Abstract
Keywords
Valproic Acid Drug-Induced Liver Injury HepG2 miR-122 miR-155
1. Background
The liver is the largest and one of the most crucial organs in the human body, serving as the site for necessary chemical reactions. Its role in detoxifying toxic substances and synthesizing valuable biomolecules is significant. Consequently, any liver injury leads to severe consequences (1). These injuries are caused by factors such as viral hepatitis, hereditary metabolic diseases, alcohol consumption, and chemical components. Chemicals that cause liver damage are called hepatotoxins (2). Excessive use of some drugs, including natural or chemical compounds (3), herbal medicines, and food supplements (4), can also be classified as hepatotoxins. Liver toxicity may be observed even when taking some drugs within the prescribed therapeutic dose range (4).
Drug-induced liver injury (DILI) is one of the frequent differential diagnoses in patients with severe liver damage of unknown origin. According to available reports, some drugs cause liver damage in many cases, and most of these drugs have known clinical symptoms when they damage the liver (5).
Valproic acid (2-propylpentanoic acid, VPA) is a small synthetic chemical compound known as the first-line and most widely used antiepileptic agent for the past 40 years (6). However, it is currently also prescribed for the treatment of other diseases such as generalized seizures in adults and children (7), bipolar disorder, migraine, cancer, multiple sclerosis, and Alzheimer's disease (8). Clinical studies have reported obesity and fatty liver disease in about 40% of patients who received VPA (9). The World Health Organization (WHO) has reported valproic acid as the third most common drug inducing liver death (10). The mechanism of liver injury is not fully known, but it may be related to interference with mitochondrial fatty acid β-oxidation (11).
miR-122 is a liver tissue-specific miRNA present in high amounts (about 70% of the total liver miRNA population). This specific microRNA is not visible in other tissues and plays an essential role in the growth and development of the liver, as well as in liver diseases (12). It has been determined that the expression levels of miR-122 in the liver and the amount released into the circulation are affected by various types of stress to the liver, including hepatotoxicity, viral hepatitis, and cancer. Accordingly, miR-122 can be considered a biomarker of DILI (13).
In addition to miR-122, miR-155 also regulates various liver diseases, including liver damage, steatosis, and fibrosis (14). Although the mechanism of action of miR-155 has not yet been determined, the increased levels of miR-155 in serum and plasma in patients with liver damage caused by alcohol and inflammation indicate that miR-155 plays a role in liver damage (15).
Overall, expression changes of miR-122 and miR-155 can be used as sensitive biomarkers for DILI.
2. Objectives
In the present study, the hepatotoxic effect of valproic acid on the HepG2 liver cell line was measured by cytotoxicity tests and by evaluating the changes in the expression of miR-122 and miR-155.
3. Methods
All methods performed in this study were designed according to the guidelines and regulations of the American Type Culture Collection (ATCC) (https://www.atcc.org/ ). All experiments were conducted after receiving ethical approval from the research ethics committee of Ahvaz Jundishapur University of Medical Sciences (ethical code: IR.AJUMS.REC.1399.670). We did not deal directly with patients, and the cells used in this study were purchased from the Iranian Biological Resource Center (IBRC) (http://en.ibrc.ir/ ).
3.1. Chemicals
Valproic acid (Batch No: 98SV021) was obtained from Rouz Darou Co. (Tehran, Iran). 3-(4,5-dimethylthiazol-2-yl)-2,5-diphenyl-2H-tetrazolium bromide (MTT) (lot # MKCK7253V) was purchased from Sigma. Dimethyl sulfoxide (DMSO) was purchased from Sinaclon Co. Trypan Blue (0.4%) was obtained from Bio-Idea (Tehran, Iran). Dulbecco's modified eagle medium/nutrient mixture F-12 (DMEM-F12) was purchased from Bio-Idea (Tehran, Iran) (Batch: 03831). Fetal bovine serum (FBS) was purchased from Bio-Idea (Tehran, Iran) (Batch: 02921). Trypsin-EDTA 0.25% (1×) was purchased from Bio-Idea (Tehran, Iran) (BI-1610). Penicillin-streptomycin (100X) (REF:10-PS1-100) was obtained from Vivacell Kit. First Strand cDNA synthesis kits were obtained from Sinaclon Co. (Cat No: RT5201). All primers were purchased from the Stem Cell Technology Research Centre (Bonyakhteh Co., Tehran, Iran). YTA Super SYBR Green qPCR MasterMix 2X (lot No: S057) and YTA Passive Reference Dye 40 ul-50X (lot: SLBV2296) were obtained from Yekta Tajhiz Azma Co. (Tehran, Iran).
