Abstract
Background:
Paraxanthine is the major metabolite of caffeine in humans. There is no definitive proof that paraxanthine affects seizures. Nitric oxide (NO) contributes to the central effects of paraxanthine.Objectives:
In this study, we examined the effect of acute paraxanthine administration on the pentylenetetrazole (PTZ)-induced seizure threshold, focusing on the NO-cyclic guanosine monophosphate (cGMP) signaling pathway.Methods:
Naval Medical Research Institute (NMRI) male mice (25 - 30 g) received paraxanthine (5, 10, 50, and 100 mg/kg) alone. L-arginine [a substrate for NO synthase (NOS)] (50 mg/kg) or L-NAME [a non-selective NOS inhibitor] (5 mg/kg) was administered alone or as pretreatment before the ineffective or effective doses of paraxanthine, respectively. The intravenous PTZ seizure threshold test was used to determine the thresholds for the onset of myoclonic twitch (MCT), generalized clonus (GNC), and forelimb tonus (FLT). Nitric oxide metabolites (NOx) were measured using the Griess method.Results:
Paraxanthine administered at doses of 10 and 50 mg/kg significantly reduced the threshold for FLT (P < 0.05 for both). At a dose of 100 mg/kg, paraxanthine significantly reduced the thresholds for the onset of MCT (P < 0.001), GNC (P < 0.01), and FLT (P < 0.001). L-arginine (50 mg/kg), either alone or as pretreatment before paraxanthine (5 mg/kg), did not significantly change the seizure threshold. L-NAME (5 mg/kg) alone had no effect, but L-NAME pretreatment before paraxanthine (100 mg/kg) significantly increased the thresholds for the onset of MCT (P < 0.001), GNC (P < 0.001), and FLT (P < 0.001). Only paraxanthine at a dose of 100 mg/kg significantly increased NOx levels in brain tissues (P < 0.05). Pretreatment with L-arginine further increased the NOx level (P < 0.001), whereas pretreatment with L-NAME decreased it (P < 0.001) compared to the paraxanthine groups.Conclusions:
Our results show that paraxanthine has a proconvulsant effect. The results of L-arginine or L-NAME pretreatment before paraxanthine support the possible interaction of the NO-cGMP pathway in the proconvulsant effect of paraxanthine.Keywords
Seizure Paraxanthine Nitric Oxide Pentylenetetrazole L-Arginine L-NAME
1. Background
An epileptic seizure is characterized by abnormal synchronous neuronal activity in the brain, resulting in specific symptoms (1). Epilepsy is a chronic neurological disorder marked by recurrent seizures, affecting approximately 1% of the global population (2). Significant uncertainty remains regarding the mechanisms by which normal brain function transitions into seizure activity. Moreover, about 30% of epilepsy patients are refractory to treatment with antiepileptic drugs (AEDs) (3). Several animal models have been developed to investigate the mechanisms underlying epilepsy, including the pentylenetetrazole (PTZ)-induced seizure model (4). Although natural products or their derivatives are widely used by individuals with epilepsy worldwide, there is a lack of robust scientific evidence supporting their safety or efficacy (5). The intravenous (i.v.) Pentylenetetrazole seizure threshold test is a useful method for evaluating the effects of these products on seizures.
Nitric oxide (NO) is a widely recognized gas that acts as a signaling molecule. It is synthesized from L-arginine by various forms of NO synthase (NOS), including endothelial NOS (eNOS), neuronal NOS (nNOS), and inducible NOS (iNOS) (6). The NO-cyclic guanosine monophosphate (cGMP) pathway regulates emotional, cognitive, and behavioral processes such as learning, depression, anxiety, and seizure activity (7). Its effects on seizures can be either anticonvulsant or proconvulsant, depending on the specific seizure model or method of administration (8). Additionally, activation of the NO pathway significantly contributes to the neuroprotective and anticonvulsant effects of PTZ preconditioning in mice (8).
Paraxanthine (1,7-dimethylxanthine) is a natural dietary ingredient and the primary metabolite of caffeine (1,3,7-trimethylxanthine) in the human body. Caffeine is extensively metabolized in the liver by the cytochrome P450 (CYP450) enzyme system, particularly by the CYP1A2 isoenzyme (9). The major metabolite of caffeine in humans is paraxanthine, which accounts for approximately 80% of the dimethylxanthines (10). In rodents, caffeine is converted into dimethylxanthines, theophylline, paraxanthine, and theobromine in equal amounts (11) (Figure 1).
The metabolism of caffeine
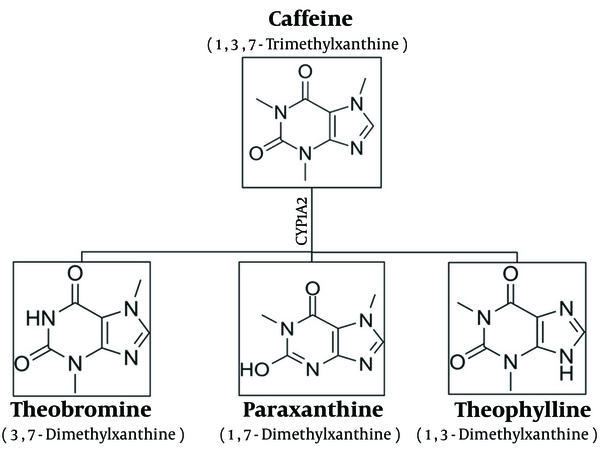
The central effects of paraxanthine are primarily due to its ability to block two subtypes of adenosine receptors (ARs), namely A1 and A2A ARs, similar to caffeine (12). However, paraxanthine is a more potent A1AR and A2AAR antagonist than caffeine (13, 14). Although caffeine and paraxanthine share a common mechanism of action, paraxanthine appears to have a distinct pharmacological profile.
