Abstract
Background:
This study synthesized alginate-chitosan nanoparticles (ALG/CS NPs) as nanocarriers for the hydrophobic drug levothyroxine and investigated their nanoparticle size.Objectives:
Alginate-chitosan nanoparticles were prepared through ionotropic gelation of the chitosan core followed by complexation with alginate.Methods:
The nanoparticles were characterized for particle morphology, size, and polydispersity index using transmission electron microscopy (TEM) and photon correlation spectroscopy.Results:
Alginate-chitosan nanoparticles were found in a nanoscale spherical shape with an average particle size of 90 - 110 nm. Results indicated that the release mechanism depends on dissolution in the initial hours. For levothyroxine release from ALG/CS NPs, the Higuchi model fits better than the other models. The drug release kinetics were investigated, and the release kinetics data were best fitted with the Higuchi model.Conclusions:
Levothyroxine was released mainly in the first 20 hours at an average rate of up to 78% at pH 7.4.Keywords
Levothyroxine Nanocarriers Chitosan Alginate Nanoparticle Drug Delivery
1. Background
The thyroid gland creates and produces hormones that play a major role in many different body systems, such as protein, fat, and carbohydrate metabolism (1-3). When the thyroid makes either too much or too little of these critical hormones, it's called a thyroid disorder. There are a few distinct types of thyroid disease, including hyperthyroidism, hypothyroidism, thyroiditis, and Hashimoto's thyroiditis, which affect around 1% of the general population (4). Thyroid disease can affect anyone—men, women, infants, children, and the elderly (5). It can be present at birth or develop as you age due to functional insufficiency, primary atrophy, partial or complete absence of the thyroid gland, or the effects of surgery, radiation, or anti-thyroid agents (6, 7). Adequate intake of thyroid hormone is essential for normal mental and physical functioning, especially for children's normal mental and physical development. Levothyroxine is used to treat hypothyroidism, replacing or providing more of the thyroid hormone normally produced by the thyroid gland (8). The dose is based on your age, weight, health, and response to treatment. Many foods and other substances can interfere with the absorption of levothyroxine (9, 10). Substances that reduce absorption include aluminum and magnesium-containing antacids, simethicone, sucralfate, cholestyramine, colestipol, and polystyrene sulfonate (11). Levothyroxine contains an alanine side chain, which causes drug absorption to be impacted by gastrointestinal pathology. Therefore, to minimize interactions, levothyroxine should be taken 30 minutes to one hour before eating or drinking anything (12, 13). Recently, researchers have reported the digestion of subcutaneous lipid, though they suggested it is unlikely to be sufficient for drug delivery (14, 15). The oral route is the most widespread route for the administration of many drugs with lipophilic, hydrophobic, and zwitterionic structures (16, 17). It allows for the administration of liquid, semisolid, and solid formulations. Over the past several decades, nanocarriers have been successfully developed to deliver a variety of chemotherapy drugs, inhibitors, nucleic acids, proteins, as well as contrast agents that can deliver drugs to the right place, at appropriate times, and at the right dosage (18, 19). Nanocarriers are composed of engineered biodegradable polymers, natural biopolymers, lipids, and polysaccharides, offering potential control to improve drug stability and increase the duration of the therapeutic effect (20). Among nanocarriers, alginate and chitosan are exceptionally promising and have been widely applied for controlling drug release (21-23). Researchers have utilized alginate-chitosan to prepare controlled drug delivery systems from liquids, solid particulates, and hydrogel-forming systems. Nalini et al. used alginate/chitosan nanoparticles as carriers of the hydrophobic drug quercetin (24). Alnaief et al. prepared hybrid chitosan-alginate aerogel microparticles without using an additional ionic crosslinker as a potential pulmonary drug delivery system (25). Li et al. prepared ALG/CS NPs by ionotropic pre-gelation of an alginate core followed by chitosan polyelectrolyte complexation for nifedipine drug delivery (26). Alginate and chitosan are polysaccharides derived from natural sources (27-29). These compounds have applications in the food, medical, and pharmaceutical fields due to their biocompatibility, nontoxicity, gel-forming properties, and biodegradability, in addition to their effect on membrane permeability (30, 31). Chitosan is composed of D-glucosamine and N-acetyl-D-glucosamine. Chitosan can impart a positive surface charge to nanoparticles, prolonging the contact time of active ingredients with the epithelium and enhancing absorption through the paracellular transport pathway via tight junctions (32). Alginate is composed of 1 - 4 linked α-L-guluronic and β-D-mannuronic acid residues. Alginate nanoparticles can be easily obtained by inducing gelation with calcium ions. Chitosan, a cationic natural polymer, has been combined with alginate to prepare nanoparticles because it is biocompatible, biodegradable, and nontoxic, making it suitable for peroral drug delivery (33-35). Alginate and chitosan were dispersed in a liquid system that undergoes in situ gelation after oral administration, with the resulting gel controlling the drug release rate in the gastric and intestinal environments. Alginate-chitosan combinations can be formulated into particulate systems of varying sizes for different routes of drug administration (36-39).
