lower in the Mor group compared to the Sal group (P < 0.001). Additionally, TAC levels in brain tissue were significantly increased in the (Mor+Prd) group (P < 0.01) and the (Mor+Prk) group (P < 0.001) compared to the Mor group.
Figure 5B depicts the effect of different PBS treatments on thiol (nmol/mg protein) in all experimental groups. Thiol levels in brain tissue were not significantly different in the Mor group compared to the Sal group (P > 0.05). Other groups showed no significant difference in thiol levels in brain tissue compared to the Mor group (P > 0.05).
The level of TAC (nmol/mg protein) (A) and Thiol (nmol/mg protein) (B) in brain tissue after treatment with probiotic supplement in all groups. Comparisons between different groups were made using one-way analysis of variance (ANOVA) followed by Tukey’s post hoc test. Data are expressed as mean ± SEM (n = 7 in each group). * P < 0.05, and *** P < 0.01 compared to Sal group. ++ P < 0.01, and +++ P < 0.001 compared to Mor group. Sal, Saline; Mor, Morphine; Prd, Prodijest; Prk, Prokid; TAC, total antioxidant capacity.
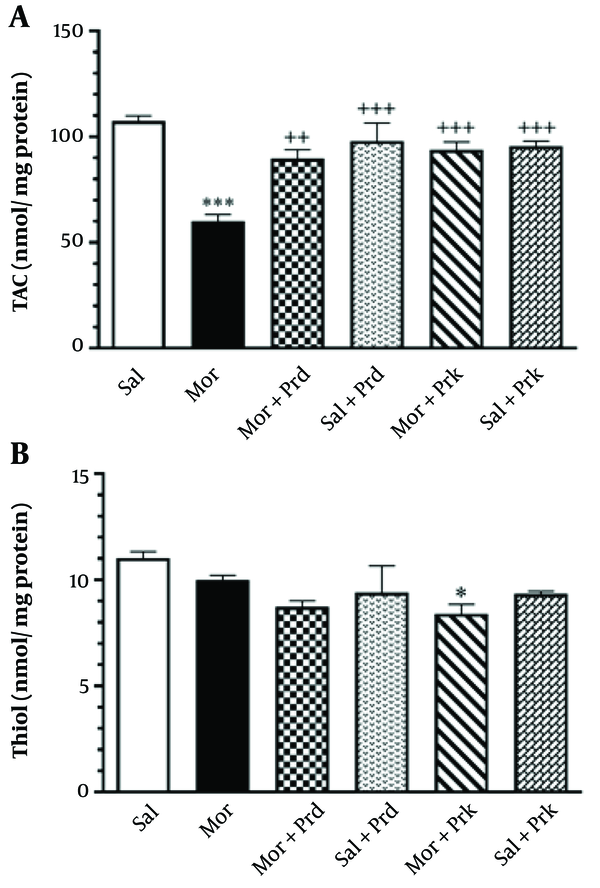
5. Discussion
Our findings revealed that Mor (7.5 mg/kg) administration significantly increased CPP compared to the Sal group. When compared to the Mor group, the probiotic treatment (Mor+Prd) significantly raised the CPP Index. There was no significant difference in locomotor activity among all groups in the open field test.
There is evidence that the gut-brain axis plays a role in dopamine function (7, 8). These findings suggest a link between gut microbiota and dopamine function, which could be useful in treating addictive disorders (9).
Furthermore, microbes have been shown to release SCFAs, which regulate G-protein-coupled receptors to mediate hormone release and neurotransmitter release (i.e., serotonin, dopamine, noradrenaline, γ-aminobutyric acid (GABA), acetylcholine, and histamine), as well as regulate inflammation, mood, and behavior (24). As a result, it is possible that PBS increased the preference index by enhancing dopamine release in the brain's reward system.
