Abstract
Background:
Due to the unique properties of Astragalus in treating diseases and strengthening the immune system, this study aimed to investigate, for the first time, the immune modulation and apoptosis induction by Astragalus ecbatanus extract against Leishmania tropica.Methods:
The study assessed the in vitro efficacy of the Astragalus ecbatanus extract against both the promastigote and amastigote stages of L. tropica (MHOM/AF/88/KK27). Additionally, the effects of the extract on inducing nitric oxide (NO) release and its cytotoxicity on human macrophage cells were determined by calculating the 50% cytotoxic concentrations (CC50). The study also evaluated the Caspase-3-like activity in extract-treated parasites and conducted quantitative real-time PCR to assess the expression of genes associated with T lymphocytes.Results:
Our study demonstrated that the A. ecbatanus extract significantly (P < 0.001) decreased the viability of both L. tropica promastigote and amastigote forms compared to the negative control. Moreover, the extract exhibited a high selectivity index (> 10), indicating its strong specificity towards intracellular parasites while showing low cytotoxicity to host cells.Conclusions:
The results demonstrated the significant impact of A. ecbatanus ethyl acetate extract on inhibiting and eradicating Leishmania parasites in laboratory settings. While some cellular mechanisms of action were identified, such as immune modulation and apoptosis induction against Leishmania parasites, further investigation is essential to elucidate the specific mechanisms of action and assess the efficacy in animal and human populations.Keywords
1. Background
Leishmaniasis is prevalent among both humans and animals worldwide (1). The disease is spread through the bites of sandflies from the Phlebotomus and Lutzomyia genera. It is a common zoonotic condition that can be transmitted between humans and various animal species, such as dogs and rodents (2). Among the various forms of Leishmaniasis, cutaneous leishmaniasis (CL) is particularly widespread and notably prevalent in countries such as Iran (3, 4). The main synthetic drugs for treatment include pentavalent antimony compounds such as meglumine antimoniate and pentostam. Other medications used in treatment regimens include amphotericin B and paromomycin (5, 6). Glucantime, or meglumine antimoniate (MA), is frequently prescribed. However, its usage is often associated with common adverse effects, especially in patients with hepatic and renal impairments. Furthermore, the high cost and emergence of drug resistance associated with MA have spurred an increase in research efforts aimed at identifying new therapeutic approaches (7, 8).
Astragalus is a medicinal plant known for its potent healing properties, with approximately 3,200 species found worldwide (9). Astragalus thrives in tropical and subtropical regions characterized by low water availability and high humidity. In Iran, there are 800 species of herbaceous plants, shrubs, and bushes, with Astragalus being valued for its fast growth and long-lasting nature (9). Some of the key species used for medicinal purposes include A. kotschyanus, A. ecbatanus, A. chaborasicus, A. teheranicus, A. gummifer labill, and A. ecbatanus (10). These plants are known for their therapeutic properties, such as boosting the immune system, alleviating kidney and bladder pain, preventing diabetes, and possessing antimicrobial and anti-cancer properties (10).
Inflammatory cytokines reportedly play diverse roles in determining susceptibility or resistance, as well as in the immunopathogenesis of the condition (11). Modifications in cytokine levels can influence or serve as markers for the clinical outcome. Notably, inflammatory cytokines of T helper 1 (Th1), specifically interferon-γ, tumor necrosis factor-α, and interleukin-12 (IL-12), are critical in initiating defensive immunity against cutaneous Leishmaniasis (CL). Conversely, Th-2 cytokines such as interleukin-10 (IL-10) and interleukin-13 (IL-13) contribute to parasite persistence by inhibiting the Th1 immune response. Therefore, finding a new drug that strengthens cellular immune responses can be an excellent approach for treating CL.
2. Objectives
This investigation aimed to study the antileishmanial effects, immune modulation, and apoptosis induction by A. ecbatanus extract on L. tropica.
3. Methods
3.1. Plant Materials
In the beginning, specimens of A. ecbatanus aerial components were collected from the elevated regions of Nurabad city in Lorestan Province, Iran, in May 2023. Following botanical verification by a specialist, a specimen was archived at the herbarium of Razi Herbal Medicines Research Center in Khorramabad, Iran, with the designation number LUMS-28354.
3.2. Preparing the Extraction
In the extraction process, 200 grams of the aerial parts of the plant were finely ground and subsequently subjected to maceration using ethyl acetate solvent (Merck, Germany) for 72 hours. The resulting extract was concentrated using a rotary evaporator under vacuum conditions at 50°C and 100 rpm, and then preserved at a temperature of 4°C for further analysis. The obtained extract yield was determined to be 15.60 grams, equivalent to 7.80% weight/volume.
