Abstract
Background:
Developing drugs with higher efficacy and fewer side effects for psychological disorders has always been of interest. The rhizome of Polypodium vulgare L. (common polypody) has been used as a mood stabilizer in Persian Medicine (PM).Objectives:
The present study evaluates the anxiolytic and antidepressant effects of the hydroethanolic extract of common polypody rhizome (PEE) and the possible underlying mechanisms in mice subjected to chronic restraint stress (CRS).Methods:
Sixty NMRI male mice were exposed to CRS and received either a vehicle, fluoxetine, or PEE (at doses of 250, 500, 1000, and 1500 mg.kg-1). The forced swim test (FST), tail suspension test (TST), open-field test (OFT), and elevated-plus maze (EPM) were conducted to evaluate depressive-like and anxiety-like behavior. Oxidative, inflammatory, and apoptotic markers, as well as brain-derived neurotrophic factor (BDNF), were measured in the prefrontal cortex (PFC) and hippocampus (HIP). Corticosterone levels were also assessed in serum samples.Results:
Polypody rhizome increased the time spent in the central zone of the OFT, as well as the time spent and the number of entries into the open arms of the EPM, while it decreased the immobility time in the FST and TST. Administration of PEE caused a significant decline in serum levels of corticosterone, as well as tissue levels of tumor necrosis factor-alpha (TNF-α), interleukin-1 beta (IL-1β), and malondialdehyde (MDA). Additionally, it increased superoxide dismutase (SOD) activity in the PFC and HIP. Polypody rhizome also significantly restored total antioxidant capacity (TAC), glutathione peroxidase (GPx) activity, and BDNF expression in the HIP. Moreover, Bcl-2 levels increased in the PFC, and Bax and caspase-3 expressions were downregulated in both regions with PEE administration.Conclusions:
Polypody rhizome exerted anxiolytic and antidepressant effects in behavioral tests and reversed CRS-induced oxidative, inflammatory, and apoptotic alterations.Keywords
Polypodium vulgare Depression Inflammation Apoptosis Antioxidant Persian Medicine
1. Background
Major depression is a potentially life-threatening mental condition that may be accompanied by episodes of anxiety. It is characterized by low mood, loss of enjoyment and satisfaction, disturbed appetite and sleep, and undesirable alterations in cognition and psychomotor functions (1, 2). Based on estimations of the World Health Organization (WHO), depression will rank as the second global burden of disease by 2030 (3).
Although the underlying mechanisms of these disorders remain elusive, several clinical and preclinical investigations have illustrated the possible role of inflammatory, oxidative, and apoptotic pathways in the prefrontal cortex (PFC) and hippocampus (HIP), leading to neuroinflammation and neurodegeneration in these areas, which are imperative for behavioral and emotional processing (1, 4). Furthermore, the excessive activation of the hypothalamic-pituitary-adrenal (HPA) axis, the central component of the neuroendocrine system involved in the stress response, has been associated with the development of anxiety and depression. This dysregulation leads to elevated levels of glucocorticoid secretion (5, 6).
Chronic stress, considered a significant factor in the development of anxiety and depressive disorders, leads to increased levels of pro-inflammatory cytokines, such as interleukin-1 beta (IL-1β) and tumor necrosis factor-alpha (TNF-α), and down-regulation of antioxidant enzymatic scavengers, including superoxide dismutase (SOD) and glutathione peroxidase (GPx). This process is followed by dysregulation of apoptotic mediators, including Bcl-2 and caspase families (1, 2, 7-9). Additionally, one of the important growth factors involved in neurogenesis and neuroplasticity, brain-derived neurotrophic factor (BDNF), is downregulated during chronic stress exposure (2, 4).
The popularity of complementary and alternative medicine (CAM), particularly herbal products, for mental disorders has increased in recent years. This is, in part, due to multiple complications of current anxiolytic and antidepressant medications, such as physical and emotional side effects, sexual dysfunction, and tolerance in long-term use (10, 11).
Polypodium vulgare L. (common polypody) belongs to the Polypodiaceae family. This fern species grows on banks and rocky humid places in North America, Europe, and some parts of Asia, including northern regions of Iran (12-14). The rhizome of common polypody has traditionally been used for digestive ailments, respiratory diseases, inflammatory conditions (12, 15-17), psychiatric disorders, and tonic effects in ancient medical systems, including Persian Medicine (PM) (18, 19).
Antimicrobial (20, 21), antioxidant (22, 23), and neuro-functional properties (12, 24, 25) have been investigated in different parts of common polypody in previous studies. Cytotoxicity along with cytoprotective activity of its fronds against different cell lines were studied. According to phytochemical analysis, the current effects could be related to its high contents of polyphenolic compounds (22). Major phytochemical constituents of common polypody include triterpenes, flavonoids, saponins, phytoecdysteroids, phloroglucinol, volatile oil, and fixed oil (12, 14, 26, 27).
2. Objectives
Based on the neuromodulatory activities and related mechanisms of action of some of the mentioned bioactive metabolites (19, 28-30), the aim of the current study is to examine the anxiolytic and antidepressant effects of the hydroethanolic extract of common polypody rhizome (PEE) in stressed mice using experimental behavioral tests and biochemical parameters.
3. Methods
3.1. Chemicals
Fluoxetine was purchased from Abidi-Pharma Co. (Tehran, Iran). Tween 80 - 1% (Merck KGaA, Darmstadt, Germany) in distilled water was used as a solvent for herbal extraction. Ketamine 10% and xylazine 2% were purchased from Alfasan (Woerden, Holland). Total antioxidant capacity (TAC), malondialdehyde (MDA), SOD, and GPx assay kits were obtained from Navand Lab Kit (Navand Salamat Co., Urmia, West Azerbaijan Province, Iran). Corticosterone (KGE009, R & D Systems, Inc., USA), TNF-α (DY410, DuoSet ELISA, Development System), and IL-1β (Dy401) assay kits were used for inflammatory measurements. Primary antibodies, including anti-BDNF [(EPR1292) ab108319, Abcam, USA], anti-Bax (sc-7480, Santa Cruz Biotechnology, Inc., USA), anti-Bcl-2 (sc-492), anti-caspase-3 (sc-7272), anti-β-actin (1: 300, sc-47778) as a loading control, and horseradish peroxidase-conjugated (HRP) mouse anti-rabbit IgG secondary antibody (1: 1000, sc-2357) were obtained for immunoblotting assessments.