CPP is one of the most widely used models for assessing the mechanisms of drug abuse. One significant advantage of this method is its ability to identify both reinforcing and aversive effects of a substance. It is also useful in the investigation of addictive and potentially addictive medicines (19). The rewarding quality of a drug is determined during the paradigm by its ability to induce a preference for an environment in which the drug has been repeatedly administered (25). In the current investigation, a 16-day CPP procedure was employed based on the results provided by Ning (18).
Several studies have highlighted the connection between the gut and brain, but to date, there has been no systematic study of the involvement of gut microbiota in drug addiction (4, 5). The gut-brain axis, or the relationship between the gut and the brain, may have a direct effect on brain metabolism (6). According to this research, the influence of gut microbiota on the human endogenous opioid system may be involved in various areas, such as endogenous opioids, opioid medications, and opioid receptors that generate effects in the CNS (26).
On the other hand, PBS-based experimental and clinical research indicates improvements in brain function. For example, Fuenzalida et al. reported that probiotics-based treatment could be an attractive intervention to attenuate alcohol-induced behaviors and retard disease progression by repairing the gut microbiota and augmenting microbiota-gut-liver-brain axis communication (27).
Measurement of free radical or ROS generation, LPO metabolites such as MDA, and antioxidant capacity may provide insight into antioxidant homeostasis under Mor stimulation and Mor-induced toxicity. The brain is extremely sensitive to peroxidative damage because it has a comparatively high amount of unsaturated fatty acids relative to its level of antioxidants and consumes more oxygen. Furthermore, a large body of research suggests that OS is involved in the development of addiction to various addictive drugs, including cocaine, methamphetamine, and Mor (11, 28). Mor can also be converted into free radicals, and an excess of ROS can cause oxidative damage (11), which should be considered in the therapy of opioid addiction (21).
In the current study, MDA and NO levels in the brain were higher in the Mor group than in the Sal group. However, PBS (particularly the Prd product) significantly reduced MDA and NO levels. These findings suggest PBS's antioxidant action and potential tissue protection against lipid oxidation and inflammatory damage (29). Furthermore, a recent study reveals that the SLAB51 multi-strain probiotic formulation can lower NO generation in intestinal cells, leading to the conclusion that decreased oxygen consumption by intestinal cells implies greater oxygen delivery to other organs, including the brain (30). Other studies have found that PBS has protective effects by improving antioxidant defense (29). Many studies have shown that different probiotic bacteria strains can exert antioxidant capacity in various ways, including stimulating the antioxidant system, increasing antioxidant activity, and producing a variety of metabolites with antioxidant activity (29). This is supported by an increase in CAT activity following PBS therapy in this investigation.
It has also been observed that PBS treatment resulted in a considerable increase in plasma TAC levels in Alzheimer's rats (31). Our findings also showed that PBS (particularly the Prk product) significantly boosted TAC levels compared to the Mor group. However, none of the PBS had a substantial influence on thiol levels.
This study also demonstrated that the use of Mor resulted in a significant drop in brain activity levels of antioxidant enzymes such as CAT and SOD when compared to the Sal group. Previous research has shown that Mor reduces the antioxidative defense system (32). OS is thought to be one of the most critical mechanisms underlying drug-induced decreases in CAT and SOD activity in rats (11). CAT's cellular antioxidant defense involves digesting hydrogen peroxide, which prevents the Fenton reaction from producing hydroxyl radicals. Superoxide is one of the most prevalent ROS produced by mitochondria. SOD catalyzes the breakdown of superoxide into hydrogen peroxide and water, making it a key regulator of ROS levels (29).
Our findings in this study also showed that PBS caused a considerable increase in CAT but not SOD activity in the brain compared to the Mor group. There is a chance that increasing the length of PBS treatment will boost SOD activity in the brain. Although SOD's antioxidant action is well recognized, its therapeutic applicability is limited due to its short circulatory half-life, which limits its bioavailability. As a result, it is possible that SOD activity in the PBS-treated groups was initially high but eventually dropped.
To highlight a shortcoming, our method for measuring total NO was indirect. We propose further investigations that use direct and precise methods for NO assaying, such as electron paramagnetic resonance spectroscopy (33).