In this context, the preparation of ALG/CS NPs as nanocarriers for levothyroxine is presented. A novel carrier for levothyroxine was created using chitosan and alginate biopolymers. Several researchers have investigated the carrier properties of ALG/CS NPs for a variety of drugs. Although levothyroxine has been studied in different nanoparticles from various perspectives, there is no detailed report on the specific use of ALG/CS NPs as a carrier for levothyroxine. Proof of concept is demonstrated by assessing the levothyroxine release from ALG/CS NPs at pH 7.4. Additionally, the kinetics of levothyroxine release were explored using first-order, Higuchi, zero-order, Korsmeyer-Peppas, and Hixon-Crowell models.
2. Methods
2.1. Materials
Levothyroxine sodium salt was kindly provided by Iran Hormone Co. Chitosan (medium molecular weight) and sodium alginate were obtained from Sigma. Ethanol (HPLC grade), methanol (HPLC grade), acetic acid (HPLC grade), and hydrochloric acid (HPLC grade) were purchased from Merck. Calcium chloride, potassium bromide, and sodium hydroxide were obtained from Sigma Aldrich. Uranyl acetate was obtained from Laad Research Industries Company. Double-distilled water was prepared in our laboratory.
2.2. Preparation of Blank Alginate-Chitosan Nanoparticles
A 10 mmol sodium alginate and 2 mmol calcium chloride solution were dissolved in deionized water, and then the pH was adjusted to 5 using hydrochloric acid (0.1 N). Chitosan was dissolved in a 1% acetic acid solution, and its pH was adjusted to 5.4 using NaOH (0.1 N). Rajaonarivony's method with slight modifications was used for preparing ALG/CS NPs (38). Calcium chloride solution (3.35 mg/mL) was added dropwise to 10 mL of sodium alginate (3.0 mg/mL). The solution was stirred at 500 rpm for 30 minutes, and after complete dissolution, 4 ml of chitosan solution (0.8 mg/mL) was added to the resulting calcium alginate pre-gel and mixed for one hour. The suspension was equilibrated overnight to allow uniform nanoparticle formation (26). The size and structure of the ALG/CS NPs were examined using a transmission electron microscope (Zeiss-EM 10C-Germany) at an accelerating voltage of 80 kV for point-to-point transmission. Before analysis, the samples were diluted 1: 2, applied on a carbon-coated grid, stained with uranyl acetate for 30 seconds, and placed on copper grids with films for observation (1).
2.3. Preparation of Alginate-Chitosan Nanoparticles Loaded with Levothyroxine
Alginate-chitosan nanoparticles were prepared by adding a sodium alginate solution containing calcium chloride to a chitosan solution while stirring constantly at 500 rpm at room temperature. Fifty milligrams of levothyroxine were dissolved in an ethanol/water mixture (1: 1), and the levothyroxine solution was then added to the above mixture while stirring for 30 minutes (26). The preparation steps are presented in Figure 1.
The preparation steps of alginate-chitosan nanoparticles (ALG/CS NPs)
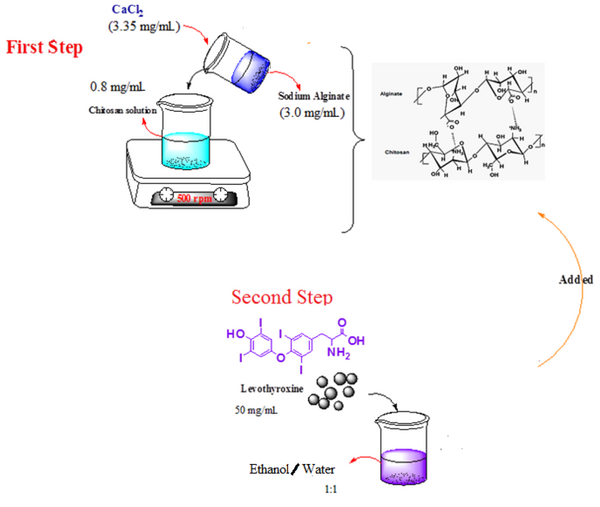
2.4. Characterization of Alginate-Chitosan Nanoparticles
The concentration of levothyroxine in solutions was measured using high-performance liquid chromatography (HPLC, Agilent Technology 1260 Infinity series) with a C18 column (250 × 4.6 mm, 5 µm). A 1% acetic acid solution-methanol mixture (50: 50 v/v) was used as the mobile phase at a flow rate of 0.8 mL/min, with detection at a wavelength of 225 nm (40). Solutions were analyzed in duplicate. Fourier transform infrared (FT-IR) spectroscopy was recorded using a Perkin Elmer-Spectrum 65 device at 4000 - 400 cm⁻¹ from KBr pellets for the detection of functional groups. Transmission electron microscopy (TEM) was used to analyze the morphology of the nanoparticles. The polydispersity index, diameter of nanoparticles, and zeta potential (ZP) of the nanoparticles were determined using photon correlation spectroscopy (Nano ZS4700, Malvern Instruments, Worcestershire, UK).