3.2. Cell Culture
The HepG2 cells were purchased from the Stem Cell Technology Research Center and grown in 25 cm² culture flasks in a CO2 incubator. The cells were maintained in DMEM-F12 supplemented with 10% heat-inactivated fetal bovine serum and 1% penicillin/streptomycin. The entire process was performed under aseptic conditions. Cells were incubated at 37°C with 95% air and 5% CO2. The medium was renewed every 2 - 3 days, and cells were passaged when they reached 60% confluence. For subculturing, cells were detached by treatment with 0.25% trypsin/0.02% EDTA at 37°C. Cell suspensions were aspirated and then centrifuged at 1000 rpm for 5 minutes. They were then counted using a hemocytometer and a 0.4% Trypan Blue solution for seeding in experimental plates.
3.3. Valproic Acid (Sodium Valproate) Treatment and MTT Assay
To evaluate the effect of VPA on HepG2 cells, therapeutic doses ranging from 500 mg to 2000 mg were used. According to previous studies, 250 mg of consumed VPA induced a serum concentration of 54.6 µg/mL (0.34 mM) (16). Hence, the application of VPA in the range of 500 to 2000 mg is equivalent to 0.68 mM and 2.72 mM in serum, respectively. Referring to previous studies (17, 18), we examined VPA at concentrations of 0.7, 1.5, and 3 mM. VPA stock was prepared by dissolving the VPA powder in serum-free DMEM-F12. All compounds were sterilized by filtration.
HepG2 cells were seeded into a 96-well plate at a density of 104 cells per well and incubated for 24 hours in a 5% CO2 incubator at 37°C. Cells were allowed to reach exponential growth. Then, the medium was removed, and 100 µL of medium containing different concentrations of VPA (0, 0.7, 1.5, and 3 mM) was added to the wells. Each concentration was assessed in three replicate wells and at five-time points, including 24 h, 48 h, 72 h, 96 h, and 120 h. The results were reported as percent toxicity versus control, with non-treated cells taken as 0% toxicity.
At each time point, cells were incubated with 10 µL of 5 mg/mL MTT (3-(4,5-dimethylthiazol-2-yl)-2,5-diphenyltetrazolium bromide) dissolved in serum-free medium at 37°C within a humidified 5% CO2 atmosphere for 3 hours. Cells with metabolic activity cleaved the yellow tetrazolium salt to a purple formazan dye. Subsequently, the medium was removed after 3 hours, and formazan crystals were dissolved in 100 µL of DMSO with 25 minutes of stirring. Absorbance was determined using a microplate reader at 570/630 nm. The percentage of viable cells was calculated using the following formula:
3.4. RNA Extraction and Gene Expression
3.4.1. Cell Treatments and RNA Extraction
Cell suspensions were seeded in a 6-well plate at a density of 7 × 105 cells per well and incubated in a 37°C incubator with 5% CO2. After 48 hours, the culture media in the wells were discarded, and culture media containing the drug were added. Different concentrations of VPA (0, 0.7, 1.5, and 3 mM) were tested in two replicate wells. Afterward, treated cells were harvested using trypsinization, and total RNA was extracted with the Jena Bioscience Kit (Cat No: PP-210S) according to the manufacturer’s instructions. The quality and integrity of the extracted RNA were precisely measured by spectrophotometry at 260 and 280 nm.
3.4.2. cDNA Synthesise and microRNA Expression Analysis Using Real-time PCR
To study miRNA expression, we adapted the stem-loop RT-PCR method (19). Ten µL of total RNA was reverse transcribed into cDNA using 1 µL specific RT primers (miR-122, miR-155, and U6 specific stem-loop (Lot#004RT)) and a cDNA Synthesis Kit on a Thermo Cycler according to the manufacturer’s protocol. The cycling conditions for the RT reaction were as follows: 25°C for 5 min, 42°C for 60 min, 85°C for 5 min, and 4°C for 1 min. The levels of miR-122 and miR-155 were measured using real-time PCR. The cDNA was amplified using SYBR Green Master Mix qPCR. Quantitative PCR was performed with the ABI Step OnePlus™ system (Applied Biosystems). U6 small nuclear RNA (snRNA) was used as the miRNA internal control. The PCR reaction included 2 µL cDNA, 1 µL forward primers (miR-122, miR-155, U6 (Lot#004FP)), 1 µL universal reverse primer (Lot#0104BR), and 5 µL SYBR select master mix. The PCR cycling conditions were as follows: 50°C for 1 min, followed by 40 cycles of 95°C for 15 sec and 60°C for 1 min. Relative miRNA production was calculated using the 2-∆∆Ct method (20), where Ct is the quantification cycle. All reactions were run in duplicate, and changes in gene expression were determined by normalizing to U6 snRNA levels.
3.5. Statistical Analysis
Data were analyzed by two-way ANOVA, and the results were presented as mean ± SEM of three separate experiments. Differences between groups were assessed using the Bonferroni correction. Statistical analysis was performed using GraphPad Prism 9.2 software, and P < 0.05 was considered statistically significant. P < 0.05, P < 0.01, P < 0.0001.
Data from the real-time PCR assay were used to compute the normalized ratio of microRNA levels in the treated HepG2 cells relative to the control at respective time points. The calculations were performed with REST©2009 software (21).
