Abstract
Background:
Thymus extracts have become attractive targets for screening bioactive compounds with potential applications in pharmaceuticals, food, and cosmetics industries.Objectives:
This is the first report assessing the anticholinesterase and antioxidant activities, along with total phenolic and flavonoid contents (TPC and TFC), of hydroethanolic extracts from six populations of Thymus caramanicus Jalas at vegetative and flowering stages, collected from different regions in Kerman province, Iran.Methods:
The anticholinesterase and antioxidant activity, as well as TPC and TFC of T. caramanicus hydroethanolic extracts, were evaluated using Ellman, DPPH, Folin-Ciocalteu, and aluminum chloride assays, respectively.Results:
All extracts inhibited both acetylcholinesterase (AChE) and butyrylcholinesterase (BuChE) enzymes, with most showing a stronger effect on AChE. The extract T2-F exhibited the highest anti-AChE (IC50: 28.74 ± 0.21 µg/mL) and anti-BuChE (IC50: 65.49 ± 0.81 µg/mL) activities. The DPPH results indicated overall suitable antioxidant activity, with T4-F showing the highest antioxidant capacity (IC50: 16.22 ± 0.07 µg/mL), though about 4.2 times lower than ascorbic acid. The TPC (76.60 ± 0.98 - 211.73 ± 2.13 mg GAE/g extract) and TFC (19.15 ± 0.09 - 53.90 ± 0.13 mg QE/g extract) analyses revealed that T6-V and T5-F are rich sources of polyphenolic and flavonoid compounds. Pearson’s correlation analysis showed no direct correlation between TPC and TFC with their anticholinesterase and antioxidant activities.Conclusions:
The hydroethanolic extracts of T. caramanicus demonstrated significant anticholinesterase and antioxidant activities, making them promising candidates for further studies aimed at developing therapeutic compounds with dual anticholinesterase and antioxidant properties, potentially for treating Alzheimer's disease (AD).Keywords
Thyme Thymus caramanicus Jalas Anticholinesterase Activity Antioxidant Activity Alzheimer's Disease (AD)
1. Background
Alzheimer's disease (AD) is the most common form of dementia in individuals over 65 years old. This neurodegenerative disorder progresses gradually and eventually leads to death. Alzheimer's disease is characterized by impairments in memory, learning, and cognition, which result from the destruction of cholinergic neurons and the subsequent reduction in acetylcholine levels in the brain. Acetylcholine, a neurotransmitter, plays a crucial role in memory and learning processes.
The nervous system expresses two enzymes responsible for breaking down acetylcholine: Acetylcholinesterase (AChE) and butyrylcholinesterase (BuChE). However, the hydrolysis of acetylcholine by AChE occurs much faster than that by BuChE. In a healthy brain, AChE is the primary enzyme involved in acetylcholine metabolism (accounting for 80%), but evidence suggests that BuChE can partly compensate for the function of AChE. Interestingly, while the overall 3D structure of both enzymes, especially their active sites, is remarkably similar, their roles in the brain differ slightly, especially in the context of neurodegenerative diseases such as AD (1).
There is currently no definitive treatment for AD. The most common strategy to mitigate the cognitive symptoms of the disease and improve patients' quality of life is the prescription of cholinesterase inhibitors (ChEIs). These inhibitors are also used in the symptomatic treatment of other neurological disorders, such as Parkinson's disease (PD), Down’s syndrome, vascular dementia, and dementia with Lewy bodies (2). In recent years, there has been growing interest in studying natural resources to discover and develop new ChEIs (3-5).
The genus Thymus L. (known as "Avishan" in Persian), belonging to the Lamiaceae or mint family, consists of more than 350 species of aromatic plants with evergreen leaves. Of these, 18 species have been identified in Iran. One such species is Thymus caramanicus Jalas, an endemic species to Iran that thrives in the mountainous regions of Kerman, Yazd, and Isfahan. Although the members of the Thymus genus are distributed across Asia, North Africa, and Europe, the western Mediterranean region is considered their main origin (6, 7).