2.5. In Vitro Release Behavior of Levothyroxine
This experiment was conducted using a static horizontal Franz diffusion cell to evaluate the amount of levothyroxine released from ALG/CS NPs. A cellulose acetate membrane with a molecular weight cutoff of 12,000 Da and a surface area of 2.0 cm² was used and mounted on the Franz diffusion cell. The receptor medium was precisely 50 mL in volume, composed of an aqueous solution of physiological saline, phosphate buffer solution (0.01 M), and 1% ethanol, stirred by a magnetic bar at 750 rpm to homogenize the medium. Two-milliliter aliquots were loaded onto the membrane in the donor compartment. The temperature of the assay was controlled at precisely 37°C. At predetermined time intervals, 1 mL aliquots of the release medium were withdrawn using a syringe needle, and the same volumes of freshly prepared receptor medium were added. The samples were analyzed using the HPLC instrument as previously described (8).
2.6. Determination of Encapsulation Efficiency
Levothyroxine's encapsulation efficiency (EE) and loading capacity (LC) were measured within the ALG/CS NPs. The solution containing levothyroxine-loaded ALG/CS NPs was centrifuged at 45,000 rpm for 30 minutes, and the supernatant was quantified spectrophotometrically at 225 nm. The percent encapsulation efficiency and loading capacity were calculated as follows (39):
3. Results
3.1. Alginate/Chitosan Interactions
Chitosan, a positively charged, linear nitrogen-containing polysaccharide made up of glucosamine and N-acetyl-glucosamine units connected by (1→4) β-glycosidic linkages, ranks as the second most prevalent polymer found in nature, surpassed only by cellulose (34). This hydrophilic polymer is derived from the deacetylation of chitin's aminoacetyl groups, with chitin being a primary constituent of crustacean shells, fungal cell walls, and insect cuticles. Chitosan not only shares the common attributes of polysaccharides, such as biocompatibility and biodegradability (35), but also possesses bioadhesive qualities. These bioadhesive properties allow for ionic bonds between the positively charged amino groups in chitosan and the negatively charged mucosal layers (35), making it an effective carrier in drug delivery systems within the pharmaceutical sector.
Alginate, on the other hand, is a hydrophilic anionic copolymer consisting of alternating sequences of (1→4) linked β-D-mannuronic acid (M units) and α-L-guluronic acid (G units). It is sourced from marine brown algae and certain bacteria, where it is found within cell walls and intercellular spaces (35, 36). Alginate's utility in pharmaceutical applications is largely due to its propensity to form hydrogels upon binding with divalent cations (37).
The gelation properties of both chitosan and alginate are exploited to create polyelectrolyte complexes that combine polycations with polyanions. The polyionic complex formed between alginate and chitosan arises from ionic interactions between the amine groups of chitosan and the carboxylic groups of alginate through a process known as ionic gelation (37-39). These interactions result in a reduction of porosity within the complex, which in turn enhances the protection of the encapsulated substances and moderates their release more effectively than when using chitosan or alginate alone. Control of the atoms and the capacity to tailor the physicochemical properties requires a comprehensive understanding of the structure, energetic properties, and the control of the degree of association between the functional groups. By adding calcium chloride dropwise to the alginate solution, the calcium ions act as a crosslinker, creating a crosslink between the alginate monomers (through the carboxylic acid units in the G residues, where a divalent cation reacts with 4 COOH groups) that forms the pre-gel. If the residence time of the solution is increased, the bond between the calcium ions and the alginate will strengthen (26). By adding a solution containing chitosan, ionic and hydrogen bonds are formed between the functional groups of chitosan and alginate, resulting in a gel. Due to the gel composition, levothyroxine added to the gel is encapsulated (Figure 2).