3.2. Herbal Analysis
3.2.1. Hydroethanolic Extraction
Common polypody rhizomes were purchased from a herbal center in Tehran, Iran, and authenticated at the Department of Pharmacognosy, Faculty of Pharmacy, Shahid Sadoughi University of Medical Sciences, Yazd, Iran (Voucher No.: SSU0063). After grinding and sieving (mesh size: 18 - 32) the rhizome, hydroethanolic extract was prepared with ethanol/distilled water (70:30) based on the percolation method (31). This liquid extract was dried by a rotary evaporator and then stored in an airtight container inside the refrigerator (4°C) until further analysis.
3.2.2. Measurement of Total Phenolic and Flavonoid Content
The total phenolic compounds in PEE were assessed based on the Folin-Ciocalteu method with some modifications. Briefly, a volume of 200 µL of the extract (1 mg/mL) was mixed with 1.5 mL Folin-Ciocalteu reagent and left at room temperature for 5 minutes. Subsequently, 1.5 mL Na2CO3 6% was added to the solution. Finally, the solution was incubated at 25°C for 90 minutes, and the absorbance was measured at 725 nm by a spectrophotometer (Unico Shanghai Co., China). Total phenolic content was calculated from the linear equation of a standard curve (Y = 0.0049X - 0.0016, R2 = 0.9979) and expressed as mg/g Gallic acid equivalents (GAE) of plant weight. To calculate the flavonoid content based on the aluminum chloride colorimetric assay method, a volume of 1 mL of the extract (1 mg/mL) was mixed initially with 300 µL NaNO2 5%- and 4-mL distilled water. The mixture was left at room temperature for 5 minutes and then mixed with 300 µL AlCl3 10% and 2 mL NaOH 1M. The absorbance was measured at 510 nm. The total flavonoid content was obtained from the calibration curve using the following linear fit (Y = 0.0025X - 0.1495, R2 = 0.9975) and presented as mg/g quercetin equivalents (QE) of plant weight (32).
3.2.3. Physicochemical Assessments
3.2.3.1. Total and Acid-Insoluble Ash
Weighed in a porcelain crucible, 2.28 g of the powdered common PEE was placed in a muffle furnace (M.L.F., Fanavaran Co., Tehran, Iran) at 600 - 800°C for 4 hours. The obtained ash was weighed after cooling and presented as a percentage of air-dried material to obtain the total ash. Twenty-five mL of hydrochloric acid was added to the porcelain crucible containing the total ash, which was then heated and filtered using ash-less filter paper. Subsequently, the filter paper was washed with hot water, re-inserted into the original crucible, and transferred into the muffle furnace at 450°C for 4 hours. The amount of acid-insoluble ash was calculated as mg.g-1 (31).
3.2.3.2. Moisture Values
Moisture content was measured using the oven-drying method (31). Accordingly, 5 g of the powdered rhizome was placed in an air oven at 100 - 115°C for 2 hours. After cooling, the sample was weighed and replaced in the oven for 15 minutes. This process was repeated until weight was constant in two consecutive measures. The moisture value was measured as the amount of material weight loss.
3.3. Experimental Design
3.3.1. Animals
Sixty NMRI adult male mice (6 - 8 weeks old, 25 - 30 g), were purchased from Royan Institute, Karaj, Iran, and housed five per cage under standard colony conditions (temperature 24 ± 1°C, 12 h light/dark cycle) with unlimited access to food and water. Following a week of acclimation, the mice were randomly divided into eight groups as follows: Group (a) was considered the non-stressed control group; group (b) was exposed to chronic restraint stress (CRS); group (c) underwent CRS and received the vehicle (1% tween 80 in distilled water); group (d) underwent CRS and received fluoxetine (20 mg.kg-1), and the four following groups were exposed to CRS and received different concentrations of PEE: (e) CRS + PEE (250 mg.kg-1); (f) CRS + PEE (500 mg/kg); (g) CRS + PEE (1000 mg.kg-1); (h) CRS + PEE (1500 mg/kg).
3.3.2. Chronic Restraint Stress and Treatment
Each mouse was carefully placed inside a well-ventilated 50 mL Falcon tube, equipped with 12 holes, for six hours a day for 14 consecutive days. Animals were deprived of food and water during the restraint period. Body weight was measured on days 1, 7, and 14 during the chronic treatment regimen. All solutions were prepared freshly every day and orally administered to subjects prior to stress induction. The behavioral tasks were carried out the day after the last stress session (9).
3.4. Behavioral Tests
3.4.1. Open-Field Test
In order to determine locomotor activity, one mouse was placed in the corner of a white open-field apparatus (72 cm × 72 cm × 40 cm) with sixteen black-bordered squares at the base and a red one in the central zone. The mouse had the opportunity to freely explore the field for 5 minutes. During this period, the number of times the black lines were crossed and the time spent in the central zone were recorded (7).
3.4.2. Elevated-Plus Maze
The device consists of a couple of crossing open and closed arms (30 cm × 5 cm) in a plus-shaped maze. Both lateral sides and the endpoints of the closed arms were enclosed with walls measuring 15 cm in height, while the open arms were surrounded by a 0.5 cm high edge to prevent the animal from falling. The mice were placed at the intersection zone facing toward the open arms and allowed to freely move and explore for 30 seconds, and the next 5 minutes were set as the test time. The percentage of open arm entries (%OAE) and the percentage of time spent in the open arms (%OAT) were calculated (32).
3.4.3. Forced Swim Test
Each mouse was forced to swim in a cylindrical container (30 cm in depth, 12 cm in diameter) filled with fresh water (23 - 25°C, 20 cm in depth). After an initial 2 minutes of adaptation, immobility time during the next 4 minutes was recorded (8).
3.4.4. Tail Suspension Test
Mice were separately hung upside-down, 50 cm above the floor, by fixing the tail with adhesive tape for 6 minutes. Immobility time was recorded during the last 4 minutes of the test (32).