Plants of the Thymus genus have been used in traditional medicine for thousands of years. They exhibit a wide range of biological effects, including anti-inflammatory, antioxidant, antimicrobial, and pain-relieving properties. Additionally, they show potential for the treatment of various conditions, such as dementia and cancer (8-12).
In this research, the anticholinesterase and antioxidant activities, along with the total phenolic content (TPC) and total flavonoid content (TFC), of hydroethanolic extracts from six populations of T.caramanicus (in both vegetative and flowering stages) collected from different regions of Kerman province, Iran, have been investigated. While the anticholinesterase activity of other species of thyme has been studied, this is the first time that the anticholinesterase activity of T. caramanicus has been evaluated. Although there are reports on the antioxidant activity of methanolic extracts and essential oils from this plant, the antioxidant activity of hydroethanolic extracts of T. caramanicus is being explored for the first time.
2. Objectives
This study is significant because it evaluates several distinct populations of this species during both the vegetative and flowering stages, offering a comprehensive analysis of its bioactivity across different growth phases.
3. Methods
3.1. Chemicals
Acetylcholinesterase, BuChE, acetylthiocholine iodide, S-butyrylthiocholine iodide, neostigmine, Ellman's reagent (DTNB), DPPH, ascorbic acid, gallic acid, Folin-Ciocalteu reagent, and quercetin were provided by Sigma-Aldrich. KH2PO4, K2HPO4, AlCl3, methanol, and ethanol were purchased from Merck.
3.2. The Thymus caramanicus Populations and the Production of Hydroethanolic Extracts
Six populations of T. caramanicus (in both vegetative and flowering stages: Figure 1) (Appendix 1 in Supplementary File) were gathered from different regions of Kerman province, Iran. The collected aerial parts of the plants were dried at room temperature (25°C) and in dark conditions for 72 hours.
The flowering (A); and vegetative (B) stages of T2 sample
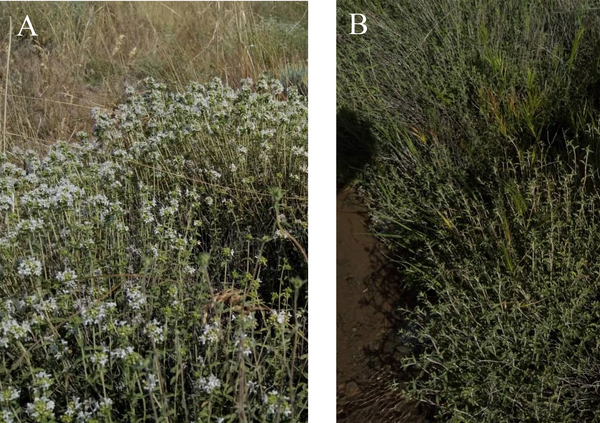
The hydroethanolic extracts of the plant samples were prepared as follows: Five grams of each dried powdered sample was soaked in 200 mL of 80% ethanol in a glass container. The mixture was continuously stirred using a magnetic stirrer for 24 hours at room temperature. After soaking, the ethanol solution was filtered through Whatman No. One filter paper to remove solid residues. The filtered solution was then concentrated using rotary evaporation at 40°C under reduced pressure (200 mbar). Finally, the extracts were dried completely in a vacuum oven at 40°C. The obtained extracts were stored in the dark at -20°C for future use.
3.3. Evaluation of Anticholinesterase Activity
The Ellman method (13), with some modifications (4), was used to evaluate the anticholinesterase activity of the extracts. The activity of the enzymes (AChE or BuChE) was measured in the absence and presence of six different concentrations of each T. caramanicus hydroethanolic extract (in 70% ethanol) and neostigmine (used as the positive control). All assays were conducted in a 96-well plate with a final volume of 200 μL per well. Each concentration was tested in triplicate to ensure reliability. The results were expressed as the IC50, which represents the concentration of extract required to inhibit 50% of AChE or BuChE activity.