5.1. Conclusions
To summarize, given the higher CPP index caused by Mor, it can be argued that PBS interacts with the Mor effect by releasing SCFA that regulate G-protein-coupled receptors to modulate hormone and neurotransmitter release, such as dopamine, which governs mood and behavior. Furthermore, the biochemical findings of this study imply that PBS has advantageous antioxidative/oxidative profiles. These findings suggest that PBS can be clinically examined as a therapeutic option for drug addiction. Nonetheless, further animal and human investigations are recommended to corroborate these findings.
References
-
1.
Cheron J, Kerchove d'Exaerde A. Drug addiction: from bench to bedside. Transl Psychiatry. 2021;11(1):424. [PubMed ID: 34385417]. [PubMed Central ID: PMC8361217]. https://doi.org/10.1038/s41398-021-01542-0.
-
2.
Cami J, Farre M. Drug addiction. N Engl J Med. 2003;349(10):975-86. [PubMed ID: 12954747]. https://doi.org/10.1056/NEJMra023160.
-
3.
Garcia-Cabrerizo R, Carbia C, O. Riordan KJ, Schellekens H, Cryan JF. Microbiota-gut-brain axis as a regulator of reward processes. J Neurochem. 2021;157(5):1495-524. [PubMed ID: 33368280]. https://doi.org/10.1111/jnc.15284.
-
4.
Erny D, Hrabe de Angelis AL, Jaitin D, Wieghofer P, Staszewski O, David E, et al. Host microbiota constantly control maturation and function of microglia in the CNS. Nat Neurosci. 2015;18(7):965-77. [PubMed ID: 26030851]. [PubMed Central ID: PMC5528863]. https://doi.org/10.1038/nn.4030.
-
5.
Parashar A, Udayabanu M. Gut microbiota regulates key modulators of social behavior. Eur Neuropsychopharmacol. 2016;26(1):78-91. [PubMed ID: 26613639]. https://doi.org/10.1016/j.euroneuro.2015.11.002.
-
6.
Kelly JR, Kennedy PJ, Cryan JF, Dinan TG, Clarke G, Hyland NP. Breaking down the barriers: the gut microbiome, intestinal permeability and stress-related psychiatric disorders. Front Cell Neurosci. 2015;9:392. [PubMed ID: 26528128]. [PubMed Central ID: PMC4604320]. https://doi.org/10.3389/fncel.2015.00392.
-
7.
Cersosimo MG, Benarroch EE. Pathological correlates of gastrointestinal dysfunction in Parkinson's disease. Neurobiol Dis. 2012;46(3):559-64. [PubMed ID: 22048068]. https://doi.org/10.1016/j.nbd.2011.10.014.
-
8.
Cersosimo MG, Raina GB, Pecci C, Pellene A, Calandra CR, Gutierrez C, et al. Gastrointestinal manifestations in Parkinson's disease: prevalence and occurrence before motor symptoms. J Neurol. 2013;260(5):1332-8. [PubMed ID: 23263478]. https://doi.org/10.1007/s00415-012-6801-2.
-
9.
Severance EG, Yolken RH, Eaton WW. Autoimmune diseases, gastrointestinal disorders and the microbiome in schizophrenia: more than a gut feeling. Schizophr Res. 2016;176(1):23-35. [PubMed ID: 25034760]. [PubMed Central ID: PMC4294997]. https://doi.org/10.1016/j.schres.2014.06.027.
-
10.
Skrabalova J, Drastichova Z, Novotny J. Morphine as a Potential Oxidative Stress-Causing Agent. Mini Rev Org Chem. 2013;10(4):367-72. [PubMed ID: 24376392]. [PubMed Central ID: PMC3871421]. https://doi.org/10.2174/1570193X113106660031.
-
11.