Numerous studies have explored the relationship between caffeine and seizures, yielding conflicting results (15-17). According to our recent findings, a crucial aspect of caffeine's effect on seizures is the modulation of the NO-cGMP pathway (18, 19). The impact of paraxanthine on seizure activity remains largely unexplored, with only one report indicating that long-term treatment decreased susceptibility to PTZ-induced seizures in mice (20). Similar to caffeine, paraxanthine also inhibits A1AR and A2AAR (12). Since activation of the A2AARs increases NO production, while activation of the A1ARs decreases NO production (21), some of the effects of paraxanthine may be due to modulation of the NO pathway. Based on previous studies, paraxanthine exerts a greater effect on locomotion than caffeine due to activation of the NO-cGMP pathway (22). Furthermore, paraxanthine, but not caffeine, potentiates NO signaling through inhibition of cGMP-preferring phosphodiesterase-9 (PDE-9) (9). Overall, it appears that the NO-cGMP pathway plays a significant role in the central effects of paraxanthine.
2. Objectives
This study aimed to investigate the effects of paraxanthine on clonic and tonic seizures using the i.v. PTZ test in mice and to explore the involvement of the NO-cGMP pathway.
3. Methods
3.1. Animals
Male NMRI (Naval Medical Research Institute) mice weighing 25 - 30 g, bred in the animal house of Kashan University of Medical Sciences, were used in this study. The animals were housed in standard polypropylene cages with a 12-hour light-dark cycle, constant temperature (22°C), and humidity (50 - 55%).
3.2. Chemicals
Paraxanthine was purchased from Tocris (UK). Pentylenetetrazole, L-arginine (a NOS substrate), and N(G)-Nitro-L-arginine Methyl Ester (L-NAME), a non-selective NOS inhibitor, were obtained from Sigma Aldrich Corporation (USA). Dimethyl sulfoxide (DMSO) was purchased from Merck (Germany). Pentylenetetrazole solution (5 mg/mL) was prepared in 0.9% sterile saline containing 10 units/mL of heparin. DMSO was used to dissolve paraxanthine according to the manufacturer's recommended protocol. All the described drugs were administered intraperitoneally (i.p.) in a volume of 5 mL per kilogram of body weight.
3.3. Experiments
Based on prior work (23) and pilot studies, male NMRI mice (25 - 30 g) were randomly divided into the following groups (N = 8 per group): (1) two control groups administered saline or DMSO 45 minutes before the i.v. infusion of PTZ; (2) four experimental groups administered paraxanthine at doses of 5, 10, 50, and 100 mg/kg, 45 minutes before the i.v. infusion of PTZ; (3) two experimental groups administered L-arginine (50 mg/kg) or L-NAME (5 mg/kg) alone, 60 minutes before the i.v. infusion of PTZ; (4) two experimental groups pretreated with L-arginine (50 mg/kg) 15 minutes before an ineffective dose of paraxanthine or L-NAME (5 mg/kg) 15 minutes before an effective dose of paraxanthine. Ineffective or effective doses of paraxanthine were administered 45 minutes before the infusion of PTZ.
3.4. The Intravenous Pentylenetetrazole Seizure Threshold Test
In summary, a 30-gauge stainless steel needle attached to a length of No. 10 polyethylene (PE) tubing was used to catheterize the lateral tail vein of each mouse. The tubing was secured to the tail with adhesive tape and attached to a 10 mL plastic syringe containing the PTZ solution (5 mg/mL PTZ in 0.9% saline with 10 units/mL of heparin). This setup was mounted on a syringe pump (Top Corporation, Japan) to infuse the PTZ solution into the tail vein of a freely moving mouse at a constant rate of 0.5 mL/min. The times from the start of the infusion to the onset of myoclonic twitch (MCT), generalized clonus (GNC), and forelimb tonus (FLT) were recorded for each mouse. These times were then converted to mg/kg PTZ for each mouse as follows:
An increase in the mg/kg dose of PTZ required for the onset of MCT, GNC, and FLT indicates that the test substance can raise the seizure threshold. Conversely, a decrease suggests that the substance has a proconvulsant effect (23, 24).