3.4. Investigation of Antioxidant Activity
The 2,2-diphenyl-1-picrylhydrazyl (DPPH) method (14) was applied to assess the antioxidant activity of T. caramanicus extracts. 250 μL of each Thymus ethanolic extract in methanol was added to 750 μL of 0.4 mM DPPH solution. The mixture was incubated for 30 minutes at room temperature in the dark. After incubation, the sample's absorbance was measured at 517 nm. The DPPH solution initially has a deep violet color, which turns pale yellow or colorless as antioxidants react with the DPPH radicals.
For each sample, six different concentrations were analyzed, and all tests were performed in triplicate. The control sample contained 250 μL of methanol and 750 μL of DPPH solution (0.4 mM). Ascorbic acid was used as the positive control.
To determine the percentage of free radical DPPH inhibition by each sample concentration, the following formula was used:
Inhibition of DPPH (%) = [(Acontrol – Asample) /Acontrol] × 100
- Acontrol: Absorbance of the control sample
- Asample: Absorbance of the sample
The IC50 values were calculated by plotting a dose-response graph, which displayed the DPPH inhibition percentage versus the logarithm of the sample concentration.
3.5. Determination of Total Phenolic Content
The TPC of T. caramanicus hydroethanolic extracts was determined using the colorimetric Folin-Ciocalteu method (15), with gallic acid as the calibration standard. A mixture containing 100 µL of 0.2 N Folin-Ciocalteu reagent, 585 μL of distilled water, and 15 μL of the extract (in 80% ethanol, final concentration of 50 µg/mL) was prepared. After incubating for one minute at room temperature in the dark, 300 μL of 7.5% sodium carbonate was added to the mixture. The mixture was then incubated for an additional 30 minutes under the same conditions.
The resulting blue color was measured at an absorbance of 735 nm using a spectrophotometer. The blank sample consisted of 600 μL of distilled water, 100 μL of 0.2 N Folin-Ciocalteu reagent, and 300 μL of sodium carbonate (7.5%). Results were reported as milligrams of gallic acid equivalents (GAE) per gram of extract (mg GAE/g extract). Each sample was tested in triplicate to ensure reliability.
3.6. Determination of Total Flavonoid Content
The TFC of T. caramanicus extracts was measured using the aluminum chloride colorimetric method (16), with quercetin serving as the calibration standard. A solution containing 500 μL of the hydroethanolic extract in 75% ethanol (final concentration: 50 µg/mL) was mixed with 500 μL of 2% aluminum chloride. After incubating the mixture for 15 minutes at room temperature in the dark, the absorbance of the resulting yellow color was measured using a spectrophotometer at 435 nm. The blank sample consisted of 500 μL of distilled water and 500 μL of 2% aluminum chloride. Each sample was analyzed in triplicate. The TFC of the Thymus extracts was expressed in milligrams of quercetin equivalents (QE) per gram of extract (mg QE/g extract).
3.7. Statistical Analysis
The data were presented as arithmetical mean values with standard deviations. Pearson’s correlation was used to analyze the relationship between the TPC and TFC of the T. caramanicus hydroethanolic extracts and the studied parameters. Statistical analysis was conducted using the one-way ANOVA method via SPSS software (version 13.0).
4. Results and Discussion
4.1. Inhibition of Cholinesterase Enzymes (Acetylcholinesterase and Butyrylcholinesterase)
The IC50 values for AChE inhibition by T. caramanicus extracts ranged from 28.74 ± 0.21 μg/mL (T2-F) to 173.78 ± 2.05 μg/mL (T6-V), as shown in Table 1. Similarly, for BuChE inhibition, the T2-F extract exhibited the strongest activity (65.49 ± 0.81 μg/mL), while T6-V showed the weakest (1862.08 ± 10.01 μg/mL). A comparison of the inhibitory potential of the extracts against AChE and BuChE revealed that the extracts generally had a stronger inhibitory effect on AChE than on BuChE. For instance, the T5-V, T6-V, and T1-F extracts inhibited AChE 17.26, 10.72, and 6.63 times more effectively than BuChE, respectively.