3.3. Analysis of Phytochemicals
A thorough analysis was conducted on the phytochemical makeup of the ethyl acetate extract obtained from the aerial components of A. ecbatanus to detect the presence of various phytochemical compounds, such as flavonoids, tannins, and alkaloids, utilizing the methodologies described in a prior study (9).
3.4. Quantification of Total Phenolics and Flavonoids
The analysis of phenolic and flavonoid compounds in the specimen was conducted using the Folin-Ciocalteau's reagent (Sigma-Aldrich, Germany) colorimetric method and the aluminum chloride (Sigma-Aldrich, Germany) colorimetric method, following established protocols outlined in the literature (10).
3.5. Cell and Parasite
The human macrophage cell lines (THP-1) and L. tropica (MHOM/AF/88/KK27), obtained from the Pasteur Institute, Iran, were cultured in RPMI 1640 medium (Merck, Germany) supplemented with 10% fetal bovine serum (Merck, Germany) and pen-strep (100 mL/IU). The cultures were maintained at 37°C with 5% CO2 for the macrophage cells, and at 23 ± 1°C for L. tropica.
3.6. Effect of Extract on Leishmania tropica Promastigotes
Initially, 100 µL of promastigotes at a concentration of 1 × 106 cells/mL were dispensed into individual wells of a 96-well plate, followed by the addition of 100 µL of varying concentrations (ranging from 6.25 to 200 µg/mL) of the extract to the wells containing the parasites. The plates were then incubated at 24°C for two days. After this incubation period, the supernatant of the wells was aspirated, and 20 μL of thiazolyl blue tetrazolium bromide (MTT) solution (0.5 mg/mL, Sigma-Aldrich, Germany) was introduced into the wells. Following a 4-hour incubation under the same conditions, dimethyl sulfoxide (DMSO, 0.1 mL, Merck, Germany) was added, and the optical density was measured at 570 nm using an enzyme-linked immunosorbent assay (ELISA) plate reader (MR4, Hiperion, Germany) (11).
3.7. Caspase-3-Like Activity of Extract-exposed Parasites
The assessment was conducted using Caspase-3 Colorimetric Activity Assay Kits (Sigma-Aldrich, Germany), following the manufacturer's instructions. In summary, promastigotes at a concentration of 1,000,000 cells/mL were treated with the extract at 1/3, 1/2, and IC50 concentrations for 48 hours. After centrifuging the mixture at 600 rpm and 4°C, the resulting cell pellet was lysed and subjected to further centrifugation at 15,000 rpm for 10 minutes. Following this, 5 μL of the supernatant was combined with 85 μL of buffer and 10 μL of caspase-3 solution, and the mixture was incubated at 37°C for 2 hours. The optical density of the solution at 405 nm was measured using an ELISA reader.
3.8. Effect of Extract on Leishmania tropica Amastigotes
Initially, 100 µL of macrophage cells (100,000 cells/mL) were introduced into a 24-well plate containing rounded coverslips at the bottom and incubated at 37°C in 5% CO2. Subsequently, 1,000,000 stationary phase promastigotes were added to the cells and incubated under the same conditions for one day. After adding different concentrations of the extract and glucantime to the mixture, the wells were incubated for an additional two days. Finally, the prepared slides were stained with Giemsa (Merck, Germany), and 100 macrophages were examined under light microscopy (12).
3.9. Effect of Extract on Nitric Oxide (NO) Release
After exposing THP-1 cells at a concentration of 1×105/mL to varying concentrations (1/3, 1/2, and IC50) of the extract for 48 hours, 0.1 mL of the resulting supernatant was transferred to a 96-well microplate. Subsequently, 0.05 mL of Griess reagent A and B (Sigma-Aldrich, Germany) was added to the designated wells. The optical density of the wells was then assessed at a wavelength of 540 nm using an ELISA reader. Cells exposed to a mixture of lipopolysaccharide (10 ng/mL) and IFN-γ (10 U/mL) were used as the positive control in this experimental setup (13).