3.5. Brain NO Metabolites (NOx) Measurement
As previously mentioned, the Griess reaction was used to measure NOx in brain tissue (18). First, nitrite standard curves were prepared. The brain tissues were homogenized using a 50 mM Tris-HCl solution containing 0.1 mM ethylenediaminetetraacetic acid (EDTA). Then, 100 µL of the tissue suspension was mixed with the Griess reagent. This reagent includes 100 µL of vanadium (III) chloride (VCl3), 50 µL of sulfanilamide, and 50 µL of N-(1-Naphthyl) ethylenediamine dihydrochloride (NEDD). VCl3 was used to convert nitrate into nitrite. After centrifugation, the supernatants were transferred to flat-bottomed 96-well microplates. Absorbance values were measured using a microplate reader at 540 nm, based on the standard nitrite curves.
3.6. Statistical Analysis
The standard error of the mean (SEM) was calculated using SPSS version 24.0 software. Data on seizures and brain NOx levels were analyzed using one-way analysis of variance (ANOVA) followed by Tukey's multiple comparison test. In all analyses, significance was set at P < 0.05.
4. Results
4.1. Intravenous Pentylenetetrazole Test in Mice Shows that Paraxanthine (5, 10, 50, and 100 mg/kg) Influences Seizure Thresholds
Figure 2 presents the effects of paraxanthine on the seizure threshold in the i.v. PTZ test. A one-way ANOVA comparing the seizure threshold revealed significant effects for multiple seizure types: [F (4,35) = 8.63, P < 0.001 for MCT; F (4,35) = 6.90, P < 0.001 for GNC; F (4,35) = 7.89, P < 0.001 for FLT]. Tukey's post-hoc test revealed that paraxanthine at a dose of 5 mg/kg had no significant effect on any seizure phase. In comparison to the control group treated with a vehicle, paraxanthine at doses of 10 and 50 mg/kg significantly reduced the threshold for the onset of FLT (P < 0.05 for both). Furthermore, paraxanthine at a dose of 100 mg/kg significantly reduced the threshold for the onset of GNC (P < 0.01), MCT (P < 0.001), and FLT (P < 0.001) compared to the control group. Based on these results, paraxanthine at a dose of 100 mg/kg (effective dose) and a dose of 5 mg/kg (ineffective dose) were selected to study the role of the NO-cGMP pathway in the effect of paraxanthine on seizures.
Effect of paraxanthine administration [0 (control), 5, 10, 50, and 100 mg/kg, i.p.] on the threshold for myoclonic twitch (MCT), generalized clonus (GNC), and forelimb tonus (FLT) onset in the intravenous (i.v.) pentylenetetrazole (PTZ) seizure threshold test. Control animals received only dimethyl sulfoxide (DMSO) as a vehicle. * P < 0.05, ** P < 0.01, *** P < 0.001 compared with the control group (mean ± SEM, N = 8).
![Effect of paraxanthine administration [0 (control), 5, 10, 50, and 100 mg/kg, i.p.] on the threshold for myoclonic twitch (MCT), generalized clonus (GNC), and forelimb tonus (FLT) onset in the intravenous (i.v.) pentylenetetrazole (PTZ) seizure threshold test. Control animals received only dimethyl sulfoxide (DMSO) as a vehicle. * P < 0.05, ** P < 0.01, *** P < 0.001 compared with the control group (mean ± SEM, N = 8). Effect of paraxanthine administration [0 (control), 5, 10, 50, and 100 mg/kg, i.p.] on the threshold for myoclonic twitch (MCT), generalized clonus (GNC), and forelimb tonus (FLT) onset in the intravenous (i.v.) pentylenetetrazole (PTZ) seizure threshold test. Control animals received only dimethyl sulfoxide (DMSO) as a vehicle. * P < 0.05, ** P < 0.01, *** P < 0.001 compared with the control group (mean ± SEM, N = 8).](https://services.brieflands.com/cdn/serve/316a9/c950c0a65eae2f1ac7fa83d4ec8c68b2094c2067/jjnpp-19-3-145031-i002-preview.png)
4.2. Intravenous Pentylenetetrazole Test in Mice Shows that Pretreatment with L-arginine Before Paraxanthine Does Not Influence Seizure Thresholds
Figure 3 illustrates the results of pretreatment with an ineffective dose of L-arginine (50 mg/kg) before an ineffective dose of paraxanthine (5 mg/kg) in the i.v. PTZ test. According to the results of our previous research, we selected a dose of L-arginine (50 mg/kg) that was ineffective (19). Administration of L-arginine alone did not affect any of the seizure stages. Compared to the paraxanthine alone group, pretreatment with L-arginine did not influence the impact of paraxanthine on various seizure phases [F (3,28) = 1.80, P = 0.17 for MCT; F (3,28) = 0.124, P = 0.945 for GNC; F (4,35) = 0.404, P = 0.751 for FLT].