The interaction of alginate-calcium and chitosan and levothyroxine
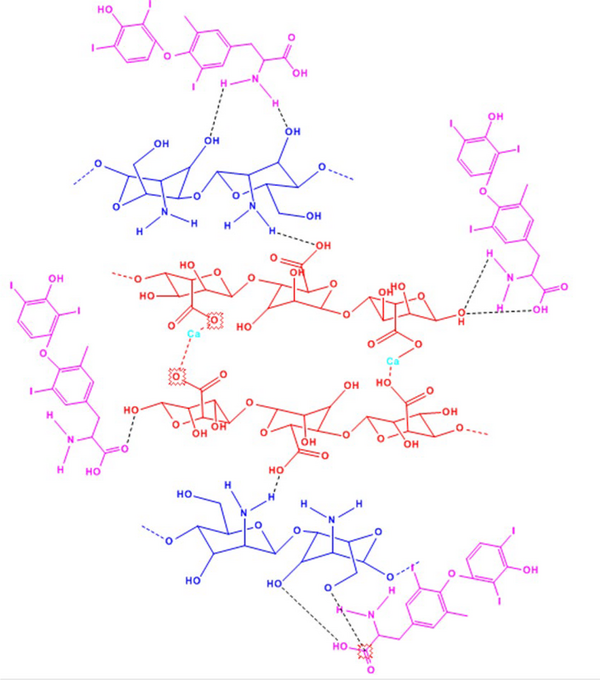
3.2. FTIR Studies
FT-IR provides data on the atomic structure of carrier and drug compounds. The FT-IR spectra of (A) levothyroxine, (B) alginate, (C) chitosan, (D) alginate/chitosan NPs, and (E) ALG/CS NPs loaded with levothyroxine are shown in Figure 3. The broad peak in the range of 3400 - 3500 cm⁻¹ indicates the stretching vibrations of O-H or N-H groups, or both. The absorption peak near 1620 cm⁻¹ is associated with the -C=O group (amide-I) from chitosan and alginate. This peak decreases after chitosan is combined with alginate because the C=O groups convert to COO⁻ groups, which then interact electrostatically with the protonated amino groups of chitosan. The absorption peak at 1550 cm⁻¹ corresponds to the stretching vibration of C=O (amide-I) and the bending vibration of -NH₂ (amine I). The peak at 1300 cm⁻¹ is related to the stretching of the C-N vibration. The absorption peak in the 1000 - 1200 cm⁻¹ region indicates C–O stretching bonds, and a decrease in the OH phenolic bending peak at 1200 - 1400 cm⁻¹ is also observed in ALG/CS NPs (23, 41-43). The best peak chosen for the quantification of levothyroxine is the C=C stretch band, which was observed at 1409.27 cm⁻¹ (1463 - 1364 cm⁻¹), where the obtained peak is intense and does not interfere with other spectral peaks, with very good correlation coefficients (6).
FTIR Spectra of A, levothyroxine; B, alginate sodium; C, chitosan; D, alginate/chitosan nanoparticle and E, levothyroxine loaded alginate/chitosan
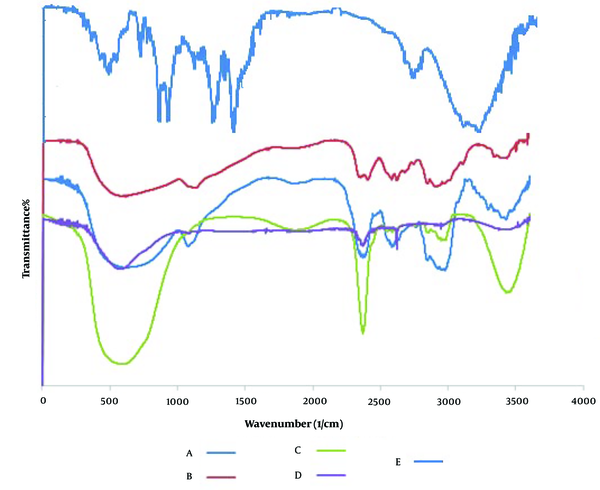
3.3. Transmission Electron Microscopy
The morphology of the manufactured ALG/CS NPs and levothyroxine-loaded ALG/CS NPs was examined using TEM images. Figure 4 shows the spherical shape of ALG/CS NPs with a diameter of around 90 nm. The TEM image revealed a spherical shape and soft surface of the NPs. The size of ALG/CS NPs is approximately 102 nm, as shown in the histogram, and the polydispersity index of ALG/CS NPs was about 0.047 (Figure 5). The particles are in the range of 80 - 110 nm. All the data confirmed one another.
Transmission electron microscopy (TEM) image of alginate-chitosan nanoparticles (ALG/CS NPs)

Dynamic light scattering (DLS) analysis of NPs
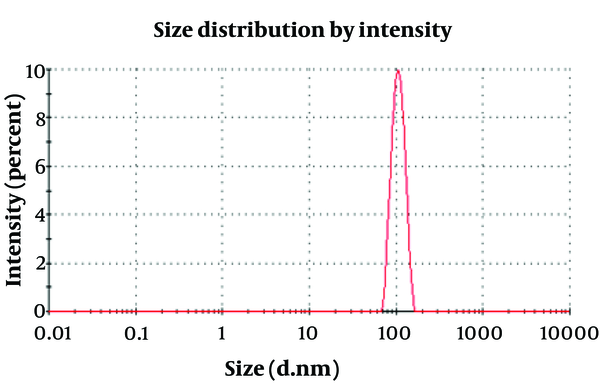
3.4. In Vitro Drug Release
Levothyroxine is a drug with poor water solubility and high lipophilicity, which makes it suitable for encapsulation within ALG/CS NPs (1-5). In general, for individuals with hypothyroidism, levothyroxine therapy should be initiated at full replacement doses as soon as possible. Delays in diagnosis and the institution of therapy may have serious effects on the child’s intellectual and physical growth and development. In this study, the in vitro release of levothyroxine from ALG/CS NPs was studied for 72 hours at 37°C using a Franz diffusion cell. Levothyroxine release from ALG/CS NPs was investigated at 37°C in phosphate buffer saline (0.01 M, pH 7.4), as shown in Figure 4. The results indicate that ALG/CS NPs released the majority of levothyroxine within the first 20 hours, with an average release rate of up to 78% at pH 7.4. The initial burst release of ALG/CS NPs within the first 12 hours can be explained by the rapid diffusion of levothyroxine from the outer shell of the ALG/CS NPs core system. After 12 hours, the drug diffused from the core of the ALG/CS NPs. This release profile is illustrated in Figure 6.