The Ellman’s Assay Results
Samples | IC50 for AChE (µg/mL) | IC50 for BuChE (µg/mL) | Selectivity for AChE |
---|---|---|---|
T1-F | 91.47 ± 1.09 | 606.31 ± 5.81 | 6.63 |
T1-V | 39.97 ± 0.43 | 109.26 ± 1.25 | 2.73 |
T2-F | 28.74 ± 0.21 | 65.49 ± 0.81 | 2.28 |
T2-V | 40.93 ± 0.51 | 138.03 ± 2.01 | 3.37 |
T3-F | 45.86 ± 0.38 | 168.11 ± 2.11 | 3.67 |
T3-V | 63.51 ± 0.61 | 181.13 ± 2.72 | 2.85 |
T4-F | 51.2 ± 0.6 | 130.76 ± 1.81 | 2.59 |
T4-V | 45.14 ± 0.46 | 123.42 ± 1.75 | 2.73 |
T5-F | 95.49 ± 0.88 | 288.4 ± 3.1 | 3.02 |
T5-V | 31.11 ± 0.37 | 537.03 ± 4.83 | 17.26 |
T6-F | 49.15 ± 0.57 | 85.23 ± 0.76 | 1.73 |
T6-V | 173.78 ± 2.05 | 1862.08 ± 10.01 | 10.72 |
Neostigmine | 0.023 ± 0.001 | 0.04 ± 0.0005 | 0.57 |
A comparison between extracts from the flowering and vegetative stages showed mixed results, with some flowering extracts and some vegetative extracts performing better in terms of cholinesterase inhibition.
In summary, the findings indicate a significant anticholinesterase activity of the T. caramanicus extracts. As noted, this is the first report on the anticholinesterase activity of T. caramanicus, although similar activities have been reported in other Thymus species. For example, the essential oils and extracts of T. lotocephalus have demonstrated strong anti-AChE and anti-BuChE activities (17). In another study on alcoholic extracts of six Thymus species, all extracts exhibited AChE inhibition, with T. longicaulis, T. pulegioides, and T. vulgaris showing the most potent anticholinesterase activity (9).
4.2. 2,2-Diphenyl-1-Picrylhydrazyl Free Radical Scavenging Assay
The results of the DPPH free radical scavenging assay (Table 2) indicate that the antioxidant activity of the studied T. caramanicus extracts ranges from 16.22 ± 0.07 μg/mL to 63.09 ± 0.41 μg/mL. Among the extracts, the flowering extract of T4 (T4-F) exhibited the highest ability to inhibit DPPH free radicals, while the vegetative extract of T4 (T4-V) showed the lowest activity. When compared to the standard compound (ascorbic acid, IC50: 3.89 ± 0.03 μg/mL), the antioxidant activity of the extracts is considered suitable. The antioxidant activity of ascorbic acid is approximately 4.2 times higher than that of the most active extract (T4-F).
The Results of DPPH, Total Phenolic Content, and Total Flavonoid Content Assays
Samples | DPPH (IC50: µg/mL) | TPC (mg GAE/g Extract) | TFC (mg QE/g Extract) |
---|---|---|---|
T1-F | 35.48 ± 0.19 | 120.82 ± 1.1 | 34.7 ± 0.19 |
T1-V | 19.05 ± 0.09 | 115.54 ± 0.78 | 31.13 ± 0.11 |
T2-F | 34.67 ± 0.21 | 108.54 ± 1. 5 | 27.13 ± 0.21 |
T2-V | 32.35 ± 0.11 | 101.17 ± 0.67 | 20.29 ± 0.13 |
T3-F | 38.01 ± 0.15 | 142.94 ± 1.4 | 40.5 ± 0.15 |
T3-V | 39.81 ± 0.32 | 76.6 ± 0.98 | 19.15 ± 0.09 |
T4-F | 16.22 ± 0.07 | 113.45 ± 2.07 | 29.36 ± 0.17 |
T4-V | 63.09 ± 0.41 | 147.85 ± 1. 8 | 37.96 ± 0.31 |
T5-F | 34.1 ± 0.16 | 179.79 ± 2.16 | 47.95 ± 0.26 |
T5-V | 37.15 ± 0.22 | 98.71 ± 1.22 | 24.3 ± 0.12 |
T6-F | 34.8 ± 0.17 | 84.1 ± 0.28 | 20.4 ± 0.08 |
T6-V | 30.19 ± 0.13 | 211.73 ± 2.13 | 53.9 ± 0.13 |
Ascorbic acid | 3.89 ± 0.03 | - | - |
A more detailed analysis reveals that the IC50 values of most extracts (9 out of 12) are relatively close, indicating no significant difference in their antioxidant activities.