Effect of pretreatment with a dose of 50 mg/kg of paraxanthine of L-arginine (L-arg 50) before a dose of 5 mg/kg of paraxanthine (Px 5) on the threshold for myoclonic twitch (MCT), generalized clonus (GNC), and forelimb tonus (FLT) onset in the intravenous (i.v.) pentylenetetrazole (PTZ) seizure threshold test (mean ± SEM, N = 8)
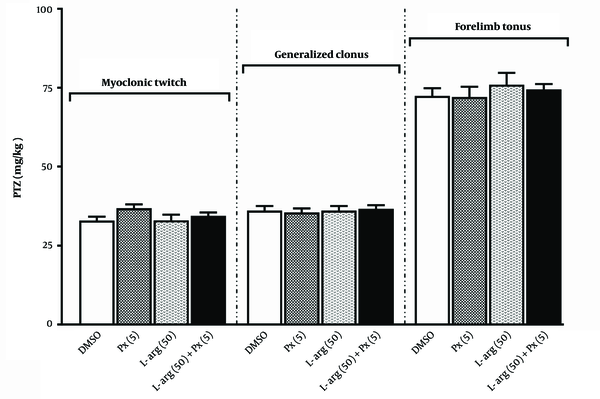
4.3. Intravenous Pentylenetetrazole Test in Mice Shows that Pretreatment with L-NAME Before the Administration of Paraxanthine Influences Seizure Thresholds
The results of an ineffective dose of L-NAME (5 mg/kg) pretreatment before the proconvulsant dose of paraxanthine (100 mg/kg) in the i.v. PTZ tests are shown in Figure 4. The ineffective dose of L-NAME (5 mg/kg) was chosen based on our previously published data (19). L-NAME alone did not alter the threshold for the onset of any examined endpoints. Compared to the group treated with paraxanthine alone, pretreatment with L-NAME significantly abolished the proconvulsant effect of paraxanthine on MCT induction [F (3,28) = 9.14, P = 0.0002 for MCT; F (3,28) = 11.5, P < 0.0001 for GNC; F (4,35) = 3.9, P < 0.0001 for FLT].
Effect of pretreatment with a dose of 5 mg/kg of L-NAME (L-NAME 5) before a dose of 100 mg/kg of paraxanthine (Px 100) on the threshold for myoclonic twitch (MCT), generalized clonus (GNC), and and forelimb tonus (FLT) onset in the intravenous (i.v.) pentylenetetrazole (PTZ) seizure threshold test. * P < 0.05, *** P < 0.001 compared with the control group, +++ P < 0.001 in comparison to the paraxanthine (100 mg/kg) group (mean ± SEM, N = 8).
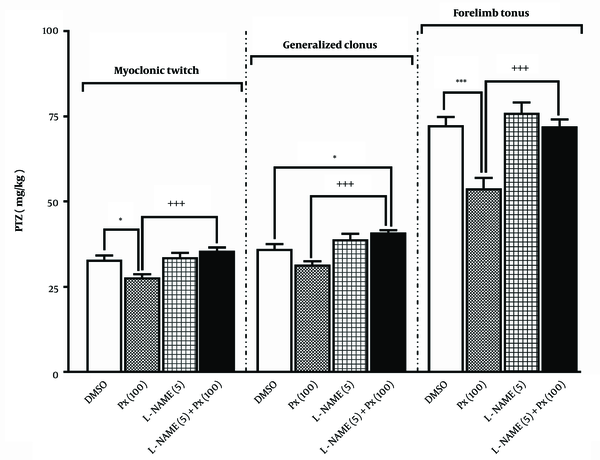
4.4. Effect of Paraxanthine Administration on Brain Nitric Oxide Metabolites Levels
The effect of acutely administered paraxanthine (5, 10, 50, and 100 mg/kg) on brain NOx levels is shown in Figure 5. A one-way ANOVA revealed a significant effect on NOx levels [F (4,35) = 3.07, P = 0.029]. Tukey’s post-hoc test showed that only the 100 mg/kg dose of paraxanthine significantly decreased brain NOx levels compared with the control group (P < 0.05).
Effect of acute paraxanthine administration [0 (control), 5, 10, 50, and 100 mg/kg, i.p.] on brain nitric oxide metabolites (NOx) levels. * P < 0.05 compared with the control group (mean ± SEM, N = 8).
![Effect of acute paraxanthine administration [0 (control), 5, 10, 50, and 100 mg/kg, i.p.] on brain nitric oxide metabolites (NOx) levels. * P < 0.05 compared with the control group (mean ± SEM, N = 8). Effect of acute paraxanthine administration [0 (control), 5, 10, 50, and 100 mg/kg, i.p.] on brain nitric oxide metabolites (NOx) levels. * P < 0.05 compared with the control group (mean ± SEM, N = 8).](https://services.brieflands.com/cdn/serve/316a9/7b240a1258a6a7e174de94615828d7ffd4d10c96/jjnpp-19-3-145031-i005-preview.png)
4.5. Pretreatment with L-arginine or L-NAME Before Paraxanthine Affects Brain Nitric Oxide Metabolites Levels
The effect of pretreatment with an ineffective dose of L-arginine (50 mg/kg) before an ineffective dose of paraxanthine (5 mg/kg) on brain NOx levels is shown in Figure 6. A one-way ANOVA revealed a significant difference in NOx levels among the groups [F (3,28) = 25.9, P < 0.0001]. Tukey's post-hoc test indicated that pretreatment with L-arginine significantly enhanced the effect of paraxanthine in increasing NOx levels (P < 0.0001), compared to the paraxanthine alone group.