Levothyroxine release of alginate-chitosan nanoparticles (ALG/CS NPs)
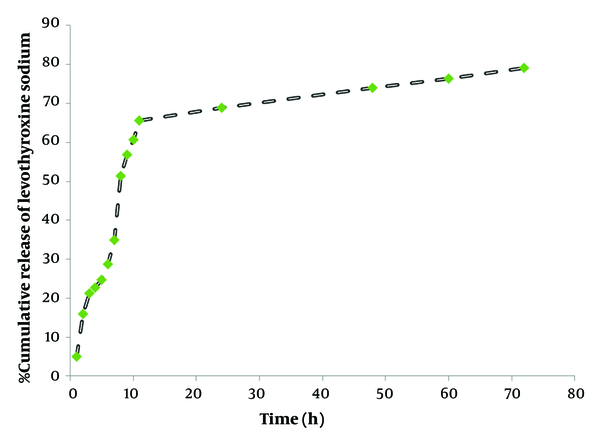
3.5. In Vitro Drug Release Kinetic Characterization
Scientific models such as first-order, Higuchi, zero-order, and Korsmeyer–Peppas equations were utilized to evaluate the fit of the obtained data (Table 1).
Kinetic Models of Drug Release
Kinetic Models | Equation | Parameters |
---|---|---|
Zero-order | C = K0t | C (concentration), K0 (zero-order rate constant), t (time) |
First-order | Log C = Log C0-kt/2.303 | C0 (initial concentration of the drug), k (first-order rate constant), t (time) |
Higuchi | Q = K t 1/2 | Q (cumulative percentage drug released), K (constant), t (time) |
Hixson-Crowell | W0 (initial amount of drug in the pharmaceutical dosage form), W (remaining amount of drug in the pharmaceutical dosage form), t (time), K (constant incorporating the surface-volume relation) |
4. Discussion
The release data were analyzed using the Higuchi kinetic equation. According to the release model of such formulations, it was clear that the prolonged release characteristic of levothyroxine was well fitted to Higuchi’s square root model, as has been reported for ALG/CS NPs systems (39-41). The regression coefficient (R²) values of the release data for all formulations obtained by the curve-fitting method on various kinetic models are reported in Table 2. Linear fits were obtained, indicating that the release profile of levothyroxine sodium from the homogenous and granular matrix system is diffusion-controlled. The R² values from in vitro release kinetics, along with the K values or release rate constants obtained from the Higuchi model plot, are presented in Table 2. The R² values of the release data for all formulations obtained by the curve-fitting method for zero-order, first-order, Higuchi model, Korsmeyer–Peppas, and Hixson-Crowell models are also reported in Table 2 (43). For the optimized formulation F, the R² value of 0.998 for the Higuchi model (closer to 1) is the most probable model compared to the others, indicating that the drug release is determined by the square root of time.
The R2 Values From in Vitro Release Kinetics and the K-Values or Release Rate Constant a, b
Formulas Code | Zero-Order | First Order | Higuchi Model | Hixson-Crowell | Krosmeyer-Peppas Model | |
---|---|---|---|---|---|---|
R2 | R2 | R2 | - | R | n | |
F | 0.922 ± 0.0024 | 0.939 ± 0.0042 | 0.998 ± 0.0014 | 0.934 ± 0.0053 | 0.912 ± 0.0031 | 0.425±0.0034 |
K | 4.24 ± 0.013 | 0.054 ± 0.015 | 0.01 ± 0.010 | 0.016 ± 0.020 | 0.895 ± 0.018 |
4.1. Conclusions
Complexes of chitosan and alginate loaded with levothyroxine as a nanocarrier were prepared using Rajaonarivony's method. FTIR spectra demonstrated functional groups and the interactions between alginate and chitosan when loaded with levothyroxine. The size and shape of the nanocarrier were examined by transmission electron microscopy, and the size distribution and polydispersity index were determined using photon correlation spectroscopy. The results showed that ALG/CS NPs were spherical with a diameter of approximately 80 - 110 nm. Alginate-chitosan nanoparticles were prepared by ionotropic pre-gelation of the chitosan core, followed by alginate polyelectrolyte complexation. The results indicated that the release mechanism is dependent on dissolution during the initial hours. For levothyroxine release from ALG/CS NPs, the Higuchi model provided a better fit than other models. The findings suggest that ALG/CS NPs can be utilized for controlled drug release in studies on therapeutic effects. The excellent properties of ALG/CS NPs expand the application range of hydrophobic drugs by enhancing their water solubility.