3.5. Biochemical Assays
3.5.1. Sampling
To collect tissue samples, the mice were anesthetized by administering an intraperitoneal injection of ketamine (90 mg/kg) and xylazine (10 mg/kg), 24 hours following the final behavioral test. To obtain serum, blood samples were collected directly from the heart and subsequently centrifuged at a speed of 3500 × g for 12 minutes. The brains were immediately dissected. Prefrontal cortex and HIP samples were removed and frozen at -80°C along with serum samples for further analysis.
3.5.2. Antioxidant and Lipid Peroxidation Measurement
Frozen PFC and HIP samples were homogenized with phosphate-buffered saline (PBS), centrifuged, and the resulting supernatant was collected for the following assessments. Total antioxidant capacity was measured according to the ferric reducing antioxidant power (FRAP) method, and absorbance was measured at 593 nm by spectrophotometer (33). The concentration of MDA, as a biomarker of lipid peroxidation, was calculated by thiobarbituric acid reactive substances (TBARS) calorimetric. The pink chromogen was measured at 550 nm (8). Superoxide dismutase activity was measured via inhibition of pyrogallol autoxidation reaction, and the absorbance was read at 405 nm. Glutathione peroxidase activity was evaluated based on NADPH oxidation accompanied by a decrease in absorbance at 340 nm (34).
3.5.3. Enzyme-Linked Immunosorbent Assay
The plasma levels of corticosterone and tissue levels of TNF-α and IL-1β in PFC and HIP supernatant samples were determined using enzyme-linked immunosorbent assay (ELISA) kits. The measurements were conducted following the manufacturer's protocol for the respective ELISA kits (6).
3.5.4. Western-Blotting
The immunoblotting method was employed to evaluate protein expression in PFC and HIP. Briefly, the frozen tissues were lysed and homogenized using Radio Immuno-Precipitation Assay (RIPA) buffer containing a protease inhibitor cocktail and EDTA, and then centrifuged at 12,000 × g for 10 minutes at 4°C. The Bradford method was used to estimate the concentration of proteins in the obtained supernatant. Subsequently, equal amounts of protein samples were loaded onto an SDS-polyacrylamide gel and electrophoretically transferred onto polyvinylidene difluoride (PVDF) membranes, which were blocked by incubation with non-fat dry milk 2% (NFDM) in tris-buffered saline with tween 20 (TBST) for 75 minutes with rotation at room temperature to avoid non-specific binding reactions. Then, the membranes were probed overnight with the following primary antibodies: Anti-BDNF, anti-Bax, anti-Bcl-2, anti-caspase-3, and anti-β-actin as a loading control. Subsequently, the blots were incubated with HRP-conjugated mouse anti-rabbit IgG secondary antibody for 75 minutes at room temperature. Finally, immunoreactive protein bands were developed using ECL detection reagents (Pierce Biotechnology, Rockford, IL 61105 USA), quantified via densitometry with ImageJ (v1.46d, National Institute of Health, USA) software, and normalized to the matching internal control (7).
3.6. Statistical Analysis
The data were reported as mean ± standard error of the mean (S.E.M). One-way or two-way analysis of variance (ANOVA), followed by a post-hoc Tukey test by Graph Pad Prism (version 5.00, Graph Pad Software Inc, La Jolla, CA, USA), was used for statistical evaluation. Results were considered statistically significant when P < 0.05.
4. Results
4.1. Total Phenolic and Flavonoid Contents
According to the extraction method, the total yield was 27.55%. The total phenolic content was 337.45 mg GAE/g in PEE, equivalent to 93 mg GAE/g of plant weight (Figure 1A). Moreover, the total flavonoid content in PEE was 628.24 mg QE/g, which was 173 mg QE/g of plant weight (Figure 1B).
A, analysis of total phenolic; and B, flavonoid contents of common polypody rhizome (PEE)
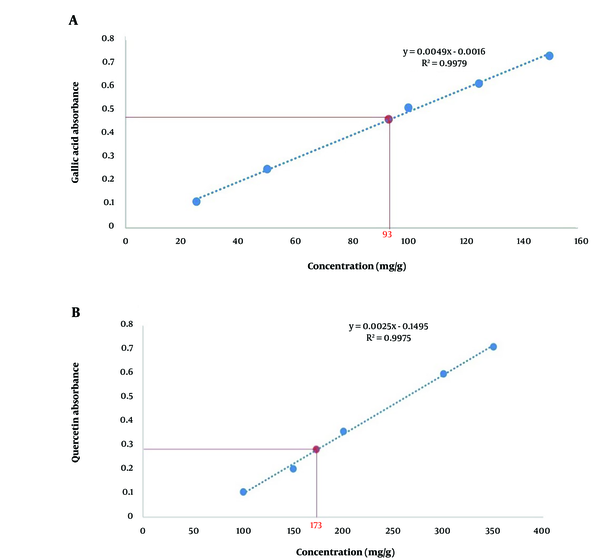
4.2. Total Ash, Acid-Insoluble Ash, and Moisture Value
The total ash and acid-insoluble ash in the common PEE were 3.91% and 0.01 mg/g of plant weight, respectively. Moreover, the moisture value was 1.03% as the index of material weight loss.
4.3. Effects of PEE on Anxiety and Depressive-Like Behaviors and Body Weight
The results of the OFT indicated that there was no significant difference in the number of line crossings among the groups (Figure 2A). However, CRS significantly decreased the time spent in the center compared to the control group (P < 0.01, Figure 2B). Nevertheless, PEE at doses of 500, 1000, and 1500 mg/kg significantly increased the time spent in the center compared to the stress group (P < 0.01, P < 0.05, P < 0.01, respectively). As demonstrated in Figures 2C, D, both %OAE and %OAT were significantly reduced in the CRS group compared to the control group (P < 0.01). Nevertheless, PEE at a dose of 1500 mg/kg significantly increased both %OAE and %OAT compared to the CRS group (P < 0.05). Moreover, PEE significantly increased %OAT at a dose of 1000 mg/kg compared to the CRS group (P < 0.01). Fluoxetine also significantly increased %OAT compared to the CRS group (P < 0.05).