4. Results
4.1. Effect of the VPA on the Viability of HepG2 Cells
In this study, HepG2 cells were exposed to different concentrations of VPA (0.7, 1.5, and 3 mM) at five incubation time points (24, 48, 72, 96, and 120 h), and cell viability was assessed using the MTT test. As shown in Figure 1, there was a statistically significant difference between the treated groups (0.7, 1.5, and 3 mM) and the control group, with VPA ranging from 0.7 to 3 mM inducing cell death in a dose-dependent manner (P < 0.0001). Treatment of HepG2 cells with 3 mM VPA after 120 h caused a significant reduction in cell viability (58.17 ± 3.62% to control, P < 0.0001). However, cell viability was slightly higher than the control at 0.7 mM and 1.5 mM doses (68.63 ± 3.62% and 67.21 ± 3.62%, respectively, P < 0.01 for both). Furthermore, the application of VPA at various incubation time points (24, 48, 72, 96, and 120 h) was significant (P < 0.049). Based on the resulting data, subsequent experiments were conducted at the best time points, including 24 h as the baseline time and 120 h as the most growth-inhibitory incubation time (P < 0.01).
Effect of different valproic acid (VPA) concentrations on the HepG2 cells viability assessed by MTT after 120 h treatment. The data was shown as a percentage of treated samples compared to the control. ** P < 0.01, *** P < 0.0001.
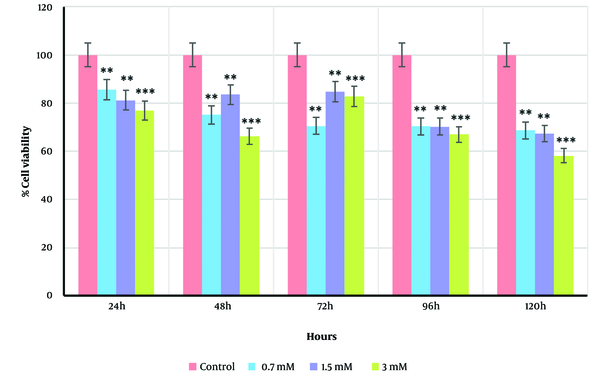
4.2. miR-122/-155 Expression Using qPCR
To evaluate the impact of VPA on miR-122 and miR-155 expression in HepG2 cells, qPCR was used. The HepG2 cells were exposed to 0.7, 1.5, and 3 mM VPA for 24 h and 120 h, with untreated cells serving as the control group. RNA isolation and qPCR were performed as described above. The qPCR results (Table 1) showed that exposure to VPA significantly increased the transcription levels of miR-122 and miR-155 at 24 h. Conversely, the expression of both miRNAs was down-regulated after 120 h (P < 0.01) (Figure 2).
Expression Levels of Target miRNAs in the HepG2 Cells After 24 h and 120 h Treatment by Different Valproic Acid Doses (Relative miRNA Production was Calculated Using the 2-∆CT Method)
Time Points and VPA Doses | Expression Level (2-ΔCT)/Control | P-Value | Up/Down |
---|---|---|---|
Target gene: miR-122 | |||
0.7 mM | |||
24 h | 37.53 | < 0.01 | Up |
120 h | 0.0003 | < 0.01 | Down |
24/120 h | 125100 | ||
1.5 mM | |||
24 h | 0.0025 | < 0.01 | Up |
120 h | 0.1812 | < 0.01 | Down |
24/120 h | 0.014 | ||
3 mM | |||
24 h | 0.3415 | < 0.01 | Up |
120 h | 0.0254 | < 0.01 | Down |
24/120 h | 13.45 | ||
Target gene: miR-155 | |||
0.7 mM | |||
24 h | 1024 | < 0.01 | Up |
120 h | 2.22 | < 0.01 | Down |
24/120 h | 462.08 | ||
1.5 mM | |||
24 h | 0.877 | < 0.01 | Up |
120 h | 1.84 | < 0.01 | Down |
24/120 h | 0.478 | ||
3 mM | |||
24 h | 0.395 | < 0.01 | Up |
120 h | 3.22 | < 0.01 | Down |
24/120 h | 0.123 |
Transcriptional levels of miR-122 and miR-155 in the untreated HepG2 cells (control) (A); and HepG2 cells treated with different concentration of valproic acid (VPA), including 0.7 mM (B); 1.5 mM (C); and 3 mM (D); measured by qPCR after: 24 h and 120 h. * P < 0.05; *** P < 0.0001.