Acknowledgements
References
-
1.
Kashanian S, Rostami E, Harding FJ, McInnes SJ, Al-Bataineh S, Voelcker NH. Controlled Delivery of Levothyroxine Using Porous Silicon as a Drug Nanocontainer. Australian Journal of Chemistry. 2016;69(2). https://doi.org/10.1071/ch15315.
-
2.
Liu H, Li W, Zhang W, Sun S, Chen C. Levothyroxine: Conventional and Novel Drug Delivery Formulations. Endocr Rev. 2023;44(3):393-416. [PubMed ID: 36412275]. [PubMed Central ID: PMC10166268]. https://doi.org/10.1210/endrev/bnac030.
-
3.
Baxter JD, Webb P. Thyroid hormone mimetics: potential applications in atherosclerosis, obesity and type 2 diabetes. Nat Rev Drug Discov. 2009;8(4):308-20. [PubMed ID: 19337272]. https://doi.org/10.1038/nrd2830.
-
4.
Bjoro T, Holmen J, Kruger O, Midthjell K, Hunstad K, Schreiner T, et al. Prevalence of thyroid disease, thyroid dysfunction and thyroid peroxidase antibodies in a large, unselected population. The Health Study of Nord-Trondelag (HUNT). Eur J Endocrinol. 2000;143(5):639-47. [PubMed ID: 11078988]. https://doi.org/10.1530/eje.0.1430639.
-
5.
Melzer D, Rice N, Depledge MH, Henley WE, Galloway TS. Association between serum perfluorooctanoic acid (PFOA) and thyroid disease in the U.S. National Health and Nutrition Examination Survey. Environ Health Perspect. 2010;118(5):686-92. [PubMed ID: 20089479]. [PubMed Central ID: PMC2866686]. https://doi.org/10.1289/ehp.0901584.
-
6.
AlBathish M, Gazy A, Al Jamal M, Bejjani A. Utilization of the FTIR spectroscopic method for the quantitative determination of the narrow therapeutic index levothyroxine sodium in pharmaceutical tablets. Pharmacia. 2024;71:1-9. https://doi.org/10.3897/pharmacia.71.e125879.
-
7.
Pearce EN, Farwell AP, Braverman LE. Thyroiditis. N Engl J Med. 2003;348(26):2646-55. [PubMed ID: 12826640]. https://doi.org/10.1056/NEJMra021194.
-
8.
Rostami E, Kashanian S, Azandaryani AH. Preparation of solid lipid nanoparticles as drug carriers for levothyroxine sodium with in vitro drug delivery kinetic characterization. Mol Biol Rep. 2014;41(5):3521-7. [PubMed ID: 24515386]. https://doi.org/10.1007/s11033-014-3216-4.
-
9.
Duntas LH, Jonklaas J. Levothyroxine Dose Adjustment to Optimise Therapy Throughout a Patient's Lifetime. Adv Ther. 2019;36(Suppl 2):30-46. [PubMed ID: 31485977]. [PubMed Central ID: PMC6822824]. https://doi.org/10.1007/s12325-019-01078-2.
-
10.
Skelin M, Lucijanic T, Amidzic Klaric D, Resic A, Bakula M, Liberati-Cizmek AM, et al. Factors Affecting Gastrointestinal Absorption of Levothyroxine: A Review. Clin Ther. 2017;39(2):378-403. [PubMed ID: 28153426]. https://doi.org/10.1016/j.clinthera.2017.01.005.
-
11.
Kocic I, Homsek I, Dacevic M, Grbic S, Parojcic J, Vucicevic K, et al. A case study on the in silico absorption simulations of levothyroxine sodium immediate-release tablets. Biopharm Drug Dispos. 2012;33(3):146-59. [PubMed ID: 22374714]. https://doi.org/10.1002/bdd.1780.
-
12.
Bach-Huynh TG, Nayak B, Loh J, Soldin S, Jonklaas J. Timing of levothyroxine administration affects serum thyrotropin concentration. J Clin Endocrinol Metab. 2009;94(10):3905-12. [PubMed ID: 19584184]. [PubMed Central ID: PMC2758731]. https://doi.org/10.1210/jc.2009-0860.
-
13.
Green WL. New questions regarding bioequivalence of levothyroxine preparations: a clinician's response. AAPS J. 2005;7(1):E54-8. [PubMed ID: 16146330]. [PubMed Central ID: PMC2751497]. https://doi.org/10.1208/aapsj070107.
-
14.