Zeng XS, Geng WS, Wang ZQ, Jia JJ. Morphine Addiction and Oxidative Stress: The Potential Effects of Thioredoxin-1. Front Pharmacol. 2020;11:82. [PubMed ID: 32153403]. [PubMed Central ID: PMC7047156]. https://doi.org/10.3389/fphar.2020.00082.
-
12.
Voltolini C, Battersby S, Etherington SL, Petraglia F, Norman JE, Jabbour HN. A novel antiinflammatory role for the short-chain fatty acids in human labor. Endocrinology. 2012;153(1):395-403. [PubMed ID: 22186417]. https://doi.org/10.1210/en.2011-1457.
-
13.
Abhari K, Shekarforoush SS, Hosseinzadeh S, Nazifi S, Sajedianfard J, Eskandari MH. The effects of orally administered Bacillus coagulans and inulin on prevention and progression of rheumatoid arthritis in rats. Food Nutr Res. 2016;60:30876. [PubMed ID: 27427194]. [PubMed Central ID: PMC4947834]. https://doi.org/10.3402/fnr.v60.30876.
-
14.
Divyashri G, Krishna G, Prapulla SG; Muralidhara. Probiotic attributes, antioxidant, anti-inflammatory and neuromodulatory effects of Enterococcus faecium CFR 3003: in vitro and in vivo evidence. J Med Microbiol. 2015;64(12):1527-40. [PubMed ID: 26450608]. https://doi.org/10.1099/jmm.0.000184.
-
15.
Ait-Belgnaoui A, Colom A, Braniste V, Ramalho L, Marrot A, Cartier C, et al. Probiotic gut effect prevents the chronic psychological stress-induced brain activity abnormality in mice. Neurogastroenterol Motil. 2014;26(4):510-20. [PubMed ID: 24372793]. https://doi.org/10.1111/nmo.12295.
-
16.
Sarkar A, Lehto SM, Harty S, Dinan TG, Cryan JF, Burnet PWJ. Psychobiotics and the Manipulation of Bacteria-Gut-Brain Signals. Trends Neurosci. 2016;39(11):763-81. [PubMed ID: 27793434]. [PubMed Central ID: PMC5102282]. https://doi.org/10.1016/j.tins.2016.09.002.
-
17.
Skosnik PD, Cortes-Briones JA. Targeting the ecology within: The role of the gut-brain axis and human microbiota in drug addiction. Med Hypotheses. 2016;93:77-80. [PubMed ID: 27372861]. https://doi.org/10.1016/j.mehy.2016.05.021.
-
18.
Ning T, Gong X, Xie L, Ma B. Gut Microbiota Analysis in Rats with Methamphetamine-Induced Conditioned Place Preference. Front Microbiol. 2017;8:1620. [PubMed ID: 28890714]. [PubMed Central ID: PMC5575146]. https://doi.org/10.3389/fmicb.2017.01620.
-
19.
Saeedfar M, Ardjmand A, Alani B, Ghaderi A, Banafshe HR, Shahaboddin ME, et al. The effect of vitamin D on morphine preference in rats: Possible biochemical and DRD2-GDNF signaling. Brain Behav. 2023;13(2). e2877. [PubMed ID: 36630182]. [PubMed Central ID: PMC9927858]. https://doi.org/10.1002/brb3.2877.
-
20.
Huston JP, Silva MA, Topic B, Muller CP. What's conditioned in conditioned place preference? Trends Pharmacol Sci. 2013;34(3):162-6. [PubMed ID: 23384389]. https://doi.org/10.1016/j.tips.2013.01.004.
-
21.
Ohkawa H, Ohishi N, Yagi K. Assay for lipid peroxides in animal tissues by thiobarbituric acid reaction. Anal Biochem. 1979;95(2):351-8. [PubMed ID: 36810]. https://doi.org/10.1016/0003-2697(79)90738-3.
-
22.
Moshage H, Kok B, Huizenga JR, Jansen PL. Nitrite and nitrate determinations in plasma: a critical evaluation. Clin Chem. 1995;41(6 Pt 1):892-6. [PubMed ID: 7768008].