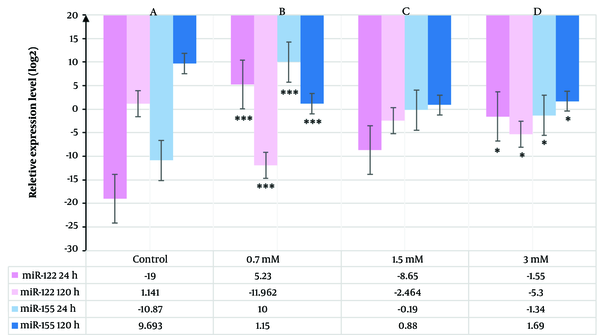
As shown in Figure 2A and C, the expression levels of miR-122 and miR-155 increased significantly over 24 h, with a significant difference observed in cells treated with 0.7 mM VPA compared to control groups (P < 0.01). Conversely, a remarkable down-regulation of both miR-122 and miR-155 was observed after 120 h (Figure 2B and D). Notably, when comparing transcription levels of both miRNAs over the treatment period, the highest fold change was observed for miR-122, except for 1.5 mM at 24 h and 0.7 mM at 120 h, where miR-155 levels were marginally higher than those of miR-122.
5. Discussion
Due to the serious liver-related morbidity and mortality caused by DILI, it is recognized as a significant health issue worldwide (22). The hepatotoxicity induced by VPA has been addressed in several previous studies (23-26). miRNAs are small endogenous RNAs that regulate numerous physiological and pathological processes by controlling gene expression at the post-transcriptional level (27). Recently, miRNAs have emerged as potential biomarkers for diagnosing human DILI (28). Among miRNAs expressed by hepatocytes, miR-122 is the most enriched (70%) in the adult liver (29), and along with miR-155, it targets different genes involved in liver injury (30).
To investigate the effect of VPA on the expression of both miR-122 and miR-155, we conducted an experiment using the HepG2 cells in an in vitro model at different time points and VPA concentrations. We found that exposure to VPA in HepG2 cells induced cell death in a time- and dose-dependent manner. Previous studies have also shown that VPA-induced liver injury is dependent on dose and time (31, 32). Komulainen et al. suggested that VPA induces mitochondrial dysfunction, oxidative stress, and increased cell death, which are related to hepatotoxicity (33).
Some studies have suggested miR-122 as a useful new biomarker in diagnosing DILI (28, 34-36). They revealed that miR-122 is an early and specific marker of hepatocellular injury and a sensitive marker of DILI (28, 36). It has been shown that miR-122 can predict the onset of liver injury before ALT levels are elevated (37). miR-155 is also a well-known microRNA that mediates inflammatory responses (38) by modifying the expression of various target genes (39), and it participates in the development of DILI through the induction of inflammatory responses (40, 41). Our results showed that both miR-122 and miR-155 expression levels were significantly up-regulated following VPA treatment, particularly at a concentration of 0.7 mM after 24 h (Table 1).
A previous study in humans with APAP poisoning showed that serum miR-122 and miR-192 were substantially higher in acetaminophen-induced acute liver injury (APAP-ALI) patients. It also demonstrated that miR-122 levels rose alongside serum ALT levels in mice with APAP-ALI (28). Significant elevations in mean levels of miR-122 were also observed along with necrosis biomarkers (sorbitol dehydrogenase, cytokeratin 18, and HMGB1) and the apoptosis biomarker caspase-cleaved cytokeratin 18 following cholestyramine treatment (36). Up-regulated miR-155 was reported to mediate hepatocyte apoptosis by producing pro-inflammatory cytokines like tumor necrosis factor-α (TNF-α) (42).
Similarly, in the current study, treatment of HepG2 cells with VPA significantly elevated the transcription levels of miR-122 and miR-155 at 24 h. However, extended exposure to VPA for 120 h significantly decreased the expression levels of both miR-122 and miR-155 compared to the control group. Similar results were obtained in another study that demonstrated the prognostic significance of miR-122 expression in patients with hepatocellular carcinoma (HCCs). It was revealed that miR-122 expression is frequently down-regulated in HCCs and is an independent prognostic factor for recurrence-free survival (RFS) after curative resection (43). Another study showed that miR-122 expression was down-regulated in rodent and human HCCs (44), and the reduction of miR-122 expression could promote the viability and inhibit the apoptosis of some hepatoma cells (45).
Consistent with the present results, other studies have demonstrated that VPA can cause cell death through apoptosis and necrosis in rat liver cell lines (26), HCC cell lines (46), and cancer colon cell lines (18) by inducing apoptotic genes (46). Moreover, Wei et al. (47) showed that up-regulation of miR-122 could stimulate apoptosis and inhibit cell viability of HCC by down-regulating TLR4 (48), leading to enhanced expression of pro-apoptotic genes and suppression of anti-apoptotic genes (47).
Previous studies have reported that various factors affect miR-122 expression differently. HBV infection down-regulates miR-122 expression, while HCV infection up-regulates miR-122 expression (49-51).
Regarding the observed changes in the expression of microRNAs in this study, one possible explanation for the dramatic decline in miR-122 and miR-155 expression levels in the treated cells is that it correlates with VPA-induced apoptosis, which increased cell death after 120 hours of VPA dosing. According to our MTT results, increased cell death and a reduced cell number were detected under VPA exposure. However, the expression of miR-122 was more variable than that of miR-155, which may reflect different regulatory mechanisms for miR-122 compared to miR-155 (52). The complexity of miR-122 precursor regulation has been reported previously. It has even been suggested that the function of a microRNA may not be determined solely by its expression level. Therefore, additional future studies are needed to provide more information about this.