A, effects of PEE on number of crossings; and B, the time spent in the central zone in OFT; C, OAE%; and D, OAT% in EPM; E, immobility time in FST; and F, TST; and G, body weight after the two weeks of restraint stress exposure. Data are presented as mean± S.E.M (n = 6 - 8/group); * P < 0.05, ** P < 0.01, *** P < 0.001, **** P < 0.0001 vs Ctrl group; # P < 0.05, ## P < 0.01 vs CRS group. (PEE, hydroethanolic extract of common polypody rhizome; OFT, open-field test; EPM, elevated-plus maze; OAE%, percentage of open arm entries; OAT%, percentage of time spent in the open arms; FST, forced swim test; TST, tail suspension test; CRS, chronic restraint stress; Ctrl, control; FLX, fluoxetine).
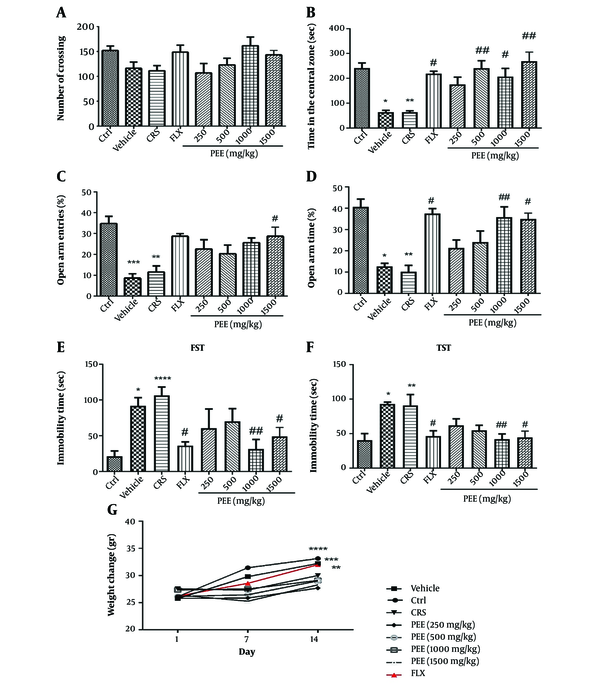
Based on the one-way ANOVA analysis, there was a significant difference in immobility time among CRS and PEE-treated groups in the FST (Figure 2E) and TST (Figure 2F). Post-hoc analysis demonstrated that chronic stress exposure significantly increased immobility time compared with the control group in both FST and TST. However, chronic treatment with PEE at doses of 1000 and 1500 mg/kg had a significant effect on immobility time in comparison with stressed mice in both FST and TST (P < 0.01, P < 0.05, respectively).
The results of one-way ANOVA on body weight analysis revealed no significant difference between initial weights among experimental groups. Body weight markedly increased after the 2-week procedure in control mice, as well as in the fluoxetine treatment group. However, the CRS-exposed mice showed no significant increase in body weight. Moreover, 2-week treatment with different doses of PEE increased body weight, although not significantly (Figure 2G).
4.4. Effects of PEE on Serum Levels of Corticosterone and Tissue Levels of Inflammatory Cytokines
As shown in Figure 3A, chronic stress significantly increased serum levels of corticosterone in comparison with non-stressed mice (P < 0.01), while different doses of PEE (500, 1000, and 1500 mg/kg) significantly reduced corticosterone levels compared with CRS mice (P < 0.05, P < 0.05, P < 0.01, respectively).
A, effects of PEE on serum levels of corticosterone; and B, tissue levels of TNF-α; and C, IL-1β in PFC and HIP regions among experimental groups. Data are presented as mean ± S.E.M; * P < 0.05, ** P < 0.01, **** P < 0.0001 vs Ctrl group; # P < 0.05, ## P < 0.01, ### P < 0.001, #### P < 0.0001 vs CRS group. (PEE, hydroethanolic extract of common polypody rhizome; IL-1β, interlukine-1 beta; TNF-α, tumor necrosis factor-alpha; PFC, prefrontal cortex; HIP, hippocampus; CRS, chronic restraint stress; Ctrl, control; FLX, fluoxetine)
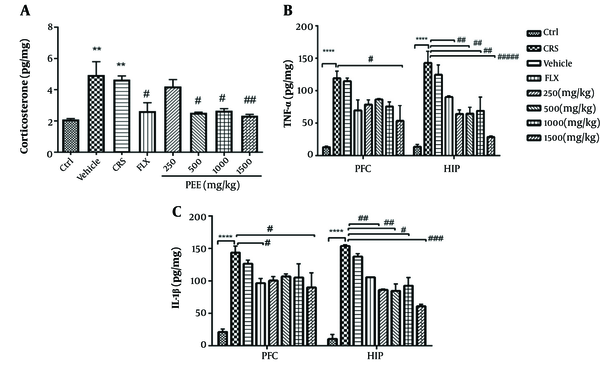
There was a statistically significant difference in tissue levels of TNF-α and IL-1β among experimental groups (Figures 3B, C). Post-hoc analysis indicated that chronic stress exposure significantly increased levels of both pro-inflammatory cytokines in the PFC and HIP (P < 0.0001). Levels of TNF-α and IL-1β significantly declined with all doses of PEE in the HIP and with 1500 mg.kg-1 PEE in the PFC. Moreover, levels of IL-1β in the PFC significantly decreased with fluoxetine therapy.
4.5. Effects of PEE on Oxidative Stress Markers
There was a significant difference in tissue levels of MDA among study groups (Figure 4A). Chronic exposure to stress significantly increased MDA levels compared to unstressed mice (P < 0.0001). However, two weeks of treatment with 1000 and 1500 mg/kg PEE had a significant effect on MDA in the PFC and HIP. Likewise, there was a significant decrease of MDA in the HIP with fluoxetine treatment. Tissue levels of TAC significantly decreased during CRS and significantly increased in the HIP following chronic treatment with 1500 mg/kg PEE (P < 0.05) (Figure 4B).