As depicted in Figure 6, pretreatment with an ineffective dose of L-NAME (5 mg/kg) before administering a proconvulsant dose of paraxanthine (100 mg/kg) affects brain NOx levels. A one-way ANOVA revealed a significant difference in NOx levels among the groups [F (3,28) = 5.77, P = 0.003]. Paraxanthine at a dose of 100 mg/kg significantly increased brain NOx levels (P < 0.05). Tukey's post-hoc test showed that pretreatment with L-NAME significantly prevented the increase in NOx levels caused by paraxanthine (P < 0.01), compared to the paraxanthine alone group.
Effect of pretreatment with a dose of 50 mg/kg of L-arginine (L-arg 50) before a dose of 5 mg/kg of paraxanthine (Px 5); A, or pretreatment with a dose of 5 mg/kg of L-NAME (L-NAME 5) before a dose of 100 mg/kg of paraxanthine (Px 100); B, on brain nitric oxide metabolites (NOx) levels. * P < 0.05 compared with the control group, +++ P < 0.001 in comparison with paraxanthine per se group (mean ± SEM, N = 8).
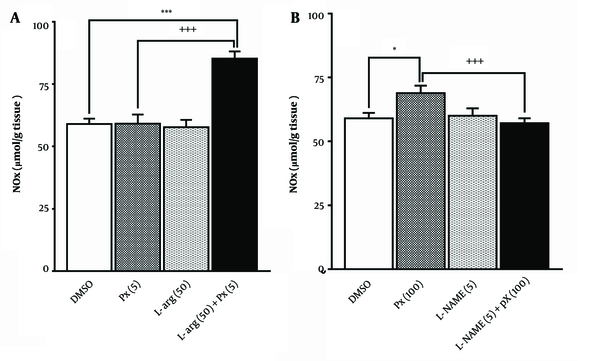
5. Discussion
The results of our investigation reveal that paraxanthine exhibits a proconvulsant effect in an i.v. PTZ test. Pretreatment with L-NAME, a NOS inhibitor, abolished the proconvulsant effect of paraxanthine. In contrast, pretreatment with L-arginine, a substrate for NOS, did not modify this effect.
Most of the caffeine ingested by humans (approximately 80%) is metabolized into paraxanthine (10). Like caffeine, paraxanthine inhibits ARs with similar potency (12). There are four AR subtypes in the brain: A1, A2A, A2B, and A3 ARs. Adenosine exerts its neuromodulatory effects primarily through the activation of A1ARs and A2AARs (25). Postsynaptic activation of A1ARs by adenosine decreases neuronal excitability, whereas postsynaptic activation of A2AARs increases neuronal excitability (26). Knockout receptor studies indicate that A1ARs play a critical role in the anticonvulsant effect of adenosine (27, 28).
Paraxanthine at a dose of 100 mg/kg significantly lowered the threshold for the onset of MCT, GNC, and FLT (Figure 2). To our knowledge, only one study has examined the effects of paraxanthine on seizures. Forelimb tonus significantly decreased when paraxanthine was administered in drinking water (0.5 g/L) for 14 days, but the clonic seizure threshold was marginally non-significant (20). Numerous studies have reported neuroprotective, psychostimulant, and locomotor-activating effects of paraxanthine (22, 29, 30). However, paraxanthine appears protective at much lower doses than its proconvulsant effect.
Only paraxanthine at a dose of 100 mg/kg significantly reduced the thresholds for the first MCT and GNC. In contrast, paraxanthine at doses of 10, 50, and 100 mg/kg significantly lowered the threshold for the onset of FLT (Figure 2). While MCT and GNC arise from the cerebellum, FLT originates from the hindbrain (31). A1ARs are widely distributed in both the forebrain and hindbrain, while A2AARs are exclusively distributed in the forebrain structures (32). Adenosine at low doses activates A1ARs, resulting in a reduced release of dopamine and glutamate. In contrast, high doses of adenosine increase glutamate release by activating A2AARs (33).
As a result, discrepancies in the effects of paraxanthine on the first MCT, GNC, and FLT may be partly explained by differences in the distribution of the A1ARs and A2AARs in the central nervous system (CNS). The exclusive distribution of A1ARs in the hindbrain likely accounts for paraxanthine's proconvulsant effects and the reduced threshold for FLT at doses of 10, 50, and 100 mg/kg. When paraxanthine is administered at low doses (10 and 50 mg/kg), its proconvulsant effect on A1ARs may be obscured by its antagonistic effect on A2AARs in the forebrain.
Caffeine and paraxanthine are non-competitive AR antagonists (9, 12). However, comparing the findings of the present study with our previous work reveals both similarities and differences in the effects of paraxanthine and caffeine on PTZ-induced seizures. At doses of 5 and 50 mg/kg, caffeine exhibited a proconvulsant effect, whereas at a dose of 100 mg/kg, it did not influence PTZ-induced clonic seizures (18). Our recent study demonstrated that the threshold dose for the onset of all seizure endpoints was significantly lowered by caffeine at doses of 5 and 50 mg/kg. Conversely, caffeine at a dose of 100 mg/kg reduced only the threshold for FLT seizures (19).