3.10. Quantitative Real-time PCR on the Expression of Genes Associated with T Lymphocytes
The study assessed the relative expression levels of inducible nitric oxide synthase (iNOS), interferon gamma (IFN-γ), tumor necrosis factor α (TNFα), and IL-10 in both treated (extract) and untreated macrophage cells through Real-Time PCR analysis. Initially, total RNA was extracted from the samples using the total RNA Extraction Kit from Qiagen, Germany, following the recommended protocol. Subsequently,copy DNA ( cDNA) was synthesized using the Fermentas cDNA synthesis kit from the USA. Quantitative PCR (qPCR) was then conducted using specific primers along with β-actin as previously described by Albalawi et al. (13) (Table 1). The process began with a primary denaturation step at 96°C for 6 minutes, followed by 35 cycles of denaturation at 94°C for 35 seconds and annealing at 75°C for 40 seconds. Finally, data analysis was performed using the 2-ΔΔCt method with the iQTM5 optical system software (Bio-Rad, Hercules, CA).
The Phytochemical Analysis of the Astragalus ecbatanus Methanolic Extract
Compound | Assay | Existance |
---|---|---|
Flavonoids | Ammonia test, alkaline reagent test | ++ |
Polysaccharides | Nitroprusside test | + |
Saponins | Frothing test | + |
Terpenoids | Salkowski test | + |
3.11. Cytotoxic Effects of Extract on THP-1 Macrophage Cells
In this experiment, 100 µL of macrophage cells at a concentration of 1×105/mL were introduced into individual wells of a 96-well plate, followed by the addition of varying concentrations of the extract to each well containing the parasite. The plate was then incubated at 24°C for two days. Subsequently, similar to the promastigote assay, the absorbance of each well was measured using the MTT assay. Furthermore, to assess the cytotoxicity and effectiveness of the extract, the selectivity index (SI) was calculated by dividing the CC50 by the IC50 for amastigotes (13).
3.12. Statistical Analysis
The findings of this research were assessed using SPSS software version 26.0 and one-way analysis of variance (ANOVA). The 50% inhibitory concentrations (IC50) and the 50% cytotoxic concentrations (CC50) were estimated using the Probit analysis. A significance level of P < 0.05 was employed. Each test was conducted in triplicate to ensure accuracy and reliability.
4. Results
4.1. Analysis of Phytochemicals
Analysis of the phytochemical composition confirmed the presence of flavonoids, saponins, terpenoids, and polysaccharides in the extract, as indicated in Table 1. The findings showed that the total phenolic and flavonoid contents were 1.23 mg gallic acid equivalents (GAE) per gram of dry weight (mg GAE/g DW) and 0.59 mg quercetin equivalents per gram of dry weight (mg QE/g DW), respectively.
4.2. Antileishmanial Effects on Promastigote and Amastigote Forms
According to the results obtained from the MTT test, the A. ecbatanus extract significantly (P < 0.001) diminished the rate of L. tropica promastigotes compared with the normal saline control (Figure 1A). Moreover, the IC50 values for A. ecbatanus extract and glucantime were 94.30 μg/mL and 129.62 μg/mL, respectively (Table 2). Based on the findings from the macrophage model, A. ecbatanus extract displayed a dose-dependent decline in the rate of L. tropica amastigotes (Figure 1B). The IC50 values for the extract and glucantime were 43.64 μg/mL and 50.36 μg/mL, respectively (Table 2). There was no significant difference in the antileishmanial effects of the extract and glucantime (P > 0.05).
Antileishmanial activity of methanolic extract of Astragalus ecbatanus and glucantime (MA) on promastigote (A); and amastigote (B) forms Leishmania tropica. Mean ± standard deviation. (n = 3). *P < 0.05, **P < 0.01, and ***P < 0.001 significant difference compared with the negative control.