A, anti-oxidative effects of PEE on levels of MDA; B, TAC; and C, enzymatic activity of SOD; and D, GPx in PFC and HIP areas in chronic stress-exposed mice. Data are presented as mean ± S.E.M; * P < 0.05, ** P < 0.01, **** P < 0.0001 vs Ctrl group; # P < 0.05, ## P < 0.01, ### P < 0.001, #### P < 0.0001 vs CRS group. (PEE, hydroethanolic extract of common polypody rhizome; MDA, malondialdehyde; TAC, total antioxidant capacity; SOD, superoxide dismutase; GPx, glutathione peroxidase; PFC, prefrontal cortex; HIP, hippocampus; CRS, chronic restraint stress; Ctrl, control; FLX, fluoxetine)
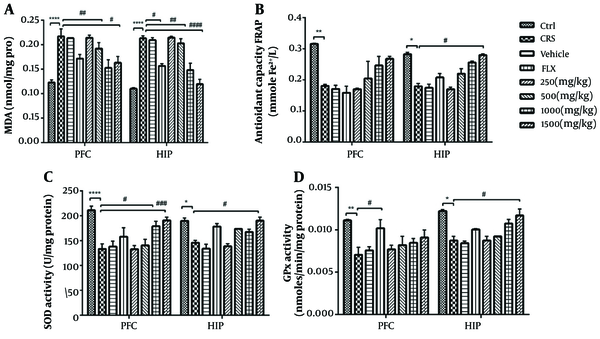
Analysis of enzymatic activity against lipid peroxidation showed that SOD and GPx activity in both tissues significantly decreased in stressed mice compared to the control group (Figures 4C, D). Polypody rhizome significantly promoted the activity of SOD in the PFC when administered at 1000 and 1500 mg.kg-1, and in the HIP at the highest administered dose (1500 mg.kg-1). Moreover, the activity of GPx significantly increased with 1500 mg.kg-1 PEE in the HIP, and the same result was observed in the PFC with fluoxetine treatment (P < 0.05).
4.6. Effects of PEE on Brain-Derived Neurotrophic Factor Expression and Apoptotic Factors
Chronic stress significantly decreased BDNF levels in both the PFC and HIP (P < 0.001). This factor was significantly enhanced in the HIP by 1500 mg.kg-1 PEE therapy (P < 0.05) or fluoxetine treatment (P < 0.01) (Figure 5A). As shown in Figures 5B, C, levels of pro-apoptotic factors Bax in the PFC and cleaved-caspase-3 in both tissues significantly increased in stressed mice compared with the control group (P < 0.01, P < 0.0001, respectively). Treatment with different doses of PEE (500, 1000, and 1500 mg.kg-1) or fluoxetine revealed a significant decrease in the PFC levels of Bax and cleaved-caspase-3. Additionally, levels of Bax in the HIP were significantly decreased by PEE at doses of 1000 and 1500 mg.kg-1 (P < 0.05). Also, the administration of all doses of PEE, as well as treatment with fluoxetine, significantly reduced levels of cleaved-caspase-3 in the HIP. Moreover, there was a significant difference in the tissue levels of Bcl-2 in stressed mice compared with the control group (P < 0.05). Polypody rhizome treatment at 1000 and 1500 mg.kg-1 doses significantly promoted this marker in the PFC (P < 0.05) (Figure 5D). The results also showed increased levels of Bcl-2 in the HIP with the administration of PEE at doses of 1000 and 1500 mg.kg-1, although this change was not statistically significant.
A, effects of PEE on protein expression of BDNF; and B, apoptotic factors including Bax; C, cleaved-caspase-3; and D, Bcl-2 among experimental groups; E, representative images of the protein band expressions measured by western blot. Data are presented as mean ± S.E.M; * P < 0.05, ** P < 0.01, *** P < 0.001, **** P < 0.0001 vs Ctrl group; # P < 0.05, ## P < 0.01, ### P < 0.001, #### P < 0.0001 vs CRS group. (PEE, hydroethanolic extract of common polypody rhizome; BDNF, brain-derived neurotrophic factor; PFC, prefrontal cortex; HIP, hippocampus; CRS, chronic restraint stress; Ctrl, control; FLX, fluoxetine)
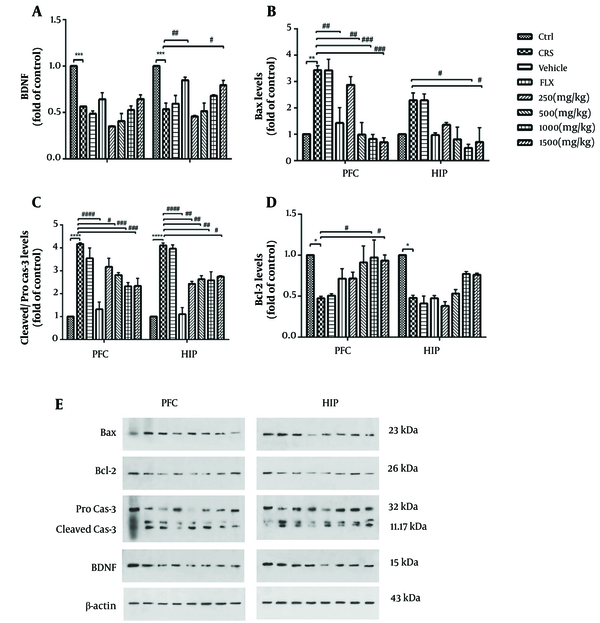
5. Discussion
The findings of the current study indicated that CRS induced anxiety and depressive-like behaviors, characterized by increased immobility time in FST and TST, and reduced time spent in the central zone of OFT and %OAE and %OAT in EPM, respectively. These changes were reversed by oral administration of PEE, particularly associated with higher doses (1000 and 1500 mg.kg-1). Behavioral effects were accompanied by diminished plasma levels of corticosterone and tissue levels of inflammatory cytokines and MDA, as well as increased enzymatic activity of SOD in the PFC and HIP. Moreover, PEE restored TAC levels, GPx activity, and BDNF expression in the HIP. It also promoted the anti-apoptotic factor Bcl-2 in the PFC and downregulated the expression of pro-apoptotic factors, Bax and caspase-3, in both regions. These findings align with the traditional use of common polypody for the treatment of anxiety and depression in PM (18).