The antioxidant activity of various Thymus species has been well-documented in the literature (9, 18-20). Scientific evidence also supports the antioxidant effects of T. caramanicus. A study on the essential oil and methanolic extract of T. caramanicus aerial parts, using DPPH and ß-carotene/linoleic acid assays, demonstrated that the polar and non-polar subfractions of the methanolic extract exhibited the highest antioxidant activity in these assays, respectively (21). In another study, the strong antioxidant activity of T. caramanicus essential oil was confirmed through its use in improving the physiochemical properties of potato chips (22).
There is a confirmed relationship between oxidative stress and the occurrence of several well-known diseases and disorders, including neurodegenerative diseases, aging, cardiovascular diseases, rheumatoid arthritis, cancer, diabetes, and inflammation. Natural antioxidants, found in foods and medicinal plants, such as polyphenolic, flavonoid, terpenoid, and carotenoid compounds, can inhibit or delay the oxidation process through various mechanisms, including the inhibition of free radicals. These natural compounds play a crucial role in preventing or treating oxidative stress-related disorders (5, 23, 24).
In general, compounds with both anticholinesterase and antioxidant properties are considered promising candidates for AD treatment. This is because they simultaneously increase the available amount of acetylcholine in the brain and prevent the destructive effects of oxidative stress (25, 26). The results of this study demonstrate the good anticholinesterase and antioxidant activity of T. caramanicus hydroethanolic extracts. Therefore, these extracts hold promise for further studies aimed at discovering medicinal compounds with both anticholinesterase and antioxidant effects, which may be beneficial for the treatment of AD.
4.3. Total Phenolic Content and Total Flavonoid Content
As shown in Table 2, the TPC of the T. caramanicus extracts ranges from 211.73 ± 2.13 mg GAE/g extract (T6-V) to 76.60 ± 0.98 mg GAE/g extract (T3-V). Similarly, the TFC varies between 53.90 ± 0.13 mg QE/g extract (T6-V) and 19.15 ± 0.09 mg QE/g extract (T3-V). Besides T6-V, T5-F also exhibits high contents of polyphenolic (179.79 ± 2.16 mg GAE/g extract) and flavonoid (47.95 ± 0.26 mg QE/g extract) compounds. Comparing the TPC and TFC results, the extracts obtained from the flowering stages generally possess higher TPC and TFC, with the exceptions of T4 and T6. According to the literature, Thymus extracts are rich sources of phenolic and flavonoid compounds such as rosmarinic acid, salvianolic acids, luteolin, apigenin, myricetin, and kaempferol (19, 27, 28).
4.4. Analysis of the Correlation Between the Studied Parameters
As demonstrated in Table 3, there is a strong positive correlation between TPC and TFC (r = 0.977), indicating that extracts with higher TPC also tend to have higher TFC and vice versa. As mentioned earlier, Thymus extracts are rich in phenolic and flavonoid compounds, and several of their biological properties, such as antioxidant, antimicrobial, and anti-inflammatory activities, are attributed to these compounds (19, 29).
The Pearson’s Correlations Between Total Phenolic Content and Total Flavonoid Content of Thymus caramanicus Hydroethanolic Extracts and the Studied Parameters
However, the correlation analysis revealed no significant correlation between the antioxidant activity of T. caramanicus extracts and their TPC (r = 0.077) and TFC (r = 0.068). Interestingly, the anti-AChE activity of the extracts exhibited a moderate negative correlation with TPC (r = 0.755) and TFC (r = 0.727). Similarly, the anti-BuChE activity showed a moderate negative correlation with TPC (r = 0.670) and TFC (r = 0.617).