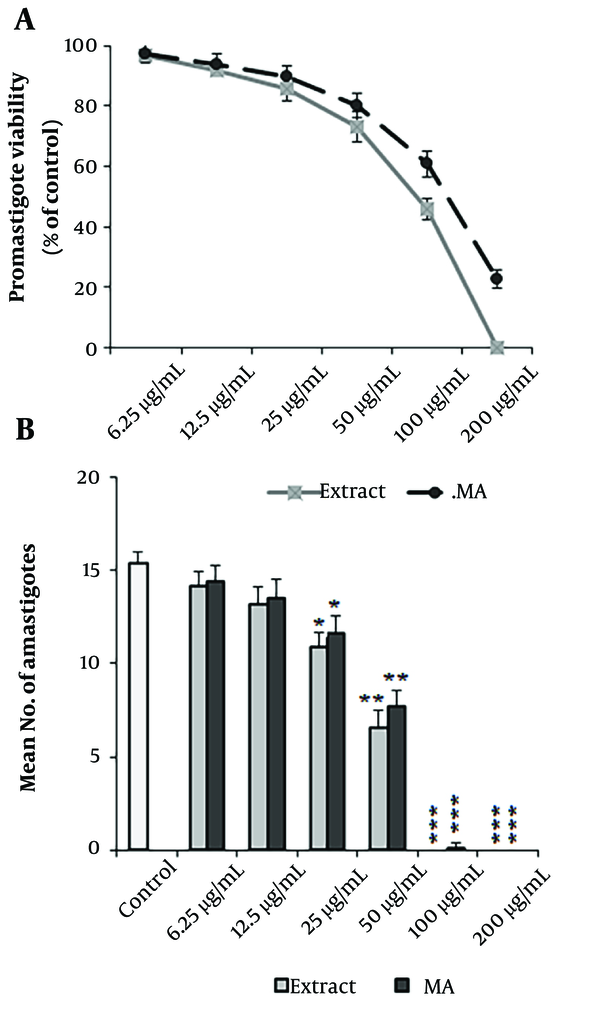
Comparison the 50% Inhibitory Concentrations (IC50) and the 50% Cytotoxic Concentrations (CC50) Values and Selectivity Index (SI) Between Astragalus ecbatanus Ethyl Acetat Extract and Glucantime (n = 3)
Drugs | (µg/mL) | |||
---|---|---|---|---|
Promastigote IC50 | Amastigote IC50 | CC50 | SI | |
A. ecbatanus | 94.30 ± 6.57 | 43.64 ± 3.51 | 485.21 ± 12.33 | 11.2 |
Glucantime | 129.62 ± 7.33 | 50.36 ± 4.12 | 1215.13 ± 15.33 | 24.1 |
4.3. Cytotoxic Effects and SI on THP-1 Macrophage Cells
The CC50 values of the chloroform extract of A. ecbatanus and glucantime were 485.21 μg/mL and 1215.13 μg/mL, respectively (Figure 2). Based on the SI > 10, the extract exhibited high specificity towards intracellular parasites with low cytotoxicity to host cells (Table 2).
Cytotoxicity activity of methanolic extract of Astragalus ecbatanus and glucantime (MA) on human macrophage THP-1 cells. Mean ± standard deviation (n = 3).
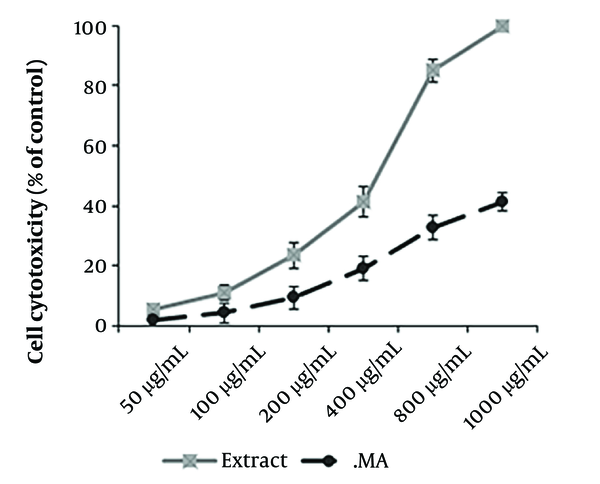
4.4. Caspase-3-Like Activity of Extract-treated Parasites
The findings demonstrated that the A. ecbatanus ethyl acetate extract at concentrations equivalent to 1/2 IC50 and IC50 significantly (P < 0.01) enhanced caspase-3 activation by 16.63% and 24.76%, respectively (Figure 3).
Effect of Astragalus ecbatanus methanolic extract on Caspase-3-like activity of treated promastigotes. Mean ± standard deviation. (n = 3). ** P < 0.01 significant difference compared with the negative control.
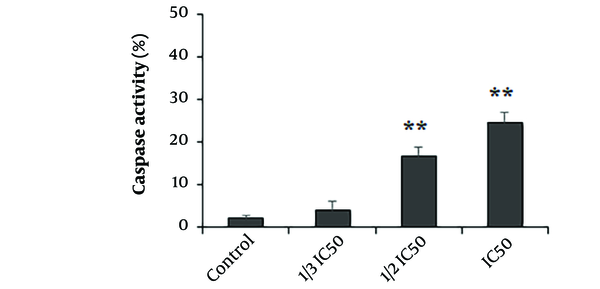
4.5. Effect on NO Release
The results indicated that the THP-1 macrophages exposed to the extract exhibited enhanced production of NO, with a statistically significant increase observed at concentrations equivalent to 1/2 IC50 and IC50 compared to non-treated cells (P < 0.001) (Figure 4).