Numerous studies have demonstrated that stress plays a pivotal role in psychiatric disorders, including depression and anxiety (1). The study by Banagozar Mohammadi et al. (7) showed that the number of central entries in OFT and %OAE in EPM were decreased in CRS-exposed mice. Likewise, CRS (2h/day, 3 weeks) enhanced the immobility time in FST and TST, which were used to assess depressive-like behavior. This study and other previous reports illustrated that these behavioral changes could be reversed by antidepressant treatments in stress-induced animal models (2, 6). In our study, behavioral alterations after two weeks of oral PEE administration identified the possible anxiolytic and antidepressant effects of the common PEE . Although the underlying mechanisms have not been fully elucidated to date, several studies have confirmed the involvement of inflammatory pathways in these chronic stress-mediated disorders. Accordingly, pro-inflammatory cytokines are elevated, while antagonist mediators are downregulated, leading to neuroinflammatory and neurodegenerative sequels in related areas, including the PFC and HIP, which are fundamental brain structures for emotional, behavioral, and cognitive functions (1, 4). Moreover, stress-induced over-activation of the HPA axis, a pivotal regulator against stressful circumstances, leads to glucocorticoid (corticosterone in rodents) release, which consequently influences limbic structures and the HIP, bringing about mood disorders such as anxiety and depression (5, 9).
The immune system and neuroinflammation play an important role in psychiatric illnesses and treatment strategies. In this regard, investigations on herbal products and phytoconstituents have interested researchers due to their safety and fewer side effects compared with classical antidepressant and anxiolytic medications, including selective serotonin reuptake inhibitors (SSRIs) (10, 11, 29). Among multiple herbal metabolites, flavonoids—a subgroup of herbal polyphenols—are the most widely studied group. Their anti-inflammatory, anti-oxidative, and antidepressant effects, along with other bioactivities, have been illustrated in numerous investigations (11, 30). Flavan-3-ols, also known as catechins, were identified in the common PEE and fronds in previous studies. Catechins are one of the main subclasses of flavonoids and have potential anxiolytic and antidepressant effects via the regulation of different related neuropathways, including the HPA axis, oxidative markers, and some neuroinflammatory cascades (30). Decreased levels of corticosterone and inflammatory cytokines with chronic administration of PEE demonstrate the potential anti-stress and anti-inflammatory effects of this herbal extract and suggest possible anxiolytic and antidepressant effects for common polypody via immunomodulatory properties.
According to a large body of evidence, maladaptive responses of the immune system trigger oxidative pathways, decrease BDNF, and consequently contribute to apoptotic alterations. The development of neuropsychiatric disorders and, more importantly, their therapeutic management involves the collaboration of these pathways (1, 4, 8). Oxidative stress occurs as a result of either excessive generation of reactive oxygen species (ROS) or impaired antioxidant capacity. The antioxidant defense system consists of enzymatic scavengers, including SOD and GPx, and non-enzymatic effectors, which are downregulated during depression and anxiety. Additionally, levels of MDA, as a marker of cell membrane lipid peroxidation, are higher in the mentioned disorders (9, 33). Herbal constituents with high antioxidant properties for the prevention and treatment of oxidative-mediated pathologies have always been considered (29). Polypodium leucotomos is another Polypodiaceae fern that is widely studied in dermatologic disorders. The antioxidant, anti-inflammatory, antimutagenic, and immunomodulatory properties of this plant were reported in various studies (35). In this context, the antioxidative effects of various parts of common polypody have been investigated in previous experiments (20, 22, 23). The study by Batur et al. (20) showed that common polypody has wound healing efficacy along with antioxidant and antimicrobial activities in both in vivo and in vitro methods. Based on current results, it could be suggested that common polypody exerts anxiolytic and antidepressant activity by modifying oxidative pathways.
Brain-derived neurotrophic factor is regarded as one of the most extensively studied members within the nerve growth factor family, contributing to the development, function, and survival of neuronal cells, and it is downregulated during chronic stress exposure (2, 4). Although physiological apoptosis is crucial for normal cellular homeostasis, it can be dysregulated by various stimuli, like environmental stress, leading to pathological apoptosis. A main regulator in the pathophysiology of cell death, the Bcl-2 family, consists of anti-apoptotic proteins such as Bcl-2 and pro-apoptotic factors including Bax protein, both of which are dysregulated in stress-exposed animals. Also, cleaved-caspase-3, the most important executioner caspase, is over-expressed as a result of chronic stress (2, 4, 32). Wang et al. (36) demonstrated that chronic immobilization (6h/day, 3 weeks) decreases BDNF levels and the phosphorylation of extracellular signal-regulated kinase (pERK) in the PFC and HIP. It also alters mRNA expression of apoptotic factors including Bax and Bcl-2 in male Wistar rats. Three weeks of treatment with resveratrol, a natural polyphenol rich in Polygonum cuspidatum, normalized these levels along with behavioral changes and exerted antidepressant-like effects in CRS rats. Consistent with this study and other previous reports (6), the results of our study suggest that common polypody exerts anxiolytic and antidepressant effects via the regulation of apoptotic factors and neurotrophic expression. An in vitro study by Farràs et al. (22) on the potential cytotoxicity, ROS production, and antioxidative properties of methanolic extract of common polypody fronds showed that at physiological levels against different cell lines, it was not cytotoxic and released cellular repair activity in 3T3 fibroblast cells. The results also showed a significant amount of shikimic acid, along with caffeoylquinic acid derivatives and flavonoids such as epicatechin and catechin, in the fronds of common polypody.
Since 2008, the rhizome of common polypody has been recognized by the European Medicines Agency (EMA) as an herbal medicine with expectorant properties for the treatment of cough and cold. It is also approved for use in cases of occasional constipation (12). In the field of neuropsychologic disorders, there are limited studies on the efficacy of the common PEE , its mechanisms of action, and related herbal components responsible for its functions (12, 25). The study by Dar et al. (24) on haloperidol-induced catalepsy in Swiss mice indicated that common PEE had anticataleptic effects through facilitating dopaminergic transmission, possibly acting as a dopamine D2 receptor agonist. As mentioned in the previous quantitative analysis, the main isolated phytochemical components from the rhizome of common polypody are flavonoids such as flavan-3-ol derivatives, triterpenoids, phytoecdysteroids, and saponin glycosides, among others (12, 14, 26, 27). Based on our findings, the effect of common PEE on inflammatory, oxidative, and apoptotic markers may be associated with its phenolic and considerable flavonoid contents, as determined in the present study. Further analysis is needed to identify the main active ingredient(s) to explain the various effects of common polypody on these changes.