Although some reports suggest that caffeine is a less potent A1AR and A2AAR antagonist than paraxanthine (9, 12), other studies indicate that caffeine and paraxanthine have similar affinities for A1AR and A2AAR (22, 34). Adenosine receptors are not essential for the high-dose effects of caffeine (35). Thus, caffeine and paraxanthine may have similar effects, partly due to their similar affinities to ARs. Caffeine and paraxanthine impact seizures differently, likely due to mechanisms other than AR blockade. Some reports suggest that the NO-cGMP pathway is more significant for the central effects of paraxanthine compared to caffeine (22, 29).
Our results show that pretreatment with L-arginine before an ineffective dose of paraxanthine (5 mg/kg) did not affect the seizure threshold (Figure 3), while pretreatment with L-NAME eliminated the proconvulsant effect of paraxanthine at a dose of 100 mg/kg (Figure 4).
As a neurotransmitter and neuromodulator, NO participates in many physiological and pathological functions. Its role involves stimulating the formation of cGMP through the activation of soluble guanylate cyclase (sGC). By activating different isoforms of protein kinase G (PKG), cGMP regulates neurotransmitter release, synaptic transmission, neuronal differentiation, and gene expression. Nitric oxide plays a significant role in seizure susceptibility, but there are discrepancies in the results of various studies. Depending on the seizure type, NO source, and other neurotransmitter systems involved, NO can exert either proconvulsant or anticonvulsant effects. There is a complex interaction between adenosine and NO. Adenosine has an anticonvulsant effect in which NO is implicated. Meanwhile, the antidepressant action of adenosine also involves NO. We have recently demonstrated that the NO-cGMP pathway participates in caffeine's effect on the PTZ-induced seizure threshold.
A high dose of paraxanthine (100 mg/kg) elevated the NOx levels in brain tissues (Figure 5). Reductions in MCT and GNC thresholds were observed only with this dose of paraxanthine. L-NAME attenuated the proconvulsant effect of paraxanthine (Figure 3), whereas L-arginine had no effect (Figure 3). Nitric oxide metabolites levels increased after L-arginine pretreatment before an otherwise ineffective dose of paraxanthine (Figure 6). In contrast, L-NAME pretreatment before a proconvulsant dose of paraxanthine reduced NOx levels in the brain (Figure 6). These results suggest that the proconvulsant effect of paraxanthine is mediated by the NO-cGMP pathway.
No research has been conducted to determine if paraxanthine influences seizures via the NO-cGMP pathway. Several studies have examined the relationship between paraxanthine and locomotion (22, 29). Adenosine receptor blockade increases locomotor activity, and activation of the NO-cGMP pathway can enhance it. Paraxanthine can effectively inhibit PDE9, as mentioned previously (29). Consequently, paraxanthine may exert some of its effects through PDE9 inhibition and an increase in cGMP levels. Pretreatment with L-NAME reduces the locomotor-activating effect of paraxanthine but does not affect caffeine's locomotor activity. In ex vivo experiments, paraxanthine increases striatal cGMP levels, whereas caffeine does not (22). It is reasonable to assume that part of the proconvulsant effect of paraxanthine may be mediated by the activation of the NO-cGMP signaling pathway.
5.1. Conclusions
We concluded that the acute administration of paraxanthine has a proconvulsant effect. A dose-dependent effect of paraxanthine was observed at various stages of PTZ-induced seizures. Finally, the levels of brain NOx in animals administered L-arginine or L-NAME before paraxanthine administration suggest an interaction of the NO-cGMP pathway in the proconvulsant effect of paraxanthine.
References
-
1.
Johnson EL. Seizures and Epilepsy. Med Clin North Am. 2019;103(2):309-24. [PubMed ID: 30704683]. https://doi.org/10.1016/j.mcna.2018.10.002.
-
2.
Bano A, Garg A, Ashif Khan M; Mumtaz; Nidhi. The anti-seizure potential of cysteine leukotriene receptor antagonists: A systematic review of animal studies. Epilepsy Res. 2024;200:107305. [PubMed ID: 38325237]. https://doi.org/10.1016/j.eplepsyres.2024.107305.
-
3.
Willems LM, Bauer S, Rosenow F, Strzelczyk A. Recent advances in the pharmacotherapy of epilepsy: brivaracetam and perampanel as broad-spectrum antiseizure drugs for the treatment of epilepsies and status epilepticus. Expert Opin Pharmacother. 2019;20(14):1755-1765.. [PubMed ID: 31264486]. https://doi.org/10.1080/14656566.2019.1637420.
-
4.
Ngoupaye GT, Adassi MB, Foutsop AF, Yassi FB, NgoBum E. Pentylenetetrazole kindling-induced epilepsy rat models: Insight on the severity state, a comparative study. IBRO Neurosci Rep. 2022;17(13):164-76. [PubMed ID: 36590094]. [PubMed Central ID: PMC9795317]. https://doi.org/10.1016/j.ibneur.2022.08.003.
-
5.
Ekstein D, Schachter SC. Natural Products in Epilepsy-the Present Situation and Perspectives for the Future. Pharmaceuticals (Basel). 2010;3(5):1426-45. [PubMed ID: 27713311]. [PubMed Central ID: PMC4033990]. https://doi.org/10.3390/ph3051426.
-
6.