Nevertheless, the downregulation of miR-122 expression in patients using VPA can be considered an independent prognostic factor for DILI. Hence, developing diagnostic approaches that evaluate miR-122 expression could be beneficial for patients taking VPA. VPA-induced hepatic injury frequently occurs during both short-term and long-term drug administration, and it can also cause delayed hepatic injury after several months or years (32, 53). It has been proposed that despite the early dysfunction of mitochondrial respiration induced by VPA, increased cell death is detectable only after prolonged exposure to high concentrations of VPA (33). This is consistent with the clinical course of VPA-induced liver failure (32, 53).
The future perspective of this study would involve examining specific groups of miRNAs involved in regulating genes associated with apoptotic cell death. This would provide information about the possible molecular mechanisms underlying VPA-induced apoptosis in vitro and in vivo.
5.1. Conclusions
In this study, we evaluated hepatocyte-specific miRNAs in VPA-treated HepG2 cells. The results demonstrated that the expression of miR-122 and miR-155 effectively represents liver damage induced by VPA in the early stages of exposure. Collectively, the findings suggest that miR-122 and miR-155 may play a significant role in the detoxification process of valproic acid. These results have potential prognostic and therapeutic implications.
Acknowledgements
References
-
1.
Conde de la Rosa L, Goicoechea L, Torres S, Garcia-Ruiz C, Fernandez-Checa JC. Role of Oxidative Stress in Liver Disorders. Livers. 2022;2(4):283-314. https://doi.org/10.3390/livers2040023.
-
2.
Abou Seif HS. Physiological changes due to hepatotoxicity and the protective role of some medicinal plants. Beni-Suef Univ J Basic Appl Sci. 2016;5(2):134-46. https://doi.org/10.1016/j.bjbas.2016.03.004.
-
3.
Singh A, Bhat TK, Sharma OP. Clinical Biochemistry of Hepatotoxicity. J Clin Toxicol. 2011;4(1):1. https://doi.org/10.4172/2161-0495.s4-001.
-
4.
Willett KL, Roth RA, Walker L. Workshop overview: Hepatotoxicity assessment for botanical dietary supplements. Toxicol Sci. 2004;79(1):4-9. [PubMed ID: 14976355]. https://doi.org/10.1093/toxsci/kfh075.
-
5.
Papay JI, Clines D, Rafi R, Yuen N, Britt SD, Walsh JS, et al. Drug-induced liver injury following positive drug rechallenge. Regul Toxicol Pharmacol. 2009;54(1):84-90. [PubMed ID: 19303041]. https://doi.org/10.1016/j.yrtph.2009.03.003.
-
6.
Balagura G, Iapadre G, Verrotti A, Striano P. Moving beyond sodium valproate: choosing the right anti-epileptic drug in children. Expert Opin Pharmacother. 2019;20(12):1449-56. [PubMed ID: 31099271]. https://doi.org/10.1080/14656566.2019.1617850.
-
7.
Meseguer ES, Elizalde MU, Borobia AM, Ramirez E. Valproic Acid-Induced Liver Injury: A Case-Control Study from a Prospective Pharmacovigilance Program in a Tertiary Hospital. J Clin Med. 2021;10(6). [PubMed ID: 33801850]. [PubMed Central ID: PMC7999396]. https://doi.org/10.3390/jcm10061153.
-
8.
Mishra MK, Kukal S, Paul PR, Bora S, Singh A, Kukreti S, et al. Insights into Structural Modifications of Valproic Acid and Their Pharmacological Profile. Molecules. 2021;27(1). [PubMed ID: 35011339]. [PubMed Central ID: PMC8746633]. https://doi.org/10.3390/molecules27010104.
-
9.
Chang R, Chou MC, Hung LY, Wang ME, Hsu MC, Chiu CH. Study of Valproic Acid-Enhanced Hepatocyte Steatosis. Biomed Res Int. 2016;2016:9576503. [PubMed ID: 27034954]. [PubMed Central ID: PMC4789392]. https://doi.org/10.1155/2016/9576503.
-
10.
Bjornsson E, Olsson R. Suspected drug-induced liver fatalities reported to the WHO database. Dig Liver Dis. 2006;38(1):33-8. [PubMed ID: 16054882]. https://doi.org/10.1016/j.dld.2005.06.004.
-
11.
Vidaurre J, Gedela S, Yarosz S. Antiepileptic Drugs and Liver Disease. Pediatr Neurol. 2017;77:23-36. [PubMed ID: 29097018]. https://doi.org/10.1016/j.pediatrneurol.2017.09.013.
-
12.
Wang K, Zhang S, Marzolf B, Troisch P, Brightman A, Hu Z, et al. Circulating microRNAs, potential biomarkers for drug-induced liver injury. Proc Natl Acad Sci U S A. 2009;106(11):4402-7. [PubMed ID: 19246379]. [PubMed Central ID: PMC2657429]. https://doi.org/10.1073/pnas.0813371106.