5.1. Conclusions
The results of the present study revealed the neuromodulatory effects of common polypody against anxiety and depressive-like behaviors in a CRS-animal model by modifying serum levels of corticosterone as well as the expression of inflammatory cytokines, oxidative, and apoptotic factors in the PFC and HIP. Our findings suggest that common polypody may be considered a potential mood stabilizer in stress-mediated psychiatric illnesses.
Acknowledgements
References
-
1.
Duman RS. Neuronal damage and protection in the pathophysiology and treatment of psychiatric illness: stress and depression. Dialogues Clin Neurosci. 2009;11(3):239-55. [PubMed ID: 19877493]. [PubMed Central ID: PMC3181922]. https://doi.org/10.31887/DCNS.2009.11.3/rsduman.
-
2.
Ning J, Jing-Wei L, Hai-Xia W, Hong H, Qiong W, Shan-Guang C, et al. Antidepressant-like effects of ginsenoside Rg1 in the chronic restraint stress-induced rat model. Digital Chinese Medicine. 2019;2(4):207-18.
-
3.
Mathers CD, Loncar D. Projections of global mortality and burden of disease from 2002 to 2030. PLoS Med. 2006;3(11). e442. [PubMed ID: 17132052]. [PubMed Central ID: PMC1664601]. https://doi.org/10.1371/journal.pmed.0030442.
-
4.
Kubera M, Obuchowicz E, Goehler L, Brzeszcz J, Maes M. In animal models, psychosocial stress-induced (neuro)inflammation, apoptosis and reduced neurogenesis are associated to the onset of depression. Prog Neuropsychopharmacol Biol Psychiatry. 2011;35(3):744-59. [PubMed ID: 20828592]. https://doi.org/10.1016/j.pnpbp.2010.08.026.
-
5.
Felger JC, Lotrich FE. Inflammatory cytokines in depression: neurobiological mechanisms and therapeutic implications. Neuroscience. 2013;246:199-229. [PubMed ID: 23644052]. [PubMed Central ID: PMC3741070]. https://doi.org/10.1016/j.neuroscience.2013.04.060.
-
6.
Geng X, Wu H, Li Z, Li C, Chen D, Zong J, et al. Jie-Yu-He-Huan Capsule Ameliorates Anxiety-Like Behaviours in Rats Exposed to Chronic Restraint Stress via the cAMP/PKA/CREB/BDNF Signalling Pathway. Oxid Med Cell Longev. 2021;2021:1703981. [PubMed ID: 34646421]. [PubMed Central ID: PMC8505060]. https://doi.org/10.1155/2021/1703981.
-
7.
Banagozar Mohammadi A, Torbati M, Farajdokht F, Sadigh-Eteghad S, Fazljou SMB, Vatandoust SM, et al. Sericin alleviates restraint stress induced depressive- and anxiety-like behaviors via modulation of oxidative stress, neuroinflammation and apoptosis in the prefrontal cortex and hippocampus. Brain Res. 2019;1715:47-56. [PubMed ID: 30898675]. https://doi.org/10.1016/j.brainres.2019.03.020.
-
8.
de Munter J, Pavlov D, Gorlova A, Sicker M, Proshin A, Kalueff AV, et al. Increased Oxidative Stress in the Prefrontal Cortex as a Shared Feature of Depressive- and PTSD-Like Syndromes: Effects of a Standardized Herbal Antioxidant. Front Nutr. 2021;8:661455. [PubMed ID: 33937310]. [PubMed Central ID: PMC8086427]. https://doi.org/10.3389/fnut.2021.661455.
-
9.
Fekri K, Nayebi AM, Sadigh-Eteghad S, Farajdokht F, Mahmoudi J. The neurochemical changes involved in immobilization stress-induced anxiety and depression: Roles for oxidative stress and neuroinflammation. Neurochemical Journal. 2020;14:133-49.
-
10.
Cartwright C, Gibson K, Read J, Cowan O, Dehar T. Long-term antidepressant use: patient perspectives of benefits and adverse effects. Patient Prefer Adherence. 2016;10:1401-7. [PubMed ID: 27528803]. [PubMed Central ID: PMC4970636]. https://doi.org/10.2147/PPA.S110632.
-
11.
Khan H, Perviz S, Sureda A, Nabavi SM, Tejada S. Current standing of plant derived flavonoids as an antidepressant. Food Chem Toxicol. 2018;119:176-88. [PubMed ID: 29704578]. https://doi.org/10.1016/j.fct.2018.04.052.
-
12.
Europian Medicine Agency. Assessment report on Polypodium Vulgare L.,rhizoma. London: Europian Medicine Agency; 2017, [cited 2023]. Available from: https://www.ema.europa.eu/en/documents/herbal-report/addendum-assessment-report-polypodium-vulgare-l-rhizoma_en.pdf.
-
13.
Khoshravesh R, Akhani H, Eskandari M, Greuter W. Ferns and fern allies of Iran. Rostaniha. 2009;10(Suppl 1):1-130.
-
14.
Simon A, Vanyolos A, Beni Z, Dekany M, Toth G, Bathori M. Ecdysteroids from Polypodium vulgare L. Steroids. 2011;76(13):1419-24. [PubMed ID: 21803067]. https://doi.org/10.1016/j.steroids.2011.07.007.
-
15.
Hashempur MH, Khademi F, Rahmanifard M, Zarshenas MM. An Evidence-Based Study on Medicinal Plants for Hemorrhoids in Medieval Persia. J Evid Based Complementary Altern Med. 2017;22(4):969-81. [PubMed ID: 29228790]. [PubMed Central ID: PMC5871264]. https://doi.org/10.1177/2156587216688597.
-
16.
Jalali A, Raesi Vanani A, Shirani M. Ethnobotanical Approaches of Traditional Medicinal Plants Used in the Management of Asthma in Iran. Jundishapur J Nat Pharm Prod. 2019;15(1). e62269. https://doi.org/10.5812/jjnpp.62269.
-
17.
Mahdizadeh S, Khaleghi Ghadiri M, Gorji A. Avicenna's Canon of Medicine: a review of analgesics and anti-inflammatory substances. Avicenna J Phytomed. 2015;5(3):182-202. [PubMed ID: 26101752]. [PubMed Central ID: PMC4469963].
-
18.