Khoshbakht S, Asghari-Sana F, Fathi-Azarbayjani A, Sharifi Y. Fabrication and characterization of tretinoin-loaded nanofiber for topical skin delivery. Biomaterials Research. 2020;24(1):8. https://doi.org/10.1186/s40824-020-00186-3.
-
15.
Azarbayjani AF, Venugopal JR, Ramakrishna S, Lim PF, Chan YW, Chan SY. Smart polymeric nanofibers for topical delivery of levothyroxine. J Pharm Pharm Sci. 2010;13(3):400-10. [PubMed ID: 21092712]. https://doi.org/10.18433/j3ts3g.
-
16.
Moghassemi S, Hadjizadeh A. Nano-niosomes as nanoscale drug delivery systems: an illustrated review. J Control Release. 2014;185:22-36. [PubMed ID: 24747765]. https://doi.org/10.1016/j.jconrel.2014.04.015.
-
17.
Brown TD, Whitehead KA, Mitragotri S. Materials for oral delivery of proteins and peptides. Nature Reviews Materials. 2019;5(2):127-48. https://doi.org/10.1038/s41578-019-0156-6.
-
18.
Chow EK, Ho D. Cancer nanomedicine: from drug delivery to imaging. Sci Transl Med. 2013;5(216):216rv4. [PubMed ID: 24353161]. https://doi.org/10.1126/scitranslmed.3005872.
-
19.
Ma L, Tu C, Le P, Chitoor S, Lim SJ, Zahid MU, et al. Multidentate Polymer Coatings for Compact and Homogeneous Quantum Dots with Efficient Bioconjugation. J Am Chem Soc. 2016;138(10):3382-94. [PubMed ID: 26863113]. https://doi.org/10.1021/jacs.5b12378.
-
20.
Sarmento B, Ribeiro AJ, Veiga F, Ferreira DC, Neufeld RJ. Insulin-loaded nanoparticles are prepared by alginate ionotropic pre-gelation followed by chitosan polyelectrolyte complexation. J Nanosci Nanotechnol. 2007;7(8):2833-41. [PubMed ID: 17685304]. https://doi.org/10.1166/jnn.2007.609.
-
21.
Shi J, Alves NM, Mano JF. Chitosan coated alginate beads containing poly(N-isopropylacrylamide) for dual-stimuli-responsive drug release. J Biomed Mater Res B Appl Biomater. 2008;84(2):595-603. [PubMed ID: 17618514]. https://doi.org/10.1002/jbm.b.30907.
-
22.
Tonnesen HH, Karlsen J. Alginate in drug delivery systems. Drug Dev Ind Pharm. 2002;28(6):621-30. [PubMed ID: 12149954]. https://doi.org/10.1081/ddc-120003853.
-
23.
Feyissa Z, Edossa GD, Gupta NK, Negera D. Development of double crosslinked sodium alginate/chitosan based hydrogels for controlled release of metronidazole and its antibacterial activity. Heliyon. 2023;9(9). e20144. [PubMed ID: 37809897]. [PubMed Central ID: PMC10559936]. https://doi.org/10.1016/j.heliyon.2023.e20144.
-
24.
Nalini T, Basha S, Mohamed Sadiq A, Kumari V, Kaviyarasu K. Development and characterization of alginate / chitosan nanoparticulate system for hydrophobic drug encapsulation. J Drug Delivery Sci Technol. 2019;52:65-72. https://doi.org/10.1016/j.jddst.2019.04.002.
-
25.
Alnaief M, Obaidat RM, Alsmadi MM. Preparation of Hybrid Alginate-Chitosan Aerogel as Potential Carriers for Pulmonary Drug Delivery. Polymers (Basel). 2020;12(10). [PubMed ID: 32992662]. [PubMed Central ID: PMC7601040]. https://doi.org/10.3390/polym12102223.
-
26.
Li P, Dai YN, Zhang JP, Wang AQ, Wei Q. Chitosan-alginate nanoparticles as a novel drug delivery system for nifedipine. Int J Biomed Sci. 2008;4(3):221-8. [PubMed ID: 23675094]. [PubMed Central ID: PMC3614711].
-
27.
d'Ayala GG, Malinconico M, Laurienzo P. Marine derived polysaccharides for biomedical applications: chemical modification approaches. Molecules. 2008;13(9):2069-106. [PubMed ID: 18830142]. [PubMed Central ID: PMC6245343]. https://doi.org/10.3390/molecules13092069.
-
28.
Guarino V, Caputo T, Altobelli R, Ambrosio L. Degradation properties and metabolic activity of alginate and chitosan polyelectrolytes for drug delivery and tissue engineering applications. AIMS Materials Science. 2015;2(4):497-502. https://doi.org/10.3934/matersci.2015.4.497.
-
29.
Cunha AG, Gandini A. Turning polysaccharides into hydrophobic materials: a critical review. Part 2. Hemicelluloses, chitin/chitosan, starch, pectin and alginates. Cellulose. 2010;17(6):1045-65. https://doi.org/10.1007/s10570-010-9435-5.