-
13.
Sharapova T, Devanarayan V, LeRoy B, Liguori MJ, Blomme E, Buck W, et al. Evaluation of miR-122 as a Serum Biomarker for Hepatotoxicity in Investigative Rat Toxicology Studies. Vet Pathol. 2016;53(1):211-21. [PubMed ID: 26123229]. https://doi.org/10.1177/0300985815591076.
-
14.
Lin X, Chen L, Li H, Liu Y, Guan Y, Li X, et al. miR-155 accelerates proliferation of mouse hepatocytes during liver regeneration by directly targeting SOCS1. Am J Physiol Gastrointest Liver Physiol. 2018;315(4):G443-53. [PubMed ID: 29792529]. https://doi.org/10.1152/ajpgi.00072.2018.
-
15.
Chen W, Han C, Zhang J, Song K, Wang Y, Wu T. Deletion of Mir155 prevents Fas-induced liver injury through up-regulation of Mcl-1. Am J Pathol. 2015;185(4):1033-44. [PubMed ID: 25794705]. [PubMed Central ID: PMC4380848]. https://doi.org/10.1016/j.ajpath.2014.12.020.
-
16.
Hamilton RA, Garnett WR, Kline BJ, Pellock JM. Effects of food on valproic acid absorption. Am J Hosp Pharm. 1981;38(10):1490-3. [PubMed ID: 6794365].
-
17.
Chateauvieux S, Morceau F, Dicato M, Diederich M. Molecular and therapeutic potential and toxicity of valproic acid. J Biomed Biotechnol. 2010;2010. [PubMed ID: 20798865]. [PubMed Central ID: PMC2926634]. https://doi.org/10.1155/2010/479364.
-
18.
Papi A, Ferreri AM, Guerra F, Orlandi M. Anti-invasive effects and proapoptotic activity induction by the rexinoid IIF and valproic acid in combination on colon cancer cell lines. Anticancer Res. 2012;32(7):2855-62. [PubMed ID: 22753748].
-
19.
Xue X, Sun J, Zhang Q, Wang Z, Huang Y, Pan W. Identification and characterization of novel microRNAs from Schistosoma japonicum. PLoS One. 2008;3(12). e4034. [PubMed ID: 19107204]. [PubMed Central ID: PMC2603315]. https://doi.org/10.1371/journal.pone.0004034.
-
20.
Livak KJ, Schmittgen TD. Analysis of relative gene expression data using real-time quantitative PCR and the 2(-Delta Delta C(T)) Method. Methods. 2001;25(4):402-8. [PubMed ID: 11846609]. https://doi.org/10.1006/meth.2001.1262.
-
21.
Pfaffl MW, Horgan GW, Dempfle L. Relative expression software tool (REST) for group-wise comparison and statistical analysis of relative expression results in real-time PCR. Nucleic Acids Res. 2002;30(9). e36. [PubMed ID: 11972351]. [PubMed Central ID: PMC113859]. https://doi.org/10.1093/nar/30.9.e36.
-
22.
Kaplowitz N. Causality assessment versus guilt-by-association in drug hepatotoxicity. Hepatology. 2001;33(1):308-10. [PubMed ID: 11124850]. https://doi.org/10.1053/jhep.2001.21083.
-
23.
Ji Q, Shi X, Lin R, Mao Y, Zhai X, Lin Q, et al. Participation of lipid transport and fatty acid metabolism in valproate sodium-induced hepatotoxicity in HepG2 cells. Toxicol In Vitro. 2010;24(4):1086-91. [PubMed ID: 20371285]. https://doi.org/10.1016/j.tiv.2010.03.014.
-
24.
Kingsley E, Gray P, Tolman KG, Tweedale R. The toxicity of metabolites of sodium valproate in cultured hepatocytes. J Clin Pharmacol. 1983;23(4):178-85. [PubMed ID: 6408143]. https://doi.org/10.1002/j.1552-4604.1983.tb02722.x.
-
25.
Neuman MG, Shear NH, Jacobson-Brown PM, Katz GG, Neilson HK, Malkiewicz IM, et al. CYP2E1-mediated modulation of valproic acid-induced hepatocytotoxicity. Clin Biochem. 2001;34(3):211-8. [PubMed ID: 11408019]. https://doi.org/10.1016/s0009-9120(01)00217-x.
-
26.
Phillips A, Bullock T, Plant N. Sodium valproate induces apoptosis in the rat hepatoma cell line, FaO. Toxicology. 2003;192(2-3):219-27. [PubMed ID: 14580788]. https://doi.org/10.1016/s0300-483x(03)00331-7.
-
27.
Lu TX, Rothenberg ME. MicroRNA. J Allergy Clin Immunol. 2018;141(4):1202-7. [PubMed ID: 29074454]. [PubMed Central ID: PMC5889965]. https://doi.org/10.1016/j.jaci.2017.08.034.