The effect of Astragalus ecbatanus methanolic extract on the release of nitric oxide (NO) in THP-1 macrophage cells was examined in relation to control groups. The findings are presented as the mean value with standard deviation (n = 3). **P < 0.01 and **P < 0.001 compared to the negative control . IC50: The 50% inhibitory concentrations (IC50).
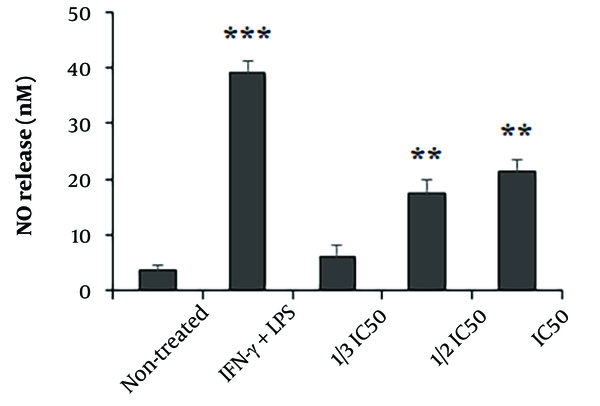
4.6. Quantitative Real-time Polymerase Chain Reaction (PCR) on the Expression of Genes Associated with T Lymphocytes
The results of real-time PCR analysis demonstrated a dose-dependent upregulation of gene expression levels of iNOS, IFN-γ, and TNF-α in macrophages after exposure to A. ecbatanus ethyl acetate extract (P < 0.01). In contrast, the gene expression level of IL-10 showed a dose-dependent downregulation (P < 0.01) after exposure to A. ecbatanus ethyl acetate extract, as illustrated in Figure 5.
Effect of Astragalus ecbatanus methanolic extract on the expression level of genes of related to T lymphocytes. The findings are presented as the mean value with standard deviation (n = 3). *P < 0.05, **P < 0.01; ***P < 0.001 compared to normal saline.
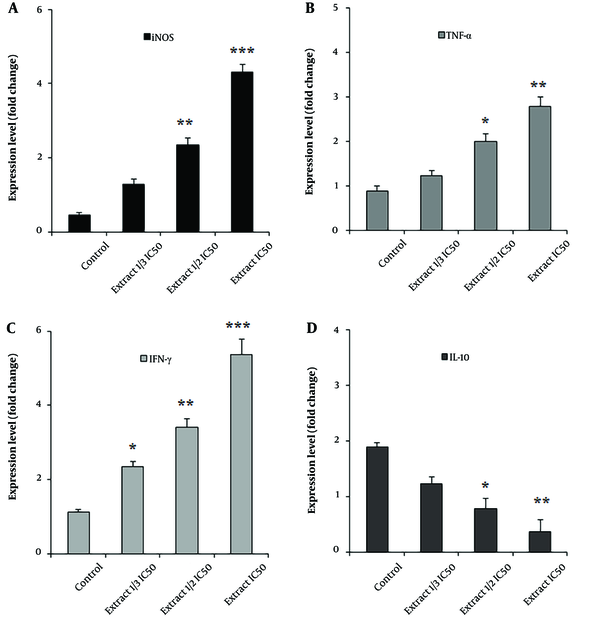
5. Discussion
Our study demonstrated that the A. ecbatanus extract significantly (P < 0.001) decreased the viability of both L. tropica promastigote and amastigote forms compared to the negative control. Moreover, the extract exhibited a high selectivity index (> 10), indicating strong specificity towards intracellular parasites while showing low cytotoxicity to host cells.
In recent times, there has been notable focus among researchers on utilizing medicinal herbs to treat various illnesses. The extensive availability, low cost, minimal side effects, high efficacy, and antimicrobial effects are key factors driving the use of natural products (9). Plants of the Astragalus genus possess therapeutic properties, including boosting the immune system, relieving kidney and bladder pain, preventing diabetes, and exhibiting antimicrobial and anti-cancer effects (10). Given the distinctive medicinal qualities of Astragalus species in disease treatment and immune system enhancement, this study is the first to investigate the immune modulation and apoptosis induction by A. ecbatanus ethyl acetate extract against L. tropica.