-
30.
Jayakumar R, Menon D, Manzoor K, Nair SV, Tamura H. Biomedical applications of chitin and chitosan based nanomaterials—A short review. Carbohydrate Polymers. 2010;82(2):227-32. https://doi.org/10.1016/j.carbpol.2010.04.074.
-
31.
Bhunchu S, Rojsitthisak P. Biopolymeric alginate-chitosan nanoparticles as drug delivery carriers for cancer therapy. Pharmazie. 2014;69(8):563-70. [PubMed ID: 25158565].
-
32.
Sarmento B, Ribeiro A, Veiga F, Sampaio P, Neufeld R, Ferreira D. Alginate/chitosan nanoparticles are effective for oral insulin delivery. Pharm Res. 2007;24(12):2198-206. [PubMed ID: 17577641]. https://doi.org/10.1007/s11095-007-9367-4.
-
33.
Singh G, Kulharia M. Insights from the analysis of alginate lyase protein model from Pseudomonas fluorescens towards the understanding of mucoid biofilm disruption. Bioinformation. 2017;13(9):318-22. [PubMed ID: 29081612]. [PubMed Central ID: PMC5651226]. https://doi.org/10.6026/97320630013318.
-
34.
Nayak PL, Sahoo D. Chitosan-alginate composites blended with cloisite 30B as a novel drug delivery system for anticancer drug paclitaxel. International Journal of Plastics Technology. 2011;15(1):68-81. https://doi.org/10.1007/s12588-011-9000-6.
-
35.
Tapdiqov S, Taghiyev D, Zeynalov N, Safaraliyeva S, Fatullayeva S, Hummetov A, et al. Cumulative release kinetics of levothyroxine-Na pentahydrate from chitosan/arabinogalactane based pH sensitive hydrogel and it's toxicology. Reactive and Functional Polymers. 2022;178:105334. https://doi.org/10.1016/j.reactfunctpolym.2022.105334.
-
36.
Dash M, Chiellini F, Ottenbrite RM, Chiellini E. Chitosan—A versatile semi-synthetic polymer in biomedical applications. Progress in Polymer Science. 2011;36(8):981-1014. https://doi.org/10.1016/j.progpolymsci.2011.02.001.
-
37.
Choi B, Kollias N, Zeng H, Kang HW, Wong BJ, Ilgner JF, et al. Photonic monitoring of chitosan nanostructured alginate microcapsules for drug release. Photonic Therapeutics and Diagnostics XI. 2015.
-
38.
Rajabiyan A, Shakiba Maram N, Ghatrami ER, Zarei Ahmady A. Preparation of magnetic methotrexate nanocarrier coated with extracted hydroxyapatite of sea urchin (Echinometra mathaei). Main Group Chemistry. 2021;20(4):447-61. https://doi.org/10.3233/mgc-210043.
-
39.
Obeid MA, Khadra I, Albaloushi A, Mullin M, Alyamani H, Ferro VA. Microfluidic manufacturing of different niosomes nanoparticles for curcumin encapsulation: Physical characteristics, encapsulation efficacy, and drug release. Beilstein J Nanotechnol. 2019;10:1826-32. [PubMed ID: 31579065]. [PubMed Central ID: PMC6753676]. https://doi.org/10.3762/bjnano.10.177.
-
40.
Collier JW, Shah RB, Bryant AR, Habib MJ, Khan MA, Faustino PJ. Development and application of a validated HPLC method for the analysis of dissolution samples of levothyroxine sodium drug products. J Pharm Biomed Anal. 2011;54(3):433-8. [PubMed ID: 20947276]. [PubMed Central ID: PMC3090652]. https://doi.org/10.1016/j.jpba.2010.08.025.
-
41.
Kiti K, Suwantong O. Bilayer wound dressing based on sodium alginate incorporated with curcumin-beta-cyclodextrin inclusion complex/chitosan hydrogel. Int J Biol Macromol. 2020;164:4113-24. [PubMed ID: 32896559]. https://doi.org/10.1016/j.ijbiomac.2020.09.013.
-
42.
Ngwabebhoh FA, Zandraa O, Patwa R, Saha N, Capakova Z, Saha P. Self-crosslinked chitosan/dialdehyde xanthan gum blended hypromellose hydrogel for the controlled delivery of ampicillin, minocycline and rifampicin. Int J Biol Macromol. 2021;167:1468-78. [PubMed ID: 33212108]. https://doi.org/10.1016/j.ijbiomac.2020.11.100.
-
43.
Rade PP, Giram PS, Shitole AA, Sharma N, Garnaik B. Physicochemical and in Vitro Antibacterial Evaluation of Metronidazole Loaded Eudragit S-100 Nanofibrous Mats for the Intestinal Drug Delivery. Advanced Fiber Materials. 2021;4(1):76-88. https://doi.org/10.1007/s42765-021-00090-y.