These findings may be due to the presence of carotenoid and terpene compounds in Thymus species (30), which are known to exhibit antioxidant (31, 32) and anticholinesterase activities (33, 34).
The results of a study on the antioxidant activity of hydroethanolic extracts from six Thymus species, assessed using six different assays, revealed that the TPC was only directly correlated with the results of the Reducing Power assay. No significant correlation was observed between the levels of polyphenolic compounds and the results of the other assays used to evaluate antioxidant activity. Additionally, the study did not find any considerable correlation between the anti-AChE activity and the polyphenol content of the extracts (9).
4.5. Conclusions
The results demonstrated that all T. caramanicus extracts are capable of inhibiting both AChE and BuChE enzymes, with most exhibiting a recognizable preference for AChE. The T2-F extract showed the highest inhibitory ability against both AChE and BuChE. The DPPH assay results indicated that the overall antioxidant activity of the studied extracts was suitable, with the most active extract (T4-F) exhibiting antioxidant activity approximately 4.2 times lower than the standard compound, ascorbic acid. The IC50 values of most extracts (9 out of 12) were close to each other, and no significant differences in antioxidant activity were observed.
The evaluation of TPC and TFC showed that the two extracts with the highest TPC and TFC levels (T6-V and T5-F) can be considered rich sources of phenolic and flavonoid compounds. However, Pearson’s correlation analysis indicated no direct correlation between the TPC and TFC of the extracts and their anticholinesterase and antioxidant activities.
In summary, the results of this study suggest that T. caramanicus hydroethanolic extracts possess suitable anticholinesterase and antioxidant activities. Therefore, these extracts are promising candidates for further research to explore drug agents with concurrent anticholinesterase and antioxidant properties for the treatment of AD and other neurological disorders.
Acknowledgements
References
-
1.
Lotfi S, Rahmani T, Hatami M, Pouramiri B, Kermani ET, Rezvannejad E, et al. Design, synthesis and biological assessment of acridine derivatives containing 1,3,4-thiadiazole moiety as novel selective acetylcholinesterase inhibitors. Bioorganic Chem. 2020;105. https://doi.org/10.1016/j.bioorg.2020.104457.
-
2.
Sharma K. Cholinesterase inhibitors as Alzheimer's therapeutics (Review). Mol Med Rep. 2019;20(2):1479-87. [PubMed ID: 31257471]. [PubMed Central ID: PMC6625431]. https://doi.org/10.3892/mmr.2019.10374.
-
3.
Dos Santos TC, Gomes TM, Pinto BAS, Camara AL, Paes AMA. Naturally Occurring Acetylcholinesterase Inhibitors and Their Potential Use for Alzheimer's Disease Therapy. Front Pharmacol. 2018;9:1192. [PubMed ID: 30405413]. [PubMed Central ID: PMC6201143]. https://doi.org/10.3389/fphar.2018.01192.
-
4.
Pishgouii F, Lotfi S, Sedaghati E. Anti-AChE and Anti-BuChE Screening of the Fermentation Broth Extracts from Twelve Aspergillus Isolates and GC-MS and Molecular Docking Studies of the Most Active Extracts. Appl Biochem Biotechnol. 2023;195(8):5199-216. [PubMed ID: 37129742]. https://doi.org/10.1007/s12010-023-04548-0.
-
5.
Fathi Hafshejani S, Lotfi S, Rezvannejad E, Mortazavi M, Riahi-Madvar A. Correlation between total phenolic and flavonoid contents and biological activities of 12 ethanolic extracts of Iranian propolis. Food Sci Nutr. 2023;11(7):4308-25. [PubMed ID: 37457164]. [PubMed Central ID: PMC10345684]. https://doi.org/10.1002/fsn3.3356.
-
6.