Our results showed that the A. ecbatanus extract markedly reduced the rate of L. tropica promastigote and amastigote forms compared with the negative control. Based on the SI > 10, it was found that the extract exhibited high specificity to intracellular parasites with low cytotoxicity to host cells. Numerous investigations have been conducted both in vitro and in vivo to explore the potential antileishmanial properties of various herbs and their derivatives (14). However, the outcomes of these studies have shown inconsistent effectiveness due to a lack of precise understanding of the underlying mechanisms. Therefore, more thorough and accurate research is needed in this area (15).
Several studies have examined the antimicrobial properties of Astragalus species against a range of pathogenic microorganisms, including bacteria (both gram-negative and gram-positive), fungi such as Aspergillus spp. and Candida species, viruses, and parasitic strains such as Eimeria papillata and Toxoplasma gondii (16-18). Nevertheless, to the best of our knowledge, there is a lack of documented research on the antileishmanial effects of A. ecbatanus. In the study conducted by Mahmoudvand et al., the results indicated that the A. ecbatanus chloroform extract, particularly when used at a concentration of 45 mg/mL, demonstrated significant in vitro and ex vivo anti-parasitic effects against Echinococcus granulosus protoscoleces. They also showed that following exposure to this extract, the levels of caspase-3 enzyme activity and plasma membrane permeability in protoscoleces showed a dose-dependent increase (19). We confirmed the presence of saponins, flavonoids, terpenoids, and polysaccharides in the extract. Studies have demonstrated that phenolic and flavonoid compounds are the predominant phytochemicals found in the genus Astragalus (20). Various studies have indicated that these compounds can impede nucleic acid synthesis, disrupt cytoplasmic and cell membrane functions, induce apoptosis, alter membrane permeability, inhibit pathogenicity, and exhibit synergistic effects with existing agents to inhibit and eradicate microbial pathogens (20, 21). Therefore, it is plausible to propose that the potential antileishmanial properties of A. ecbatanus are attributed to the presence of these phytochemicals.
According to previous studies, inflammatory cytokines play various roles in determining susceptibility or resistance, as well as in the immunopathogenesis of the condition (22). The levels of cytokines and their balance can impact clinical outcomes and may serve as markers for disease progression. Specifically, Th1 cytokines, such as IFN-γ, TNF-α, and IL-12, are essential for initiating defensive immunity against CL. Conversely, Th2 cytokines such as IL-10 and IL-13 can inhibit the Th1 immune response, leading to parasite persistence (22). Additionally, nitric oxide (NO) is widely recognized as a key element in the innate immune response for regulating and eradicating intracellular pathogens such as Leishmania spp (23).
Our results indicated a dose-dependent upregulation of the expression levels of genes iNOS, IFN-γ, and TNF-α in macrophages after exposure to the ethyl acetate extract of A. ecbatanus. In contrast, the gene expression level of IL-10 exhibited a dose-dependent downregulation after exposure to the A. ecbatanus ethyl acetate extract. We also found that the THP-1 macrophages exposed to the ethyl acetate extract of A. ecbatanus exhibited enhanced production of NO. Therefore, we suggest that this extract can control L. tropica parasites through immune modulation.
Apoptosis is recognized as a critical mechanism that connects an organism's survival to its ability to initiate programmed cell death (24). Caspases play a central role in this process, with Caspase-3 being widely acknowledged as the primary caspase responsible for activating death proteases and subsequently initiating cell death. In recent years, the induction of apoptosis has been identified as a promising antimicrobial mechanism of various drugs under investigation (25). Consequently, we evaluated the Caspase-3-like activity of parasites following exposure to the ethyl acetate extract of A. ecbatanus. Our findings clearly demonstrate that the ethyl acetate extract of A. ecbatanus significantly enhances caspase-3 activation in Leishmania parasites, suggesting that the extract induces apoptosis and inhibits the growth rate of Leishmania parasites.
5.1. Conclusions
The results demonstrate the significant impact of A. ecbatanus ethyl acetate extract on inhibiting and eradicating Leishmania parasites in laboratory settings. While some cellular mechanisms of action were identified, such as immune modulation and apoptosis induction against Leishmania parasites, further examination is essential to elucidate the specific mechanisms of action and efficacy in animal and human populations.
References
-
1.
Monzote L. Current Treatment of Leishmaniasis: A Review. Open Antimicrob Age J. 2009;1(1):9-19. https://doi.org/10.2174/1876518100901010009.
-
2.