-
23.
Hammond JB, Kruger NJ. The bradford method for protein quantitation. Methods Mol Biol. 1988;3:25-32. [PubMed ID: 21400151]. https://doi.org/10.1385/0-89603-126-8:25.
-
24.
Panther EJ, Dodd W, Clark A, Lucke-Wold B. Gastrointestinal Microbiome and Neurologic Injury. Biomedicines. 2022;10(2). [PubMed ID: 35203709]. [PubMed Central ID: PMC8962360]. https://doi.org/10.3390/biomedicines10020500.
-
25.
Zhang J, Yang J, Yang C, Chen T, Wang Z, Li J, et al. Sensitivity to Morphine Reward Associates With Gut Dysbiosis in Rats With Morphine-Induced Conditioned Place Preference. Front Psychiatry. 2020;11:631. [PubMed ID: 33005148]. [PubMed Central ID: PMC7484999]. https://doi.org/10.3389/fpsyt.2020.00631.
-
26.
Rueda-Ruzafa L, Cruz F, Cardona D, Hone AJ, Molina-Torres G, Sanchez-Labraca N, et al. Opioid system influences gut-brain axis: Dysbiosis and related alterations. Pharmacol Res. 2020;159:104928. [PubMed ID: 32504837]. https://doi.org/10.1016/j.phrs.2020.104928.
-
27.
Fuenzalida C, Dufeu MS, Poniachik J, Roblero JP, Valenzuela-Perez L, Beltran CJ. Probiotics-Based Treatment as an Integral Approach for Alcohol Use Disorder in Alcoholic Liver Disease. Front Pharmacol. 2021;12:729950. [PubMed ID: 34630107]. [PubMed Central ID: PMC8497569]. https://doi.org/10.3389/fphar.2021.729950.
-
28.
Jang EY, Yang CH, Hedges DM, Kim SP, Lee JY, Ekins TG, et al. The role of reactive oxygen species in methamphetamine self-administration and dopamine release in the nucleus accumbens. Addict Biol. 2017;22(5):1304-15. [PubMed ID: 27417190]. [PubMed Central ID: PMC5237425]. https://doi.org/10.1111/adb.12419.
-
29.
Wang Y, Wu Y, Wang Y, Xu H, Mei X, Yu D, et al. Antioxidant Properties of Probiotic Bacteria. Nutrients. 2017;9(5). [PubMed ID: 28534820]. [PubMed Central ID: PMC5452251]. https://doi.org/10.3390/nu9050521.
-
30.
Ceccarelli G, Marazzato M, Celani L, Lombardi F, Piccirilli A, Mancone M, et al. Oxygen Sparing Effect of Bacteriotherapy in COVID-19. Nutrients. 2021;13(8). [PubMed ID: 34445055]. [PubMed Central ID: PMC8401839]. https://doi.org/10.3390/nu13082898.
-
31.
Rezaei Asl Z, Sepehri G, Salami M. Probiotic treatment improves the impaired spatial cognitive performance and restores synaptic plasticity in an animal model of Alzheimer's disease. Behav Brain Res. 2019;376:112183. [PubMed ID: 31472194]. https://doi.org/10.1016/j.bbr.2019.112183.
-
32.
Motaghinejad M, Karimian M, Motaghinejad O, Shabab B, Yazdani I, Fatima S. Protective effects of various dosage of Curcumin against morphine induced apoptosis and oxidative stress in rat isolated hippocampus. Pharmacol Rep. 2015;67(2):230-5. [PubMed ID: 25712644]. https://doi.org/10.1016/j.pharep.2014.09.006.
-
33.
Kozlov AV, Biagini G, Tomasi A, Zini I. Ex vivo demonstration of nitric oxide in the rat brain: effects of intrastriatal endothelin-1 injection. Neurosci Lett. 1995;196(1-2):140-4. [PubMed ID: 7501245]. https://doi.org/10.1016/0304-3940(95)11822-e.