Bagheri R, Arjomand Tajadini MJ. [Allelopathic effects of Thymus caramanicus.Jalas on some vegetative indexes of Amygdalus scoparia Spach]. Iran J Med Aromatic Plant Res. 2011;27(2):261-70. FA. https://doi.org/10.22092/ijmapr.2011.6402.
-
7.
Talebian Z, Jafari AO, Yusefinejad M. [Morfometric Studies on the 14 species of Thymus L.(Lamiaceae) in Iran]. J Plant Res Biol. 2016;29(1):96-106. FA.
-
8.
Uritu CM, Mihai CT, Stanciu GD, Dodi G, Alexa-Stratulat T, Luca A, et al. Medicinal Plants of the Family Lamiaceae in Pain Therapy: A Review. Pain Res Manag. 2018;2018:7801543. [PubMed ID: 29854039]. [PubMed Central ID: PMC5964621]. https://doi.org/10.1155/2018/7801543.
-
9.
Kindl M, Blažeković B, Bucar F, Vladimir-Knežević S. Antioxidant and Anticholinesterase Potential of Six Thymus Species. Evid Base Complement Alternat Med. 2015;2015:10. [PubMed ID: 26351513]. https://doi.org/10.1155/2015/403950.
-
10.
Capatina L, Todirascu-Ciornea E, Napoli EM, Ruberto G, Hritcu L, Dumitru G. Thymus vulgaris Essential Oil Protects Zebrafish against Cognitive Dysfunction by Regulating Cholinergic and Antioxidants Systems. Antioxidants (Basel). 2020;9(11). [PubMed ID: 33158153]. [PubMed Central ID: PMC7694219]. https://doi.org/10.3390/antiox9111083.
-
11.
Rabiei Z, Mokhtari S, Asgharzade S, Gholami M, Rahnama S, Rafieian-kopaei M. Inhibitory effect of Thymus vulgaris extract on memory impairment induced by scopolamine in rat. Asia Pac J Trop Biomed. 2015;5(10):845-51. https://doi.org/10.1016/j.apjtb.2015.07.006.
-
12.
Kubatka P, Uramova S, Kello M, Kajo K, Samec M, Jasek K, et al. Anticancer Activities of Thymus vulgaris L. in Experimental Breast Carcinoma in Vivo and in Vitro. Int J Mol Sci. 2019;20(7). [PubMed ID: 30970626]. [PubMed Central ID: PMC6479806]. https://doi.org/10.3390/ijms20071749.
-
13.
Ellman GL, Courtney KD, Andres V, Feather-Stone RM. A new and rapid colorimetric determination of acetylcholinesterase activity. Biochem Pharmacol. 1961;7:88-95. [PubMed ID: 13726518]. https://doi.org/10.1016/0006-2952(61)90145-9.
-
14.
Gulcin İ, Alwasel SH. DPPH Radical Scavenging Assay. Processes. 2023;11(8). https://doi.org/10.3390/pr11082248.
-
15.
Pérez M, Dominguez-López I, Lamuela-Raventós RM. The Chemistry Behind the Folin-Ciocalteu Method for the Estimation of (Poly)phenol Content in Food: Total Phenolic Intake in a Mediterranean Dietary Pattern. J Agric Food Chem. 2023;71(46):17543-53. [PubMed ID: 37948650]. [PubMed Central ID: PMC10682990]. https://doi.org/10.1021/acs.jafc.3c04022.
-
16.
Magalhães LM, Almeida MG, Barreiros L, Reis S, Segundo MA. Automatic Aluminum Chloride Method for Routine Estimation of Total Flavonoids in Red Wines and Teas. Food Analytic Meth. 2011;5(3):530-9. https://doi.org/10.1007/s12161-011-9278-1.
-
17.
Costa P, Gonçalves S, Grosso C, Andrade PB, Valentão P, Bernardo-Gil MG, et al. Chemical profiling and biological screening of Thymus lotocephalus extracts obtained by supercritical fluid extraction and hydrodistillation. Ind Crops Product. 2012;36(1):246-56. https://doi.org/10.1016/j.indcrop.2011.09.014.