Torres-Guerrero E, Quintanilla-Cedillo MR, Ruiz-Esmenjaud J, Arenas R. Leishmaniasis: a review. F1000Res. 2017;6:750. [PubMed ID: 28649370]. [PubMed Central ID: PMC5464238]. https://doi.org/10.12688/f1000research.11120.1.
-
3.
Albalawi AE, Alanazi AD, Sharifi I, Ezzatkhah F. A Systematic Review of Curcumin and its Derivatives as Valuable Sources of Antileishmanial Agents. Acta Parasitol. 2021;66(3):797-811. [PubMed ID: 33770343]. https://doi.org/10.1007/s11686-021-00351-1.
-
4.
Oliveira LF, Schubach AO, Martins MM, Passos SL, Oliveira RV, Marzochi MC, et al. Systematic review of the adverse effects of cutaneous leishmaniasis treatment in the New World. Acta Trop. 2011;118(2):87-96. [PubMed ID: 21420925]. https://doi.org/10.1016/j.actatropica.2011.02.007.
-
5.
Ríos JL, Ríos JL, Waterman PG. A review of the pharmacology and toxicology of Astragalus. Phytotherapy Research. 1997;11(6). https://doi.org/10.1002/(SICI)1099-1573(199709)11:6<411::AID-PTR132>3.0.CO;2-6.
-
6.
Li X, Qu L, Dong Y, Han L, Liu E, Fang S, et al. A review of recent research progress on the astragalus genus. Molecules. 2014;19(11):18850-80. [PubMed ID: 25407722]. [PubMed Central ID: PMC6270929]. https://doi.org/10.3390/molecules191118850.
-
7.
Shahrajabian MH. A Review of Astragalus Species as Foodstuffs, Dietary Supplements, a Traditional Chinese Medicine and a Part of Modern Pharmaceutical Science. Applied Ecology and Environmental Research. 2019;17(6). https://doi.org/10.15666/aeer/1706_1337113382.
-
8.
Niazi M, Saki M, Sepahvand M, Jahanbakhsh S, Khatami M, Beyranvand M. In vitro and ex vivo scolicidal effects of Olea europaea L. to inactivate the protoscolecs during hydatid cyst surgery. Ann Med Surg (Lond). 2019;42:7-10. [PubMed ID: 31193397]. [PubMed Central ID: PMC6527941]. https://doi.org/10.1016/j.amsu.2019.04.006.
-
9.
Ezatpour B, Saedi Dezaki E, Mahmoudvand H, Azadpour M, Ezzatkhah F. In Vitro and In Vivo Antileishmanial Effects of Pistacia khinjuk against Leishmania tropica and Leishmania major. Evid Based Complement Alternat Med. 2015;2015:149707. [PubMed ID: 25815025]. [PubMed Central ID: PMC4359887]. https://doi.org/10.1155/2015/149707.
-
10.
Albalawi AE, Khalaf AK, Alyousif MS, Alanazi AD, Baharvand P, Shakibaie M, et al. Fe3O4(@)piroctone olamine magnetic nanoparticles: Synthesize and therapeutic potential in cutaneous leishmaniasis. Biomed Pharmacother. 2021;139:111566. [PubMed ID: 33839494]. https://doi.org/10.1016/j.biopha.2021.111566.
-
11.
Scott P, Novais FO. Cutaneous leishmaniasis: immune responses in protection and pathogenesis. Nat Rev Immunol. 2016;16(9):581-92. [PubMed ID: 27424773]. https://doi.org/10.1038/nri.2016.72.
-
12.
Mahmoudvand H, Ezzatkhah F, Sharififar F, Sharifi I, Dezaki ES. Antileishmanial and cytotoxic effects of essential oil and methanolic extract of Myrtus communis L. Korean J Parasitol. 2015;53(1):21-7. [PubMed ID: 25748705]. [PubMed Central ID: PMC4384785]. https://doi.org/10.3347/kjp.2015.53.1.21.
-
13.
Albalawi AE, Abdel-Shafy S, Khudair Khalaf A, Alanazi AD, Baharvand P, Ebrahimi K, et al. Therapeutic Potential of Green Synthesized Copper Nanoparticles Alone or Combined with Meglumine Antimoniate (Glucantime((R))) in Cutaneous Leishmaniasis. Nanomaterials (Basel). 2021;11(4). [PubMed ID: 33807273]. [PubMed Central ID: PMC8065924]. https://doi.org/10.3390/nano11040891.
-
14.