Andrabi SM, Sharma NS, Karan A, Shahriar SMS, Cordon B, Ma B, et al. Nitric Oxide: Physiological Functions, Delivery, and Biomedical Applications. Adv Sci (Weinh). 2023;10(30). e2303259. [PubMed ID: 37632708]. [PubMed Central ID: PMC10602574]. https://doi.org/10.1002/advs.202303259.
-
7.
Kaster MP, Rosa AO, Santos AR, Rodrigues AL. Involvement of nitric oxide-cGMP pathway in the antidepressant-like effects of adenosine in the forced swimming test. Int J Neuropsychopharmacol. 2005;8(4):601-6. [PubMed ID: 16202183]. https://doi.org/10.1017/S1461145705005316.
-
8.
Faghir-Ghanesefat H, Keshavarz-Bahaghighat H, Rajai N, Mokhtari T, Bahramnejad E, Kazemi Roodsari S, et al. The Possible Role of Nitric Oxide Pathway in Pentylenetetrazole Preconditioning Against Seizure in Mice. J Mol Neurosci. 2019;67(3):477-83. [PubMed ID: 30627955]. https://doi.org/10.1007/s12031-018-1256-2.
-
9.
Matsumura N, Aoyama K. Glutathione-Mediated Neuroprotective Effect of Purine Derivatives. Int J Mol Sci. 2023;24(17). [PubMed ID: 37685879]. [PubMed Central ID: PMC10487553]. https://doi.org/10.3390/ijms241713067.
-
10.
Purkiewicz A, Pietrzak-Fiecko R, Sorgel F, Kinzig M. Caffeine, Paraxanthine, Theophylline, and Theobromine Content in Human Milk. Nutrients. 2022;14(11). [PubMed ID: 35683994]. [PubMed Central ID: PMC9182860]. https://doi.org/10.3390/nu14112196.
-
11.
Arnaud MJ. Pharmacokinetics and metabolism of natural methylxanthines in animal and man. Handb Exp Pharmacol. 2011;(200):33-91. [PubMed ID: 20859793]. https://doi.org/10.1007/978-3-642-13443-2_3.
-
12.
Yoo C, Xing D, Gonzalez D, Jenkins V, Nottingham K, Dickerson B, et al. Acute Paraxanthine Ingestion Improves Cognition and Short-Term Memory and Helps Sustain Attention in a Double-Blind, Placebo-Controlled, Crossover Trial. Nutrients. 2021;13(11). [PubMed ID: 34836235]. [PubMed Central ID: PMC8622427]. https://doi.org/10.3390/nu13113980.
-
13.
Fredholm BB. Adenosine receptors as drug targets. Exp Cell Res. 2010;316(8):1284-8. [PubMed ID: 20153317]. [PubMed Central ID: PMC2866745]. https://doi.org/10.1016/j.yexcr.2010.02.004.
-
14.
Muller CE, Jacobson KA. Recent developments in adenosine receptor ligands and their potential as novel drugs. Biochim Biophys Acta. 2011;1808(5):1290-308. [PubMed ID: 21185259]. [PubMed Central ID: PMC3437328]. https://doi.org/10.1016/j.bbamem.2010.12.017.
-
15.
van Koert RR, Bauer PR, Schuitema I, Sander JW, Visser GH. Caffeine and seizures: A systematic review and quantitative analysis. Epilepsy Behav. 2018;80:37-47. [PubMed ID: 29414557]. https://doi.org/10.1016/j.yebeh.2017.11.003.
-
16.
Jargiello-Baszak M, Chroscinska-Krawczyk M, Andres-Mach M, Luszczki JJ, Czuczwar SJ. Influence of caffeine on the protective activity of gabapentin and topiramate in a mouse model of generalized tonic-clonic seizures. Pharmacol Rep. 2016;68(4):680-5. [PubMed ID: 27116699]. https://doi.org/10.1016/j.pharep.2016.03.011.
-
17.
Germe K, Faure JB, Koning E, Nehlig A. Effect of caffeine and adenosine receptor ligands on the expression of spike-and-wave discharges in Genetic Absence Epilepsy Rats from Strasbourg (GAERS). Epilepsy Res. 2015;110:105-14. [PubMed ID: 25616462]. https://doi.org/10.1016/j.eplepsyres.2014.11.022.
-
18.
Esmaili Z, Heydari A. Effect of acute caffeine administration on PTZ-induced seizure threshold in mice: Involvement of adenosine receptors and NO-cGMP signaling pathway. Epilepsy Res. 2019;149:1-8. [PubMed ID: 30391360]. https://doi.org/10.1016/j.eplepsyres.2018.10.013.
-
19.
Jamali H, Heydari A. Interactions of caffeine and nitric oxide on pentylenetetrazole-induced clonic and tonic seizures in mice. Biochem Nutr Metab Dis. 2024;1(1):16-23.
-
20.
Johansson B, Georgiev V, Kuosmanen T, Fredholm BB. Long-term treatment with some methylxanthines decreases the susceptibility to bicuculline- and pentylenetetrazol-induced seizures in mice. Relationship to c-fos expression and receptor binding. Eur J Neurosci. 1996;8(12):2447-58. [PubMed ID: 8996794]. https://doi.org/10.1111/j.1460-9568.1996.tb01539.x.