Khodaei MA, Noorbala AA, Parsian Z, Targhi ST, Emadi F, Alijaniha F, et al. Avicenna (980-1032CE): The Pioneer in Treatment of Depression 1. Transylvanian Review. 2017;25(17):4377-89.
-
19.
Bathori M, Pongracz Z. Phytoecdysteroids--from isolation to their effects on humans. Curr Med Chem. 2005;12(2):153-72. [PubMed ID: 15638733]. https://doi.org/10.2174/0929867053363450.
-
20.
Batur S, Ayla S, Sakul AA, Okur ME, Karadag AE, Daylan B, et al. An Alternative Approach Wound Healing Field with Polypodium Vulgare. Medeni Med J. 2020;35(4):315-23. [PubMed ID: 33717624]. [PubMed Central ID: PMC7945725]. https://doi.org/10.5222/MMJ.2020.89983.
-
21.
Tichaczek-Goska D, Glensk M, Wojnicz D. The Enhancement of the Photodynamic Therapy and Ciprofloxacin Activity against Uropathogenic Escherichia coli Strains by Polypodium vulgare Rhizome Aqueous Extract. Pathogens. 2021;10(12). [PubMed ID: 34959499]. [PubMed Central ID: PMC8704307]. https://doi.org/10.3390/pathogens10121544.
-
22.
Farras A, Mitjans M, Maggi F, Caprioli G, Vinardell MP, Lopez V. Polypodium vulgare L. (Polypodiaceae) as a Source of Bioactive Compounds: Polyphenolic Profile, Cytotoxicity and Cytoprotective Properties in Different Cell Lines. Front Pharmacol. 2021;12:727528. [PubMed ID: 34603041]. [PubMed Central ID: PMC8482143]. https://doi.org/10.3389/fphar.2021.727528.
-
23.
Farràs A, Cásedas G, Les F, Terrado EM, Mitjans M, López V. Evaluation of anti-tyrosinase and antioxidant properties of four fern species for potential cosmetic applications. Forests. 2019;10(2):179.
-
24.
Dar PA, Sofi G, Parray SA, Jafri MA. Effect of rhizome extract of Bisfayej (Polypodium vulgare L) on chemically induced catalepsy in mice. International Journal of Institutional Pharmacy and Life Sciences. 2012;2(4):94-108.
-
25.
Saeedi M, Babaie K, Karimpour-Razkenari E, Vazirian M, Akbarzadeh T, Khanavi M, et al. In vitro cholinesterase inhibitory activity of some plants used in Iranian traditional medicine. Nat Prod Res. 2017;31(22):2690-4. [PubMed ID: 28278615]. https://doi.org/10.1080/14786419.2017.1290620.
-
26.
Glensk M, Dudek MK, Ciach M, Wlodarczyk M. Isolation and structural determination of flavan-3-ol derivatives from the Polypodium vulgare L. rhizomes water extract. Nat Prod Res. 2021;35(9):1474-83. [PubMed ID: 31538499]. https://doi.org/10.1080/14786419.2019.1655302.
-
27.
Swamy MK, Akhtar MS. Natural Bio-Active Compounds: Volume 2: Chemistry, Pharmacology and Health Care Practices. Springer; 2019.
-
28.
Das N, Mishra SK, Bishayee A, Ali ES, Bishayee A. The phytochemical, biological, and medicinal attributes of phytoecdysteroids: An updated review. Acta Pharm Sin B. 2021;11(7):1740-66. [PubMed ID: 34386319]. [PubMed Central ID: PMC8343124]. https://doi.org/10.1016/j.apsb.2020.10.012.
-
29.
Özdemir Z, Bildziukevich U, Wimmerová M, Macůrková A, Lovecká P, Wimmer Z. Plant adaptogens: natural medicaments for 21st century? ChemistrySelect. 2018;3(7):2196-214.
-
30.
Pannu A, Sharma PC, Thakur VK, Goyal RK. Emerging Role of Flavonoids as the Treatment of Depression. Biomolecules. 2021;11(12). [PubMed ID: 34944471]. [PubMed Central ID: PMC8698856]. https://doi.org/10.3390/biom11121825.
-
31.
A. Alasmary F, Assirey EA, El-Meligy RM, Awaad AS, El-Sawaf LA, Allah MM, et al. Analysis of Alpina officinarum Hance, chemically and biologically. Saudi Pharm J. 2019;27(8):1107-12. [PubMed ID: 31885470]. [PubMed Central ID: PMC6921168]. https://doi.org/10.1016/j.jsps.2019.09.007.
-
32.
Ghazizadeh J, Hamedeyazdan S, Torbati M, Farajdokht F, Fakhari A, Mahmoudi J, et al. Melissa officinalis L. hydro-alcoholic extract inhibits anxiety and depression through prevention of central oxidative stress and apoptosis. Exp Physiol. 2020;105(4):707-20. [PubMed ID: 32003913]. https://doi.org/10.1113/EP088254.
-
33.
Nabaee E, Kesmati M, Shahriari A, Khajehpour L, Torabi M. Cognitive and hippocampus biochemical changes following sleep deprivation in the adult male rat. Biomed Pharmacother. 2018;104:69-76. [PubMed ID: 29772442]. https://doi.org/10.1016/j.biopha.2018.04.197.
-
34.
Samarghandian S, Azimi-Nezhad M, Farkhondeh T, Samini F. Anti-oxidative effects of curcumin on immobilization-induced oxidative stress in rat brain, liver and kidney. Biomed Pharmacother. 2017;87:223-9. [PubMed ID: 28061405]. https://doi.org/10.1016/j.biopha.2016.12.105.
-
35.
Rodriguez-Luna A, Zamarron A, Juarranz A, Gonzalez S. Clinical Applications of Polypodium leucotomos (Fernblock((R))): An Update. Life (Basel). 2023;13(7). [PubMed ID: 37511888]. [PubMed Central ID: PMC10381169]. https://doi.org/10.3390/life13071513.
-
36.
Wang X, Xie Y, Zhang T, Bo S, Bai X, Liu H, et al. Resveratrol reverses chronic restraint stress-induced depression-like behaviour: Involvement of BDNF level, ERK phosphorylation and expression of Bcl-2 and Bax in rats. Brain Res Bull. 2016;125:134-43. [PubMed ID: 27346276]. https://doi.org/10.1016/j.brainresbull.2016.06.014.