-
28.
Starkey Lewis PJ, Dear J, Platt V, Simpson KJ, Craig DG, Antoine DJ, et al. Circulating microRNAs as potential markers of human drug-induced liver injury. Hepatology. 2011;54(5):1767-76. [PubMed ID: 22045675]. https://doi.org/10.1002/hep.24538.
-
29.
Girard M, Jacquemin E, Munnich A, Lyonnet S, Henrion-Caude A. miR-122, a paradigm for the role of microRNAs in the liver. J Hepatol. 2008;48(4):648-56. [PubMed ID: 18291553]. https://doi.org/10.1016/j.jhep.2008.01.019.
-
30.
Feng X, Bao J, Song C, Xie L, Tan X, Li J, et al. Functional role of miR‑155 in physiological and pathological processes of liver injury (Review). Mol Med Rep. 2021;24(4). [PubMed ID: 34396452]. https://doi.org/10.3892/mmr.2021.12353.
-
31.
Begriche K, Massart J, Robin MA, Borgne-Sanchez A, Fromenty B. Drug-induced toxicity on mitochondria and lipid metabolism: mechanistic diversity and deleterious consequences for the liver. J Hepatol. 2011;54(4):773-94. [PubMed ID: 21145849]. https://doi.org/10.1016/j.jhep.2010.11.006.
-
32.
Schmid MM, Freudenmann RW, Keller F, Connemann BJ, Hiemke C, Gahr M, et al. Non-fatal and fatal liver failure associated with valproic acid. Pharmacopsychiatry. 2013;46(2):63-8. [PubMed ID: 22915484]. https://doi.org/10.1055/s-0032-1323671.
-
33.
Komulainen T, Lodge T, Hinttala R, Bolszak M, Pietila M, Koivunen P, et al. Sodium valproate induces mitochondrial respiration dysfunction in HepG2 in vitro cell model. Toxicology. 2015;331:47-56. [PubMed ID: 25745980]. https://doi.org/10.1016/j.tox.2015.03.001.
-
34.
Kullak-Ublick GA, Andrade RJ, Merz M, End P, Benesic A, Gerbes AL, et al. Drug-induced liver injury: recent advances in diagnosis and risk assessment. Gut. 2017;66(6):1154-64. [PubMed ID: 28341748]. [PubMed Central ID: PMC5532458]. https://doi.org/10.1136/gutjnl-2016-313369.
-
35.
Liu Y, Li P, Liu L, Zhang Y. The diagnostic role of miR-122 in drug-induced liver injury: A systematic review and meta-analysis. Medicine (Baltimore). 2018;97(49). e13478. [PubMed ID: 30544438]. [PubMed Central ID: PMC6310488]. https://doi.org/10.1097/MD.0000000000013478.
-
36.
Singhal R, Harrill AH, Menguy-Vacheron F, Jayyosi Z, Benzerdjeb H, Watkins PB. Benign elevations in serum aminotransferases and biomarkers of hepatotoxicity in healthy volunteers treated with cholestyramine. BMC Pharmacol Toxicol. 2014;15:42. [PubMed ID: 25086653]. [PubMed Central ID: PMC4130124]. https://doi.org/10.1186/2050-6511-15-42.
-
37.
Antoine DJ, Dear JW, Lewis PS, Platt V, Coyle J, Masson M, et al. Mechanistic biomarkers provide early and sensitive detection of acetaminophen-induced acute liver injury at first presentation to hospital. Hepatology. 2013;58(2):777-87. [PubMed ID: 23390034]. [PubMed Central ID: PMC3842113]. https://doi.org/10.1002/hep.26294.
-
38.
Xue X, Wang J, Fu K, Dai S, Wu R, Peng C, et al. The role of miR-155 on liver diseases by modulating immunity, inflammation and tumorigenesis. Int Immunopharmacol. 2023;116:109775. [PubMed ID: 36753984]. https://doi.org/10.1016/j.intimp.2023.109775.
-
39.
Zhou S, Wang Y, Meng Y, Xiao C, Liu Z, Brohawn P, et al. In Vivo Therapeutic Success of MicroRNA-155 Antagomir in a Mouse Model of Lupus Alveolar Hemorrhage. Arthritis Rheumatol. 2016;68(4):953-64. [PubMed ID: 26556607]. https://doi.org/10.1002/art.39485.
-
40.
Blaya D, Aguilar-Bravo B, Hao F, Casacuberta-Serra S, Coll M, Perea L, et al. Expression of microRNA-155 in inflammatory cells modulates liver injury. Hepatology. 2018;68(2):691-706. [PubMed ID: 29420849]. [PubMed Central ID: PMC6082738]. https://doi.org/10.1002/hep.29833.
-
41.
Yuan K, Zhang X, Lv L, Zhang J, Liang W, Wang P. Fine-tuning the expression of microRNA-155 controls acetaminophen-induced liver inflammation. Int Immunopharmacol. 2016;40:339-46. [PubMed ID: 27673475]. https://doi.org/10.1016/j.intimp.2016.09.011.