Tajbakhsh E, Khamesipour A, Hosseini SR, Kosari N, Shantiae S, Khamesipour F. The effects of medicinal herbs and marine natural products on wound healing of cutaneous leishmaniasis: A systematic review. Microb Pathog. 2021;161(Pt A):105235. [PubMed ID: 34648927]. https://doi.org/10.1016/j.micpath.2021.105235.
-
15.
Gharirvand Eskandari E, Setorki M, Doudi M. Medicinal Plants With Antileishmanial Properties: A Review Study. Pharmaceutical and Biomedical Research. 2020. https://doi.org/10.18502/pbr.v6i1.3422.
-
16.
Mikaeili A, Karimi I, Shamspur T, Gholamine B, Modaresi M, Khanlari A. Anti-candidal activity of Astragalus verus in the in vitro and in vivo guinea pig models of cutaneous and systemic candidiasis. Rev Bras Farmacogn. 2012;22(5):1035-43. https://doi.org/10.1590/s0102-695x2012005000035.
-
17.
Kanaan H, El-Mestrah M, Sweidan A, As-Sadi F, Bazzal AA, Chokr A. Screening for antibacterial and antibiofilm activities in Astragalus angulosus. J Intercult Ethnopharmacol. 2017;6(1):50-7. [PubMed ID: 28163960]. [PubMed Central ID: PMC5289088]. https://doi.org/10.5455/jice.20161018101720.
-
18.
Khan MN, Ahmed M, Khan MW, Khan RA. In vitro pharmacological effects of Astragalus eremophilus and Melilotus parviflora. Acta Biol Hung. 2018;69(4):411-22. [PubMed ID: 30587023]. https://doi.org/10.1556/018.69.2018.4.4.
-
19.
Mahmoudvand H, Al-Abodi HR, Zolfagharkhani P, Ghasemian Yadegari J. Anti-helminthic effects and cellular mechanisms of Astragalus ecbatanus extract against Echinococcus granulosus protoscoleces. J Parasit Dis. 2022;46(4):1047-54. [PubMed ID: 36457771]. [PubMed Central ID: PMC9606165]. https://doi.org/10.1007/s12639-022-01517-y.
-
20.
Miklasinska-Majdanik M, Kepa M, Wojtyczka RD, Idzik D, Wasik TJ. Phenolic Compounds Diminish Antibiotic Resistance of Staphylococcus Aureus Clinical Strains. Int J Environ Res Public Health. 2018;15(10). [PubMed ID: 30360435]. [PubMed Central ID: PMC6211117]. https://doi.org/10.3390/ijerph15102321.
-
21.
Panche AN, Diwan AD, Chandra SR. Flavonoids: an overview. J Nutr Sci. 2016;5. e47. [PubMed ID: 28620474]. [PubMed Central ID: PMC5465813]. https://doi.org/10.1017/jns.2016.41.
-
22.
Miklasinska-Majdanik M, Kepa M, Wojtyczka RD, Idzik D, Wasik TJ. Phenolic Compounds Diminish Antibiotic Resistance of Staphylococcus Aureus Clinical Strains. Int J Environ Res Public Health. 2018;15(10). [PubMed ID: 30360435]. [PubMed Central ID: PMC6211117]. https://doi.org/10.3390/ijerph15102321.
-
23.
Maspi N, Abdoli A, Ghaffarifar F. Pro- and anti-inflammatory cytokines in cutaneous leishmaniasis: a review. Pathog Glob Health. 2016;110(6):247-60. [PubMed ID: 27660895]. [PubMed Central ID: PMC5070640]. https://doi.org/10.1080/20477724.2016.1232042.
-
24.
Panaro MA, Brandonisio O, Sisto M, Acquafredda A, Leogrande D, Fumarola L, et al. Nitric oxide production by Leishmania-infected macrophages and modulation by prostaglandin E2. Clin Exp Med. 2001;1(3):137-43. [PubMed ID: 11833850]. https://doi.org/10.1007/s10238-001-8025-0.
-
25.
Savitskaya MA, Onishchenko GE. Mechanisms of Apoptosis. Biochemistry (Mosc). 2015;80(11):1393-405. [PubMed ID: 26615431]. https://doi.org/10.1134/S0006297915110012.
-
26.
Van Opdenbosch N, Lamkanfi M. Caspases in Cell Death, Inflammation, and Disease. Immunity. 2019;50(6):1352-64. [PubMed ID: 31216460]. [PubMed Central ID: PMC6611727]. https://doi.org/10.1016/j.immuni.2019.05.020.