-
21.
Liu YJ, Chen J, Li X, Zhou X, Hu YM, Chu SF, et al. Research progress on adenosine in central nervous system diseases. CNS Neurosci Ther. 2019;25(9):899-910. [PubMed ID: 31334608]. [PubMed Central ID: PMC6698970]. https://doi.org/10.1111/cns.13190.
-
22.
Orru M, Guitart X, Karcz-Kubicha M, Solinas M, Justinova Z, Barodia SK, et al. Psychostimulant pharmacological profile of paraxanthine, the main metabolite of caffeine in humans. Neuropharmacol. 2013;67:476-84. [PubMed ID: 23261866]. [PubMed Central ID: PMC3562388]. https://doi.org/10.1016/j.neuropharm.2012.11.029.
-
23.
Heydari A, Davoudi S. The effect of sertraline and 8-OH-DPAT on the PTZ_induced seizure threshold: Role of the nitrergic system. Seizure. 2017;45:119-24. [PubMed ID: 28012414]. https://doi.org/10.1016/j.seizure.2016.12.005.
-
24.
Mesdaghinia A, Alinejad M, Abed A, Heydari A, Banafshe HR. Anticonvulsant effects of thiamine on pentylenetetrazole-induced seizure in mice. Nutr Neurosci. 2019;22(3):165-73. [PubMed ID: 28766407]. https://doi.org/10.1080/1028415X.2017.1357919.
-
25.
Stockwell J, Jakova E, Cayabyab FS. Adenosine A1 and A2A Receptors in the Brain: Current Research and Their Role in Neurodegeneration. Mol. 2017;22(4). [PubMed ID: 28441750]. [PubMed Central ID: PMC6154612]. https://doi.org/10.3390/molecules22040676.
-
26.
Ribeiro JA, Sebastiao AM, de Mendonca A. Participation of adenosine receptors in neuroprotection. Drug News Perspect. 2003;16(2):80-6. [PubMed ID: 12792668]. https://doi.org/10.1358/dnp.2003.16.2.740246.
-
27.
Kochanek PM, Vagni VA, Janesko KL, Washington CB, Crumrine PK, Garman RH, et al. Adenosine A1 receptor knockout mice develop lethal status epilepticus after experimental traumatic brain injury. J Cereb Blood Flow Metab. 2006;26(4):565-75. [PubMed ID: 16121125]. https://doi.org/10.1038/sj.jcbfm.9600218.
-
28.
Akula KK, Dhir A, Kulkarni SK. Nitric oxide signaling pathway in the anti-convulsant effect of adenosine against pentylenetetrazol-induced seizure threshold in mice. Eur J Pharmacol. 2008;587(1-3):129-34. [PubMed ID: 18457833]. https://doi.org/10.1016/j.ejphar.2008.03.038.
-
29.
Ferre S, Orru M, Guitart X. Paraxanthine: Connecting Caffeine to Nitric Oxide Neurotransmission. J Caffeine Res. 2013;3(2):72-8. [PubMed ID: 24761277]. [PubMed Central ID: PMC3680978]. https://doi.org/10.1089/jcr.2013.0006.
-
30.
Guerreiro S, Toulorge D, Hirsch E, Marien M, Sokoloff P, Michel PP. Paraxanthine, the primary metabolite of caffeine, provides protection against dopaminergic cell death via stimulation of ryanodine receptor channels. Mol Pharmacol. 2008;74(4):980-9. [PubMed ID: 18621927]. https://doi.org/10.1124/mol.108.048207.
-
31.
Wang YJ, Hsieh CP, Chan MH, Chan TY, Chen L, Chen HH. Distinct effects of resveratrol on seizures and hyperexcitability induced by NMDA and 4-aminopyridine. Nutr Neurosci. 2019;22(12):867-76. [PubMed ID: 29642764]. https://doi.org/10.1080/1028415X.2018.1461458.
-
32.
Dunwiddie TV, Masino SA. The role and regulation of adenosine in the central nervous system. Annu Rev Neurosci. 2001;24:31-55. [PubMed ID: 11283304]. https://doi.org/10.1146/annurev.neuro.24.1.31.
-
33.
Borycz J, Pereira MF, Melani A, Rodrigues RJ, Kofalvi A, Panlilio L, et al. Differential glutamate-dependent and glutamate-independent adenosine A1 receptor-mediated modulation of dopamine release in different striatal compartments. J Neurochem. 2007;101(2):355-63. [PubMed ID: 17254024]. https://doi.org/10.1111/j.1471-4159.2006.04386.x.
-
34.
Snyder SH, Katims JJ, Annau Z, Bruns RF, Daly JW. Adenosine receptors and behavioral actions of methylxanthines. Proc Natl Acad Sci USA. 1981;78(5):3260-4. [PubMed ID: 6265942]. [PubMed Central ID: PMC319541]. https://doi.org/10.1073/pnas.78.5.3260.
-
35.
Fredholm BB, Yang J, Wang Y. Low, but not high, dose caffeine is a readily available probe for adenosine actions. Mol Aspects Med. 2017;55:20-5. [PubMed ID: 27915051]. https://doi.org/10.1016/j.mam.2016.11.011.