-
42.
Zhang G, Yan C, Chen D, Wu X, Zhang Y, Zhan Q, et al. Up-regulation of miR-155 contributes to TNF-mediated hepatocyte apoptosis in acute liver failure. Turk J Gastroenterol. 2019;30(5):475-84. [PubMed ID: 31061003]. [PubMed Central ID: PMC6505647]. https://doi.org/10.5152/tjg.2019.18159.
-
43.
Ha SY, Yu JI, Choi C, Kang SY, Joh JW, Paik SW, et al. Prognostic significance of miR-122 expression after curative resection in patients with hepatocellular carcinoma. Sci Rep. 2019;9(1):14738. [PubMed ID: 31611609]. [PubMed Central ID: PMC6791887]. https://doi.org/10.1038/s41598-019-50594-2.
-
44.
Kutay H, Bai S, Datta J, Motiwala T, Pogribny I, Frankel W, et al. Downregulation of miR-122 in the rodent and human hepatocellular carcinomas. J Cell Biochem. 2006;99(3):671-8. [PubMed ID: 16924677]. [PubMed Central ID: PMC3033198]. https://doi.org/10.1002/jcb.20982.
-
45.
Wu X, Wu S, Tong L, Luan T, Lin L, Lu S, et al. miR-122 affects the viability and apoptosis of hepatocellular carcinoma cells. Scand J Gastroenterol. 2009;44(11):1332-9. [PubMed ID: 19891584]. https://doi.org/10.3109/00365520903215305.
-
46.
Sanaei M, Kavoosi F. Effect of Valproic Acid on the Class I Histone Deacetylase 1, 2 and 3, Tumor Suppressor Genes p21WAF1/CIP1 and p53, and Intrinsic Mitochondrial Apoptotic Pathway, Pro- (Bax, Bak, and Bim) and anti- (Bcl-2, Bcl-xL, and Mcl-1) Apoptotic Genes Expression, Cell Viability, and Apoptosis Induction in Hepatocellular Carcinoma HepG2 Cell Line. Asian Pac J Cancer Prev. 2021;22(S1):89-95. [PubMed ID: 33576217]. https://doi.org/10.31557/APJCP.2021.22.S1.89.
-
47.
Wei X, Liu H, Li X, Liu X. Over-expression of MiR-122 promotes apoptosis of hepatocellular carcinoma via targeting TLR4. Ann Hepatol. 2019;18(6):869-78. [PubMed ID: 31477445]. https://doi.org/10.1016/j.aohep.2019.07.005.
-
48.
Chun KH. Molecular Targets and Signaling Pathways of microRNA-122 in Hepatocellular Carcinoma. Pharmaceutics. 2022;14(7). [PubMed ID: 35890276]. [PubMed Central ID: PMC9316959]. https://doi.org/10.3390/pharmaceutics14071380.
-
49.
Chen Y, Shen A, Rider PJ, Yu Y, Wu K, Mu Y, et al. A liver-specific microRNA binds to a highly conserved RNA sequence of hepatitis B virus and negatively regulates viral gene expression and replication. FASEB J. 2011;25(12):4511-21. [PubMed ID: 21903935]. [PubMed Central ID: PMC3236624]. https://doi.org/10.1096/fj.11-187781.
-
50.
Mahmoudian-Sani MR, Asgharzade S, Alghasi A, Saeedi-Boroujeni A, Adnani Sadati SJ, Moradi MT. MicroRNA-122 in patients with hepatitis B and hepatitis B virus-associated hepatocellular carcinoma. J Gastrointest Oncol. 2019;10(4):789-96. [PubMed ID: 31392060]. [PubMed Central ID: PMC6657334]. https://doi.org/10.21037/jgo.2019.02.14.
-
51.
Li C, Wang Y, Wang S, Wu B, Hao J, Fan H, et al. Hepatitis B virus mRNA-mediated miR-122 inhibition upregulates PTTG1-binding protein, which promotes hepatocellular carcinoma tumor growth and cell invasion. J Virol. 2013;87(4):2193-205. [PubMed ID: 23221562]. [PubMed Central ID: PMC3571498]. https://doi.org/10.1128/JVI.02831-12.
-
52.
Song L, Zhang Z, Zhang J, Zhu X, He L, Shi Z, et al. Ratio of microRNA-122/155 in isoniazid-induced acute liver injury in mice. Exp Ther Med. 2016;12(2):889-94. [PubMed ID: 27446292]. [PubMed Central ID: PMC4950840]. https://doi.org/10.3892/etm.2016.3375.
-
53.
Koenig SA, Buesing D, Longin E, Oehring R, Haussermann P, Kluger G, et al. Valproic acid-induced hepatopathy: nine new fatalities in Germany from 1994 to 2003. Epilepsia. 2006;47(12):2027-31. [PubMed ID: 17201699]. https://doi.org/10.1111/j.1528-1167.2006.00846.x.