-
18.
Gedikoglu A, Sokmen M, Civit A. Evaluation of Thymus vulgaris and Thymbra spicata essential oils and plant extracts for chemical composition, antioxidant, and antimicrobial properties. Food Sci Nutr. 2019;7(5):1704-14. [PubMed ID: 31139383]. [PubMed Central ID: PMC6526640]. https://doi.org/10.1002/fsn3.1007.
-
19.
Afonso AF, Pereira OR, Cardoso SM. Health-Promoting Effects of Thymus Phenolic-Rich Extracts: Antioxidant, Anti-Inflammatory and Antitumoral Properties. Antioxidants (Basel). 2020;9(9). [PubMed ID: 32882987]. [PubMed Central ID: PMC7555682]. https://doi.org/10.3390/antiox9090814.
-
20.
Rafique S, Murtaza MA, Hafiz I, Ameer K, Qayyum MMN, Yaqub S, et al. Investigation of the antimicrobial, antioxidant, hemolytic, and thrombolytic activities of Camellia sinensis, Thymus vulgaris, and Zanthoxylum armatum ethanolic and methanolic extracts. Food Sci Nutr. 2023;11(10):6303-11. [PubMed ID: 37823136]. [PubMed Central ID: PMC10563746]. https://doi.org/10.1002/fsn3.3569.
-
21.
Safaei-Ghomi J, Ebrahimabadi AH, Djafari-Bidgoli Z, Batooli H. GC/MS analysis and in vitro antioxidant activity of essential oil and methanol extracts of Thymus caramanicus Jalas and its main constituent carvacrol. Food Chem. 2009;115(4):1524-8. https://doi.org/10.1016/j.foodchem.2009.01.051.
-
22.
Boroujeni LS, Hojjatoleslamy M. Using Thymus carmanicus and Myrtus communis essential oils to enhance the physicochemical properties of potato chips. Food Sci Nutr. 2018;6(4):1006-14. [PubMed ID: 29983964]. [PubMed Central ID: PMC6021700]. https://doi.org/10.1002/fsn3.597.
-
23.
Wang X, Sankarapandian K, Cheng Y, Woo SO, Kwon HW, Perumalsamy H, et al. Relationship between total phenolic contents and biological properties of propolis from 20 different regions in South Korea. BMC Complement Altern Med. 2016;16:65. [PubMed ID: 26888665]. [PubMed Central ID: PMC4758169]. https://doi.org/10.1186/s12906-016-1043-y.
-
24.
Rahaman MM, Hossain R, Herrera-Bravo J, Islam MT, Atolani O, Adeyemi OS, et al. Natural antioxidants from some fruits, seeds, foods, natural products, and associated health benefits: An update. Food Sci Nutr. 2023;11(4):1657-70. [PubMed ID: 37051367]. [PubMed Central ID: PMC10084981]. https://doi.org/10.1002/fsn3.3217.
-
25.
Kashyap P, Choudhary H, Kumar S. Dual anticholinesterase and antioxidant activity of selected plants used in Unani medicines and in silico studies of identified compounds from UPLC-QTOF analysis. J Herb Med. 2023;41. https://doi.org/10.1016/j.hermed.2023.100709.
-
26.
Liu P, Cheng M, Guo J, Cao D, Luo J, Wan Y, et al. Dual functional antioxidant and butyrylcholinesterase inhibitors for the treatment of Alzheimer's disease: Design, synthesis and evaluation of novel melatonin-alkylbenzylamine hybrids. Bioorg Med Chem. 2023;78:117146. [PubMed ID: 36580744]. https://doi.org/10.1016/j.bmc.2022.117146.
-
27.
Kontogiorgis C, Ntella M, Mpompou L, Karallaki F, Athanasios P, Hadjipavlou-Litina D, et al. Study of the antioxidant activity of Thymus sibthorpii Bentham (Lamiaceae). J Enzyme Inhib Med Chem. 2016;31(sup4):154-9. [PubMed ID: 27612190]. https://doi.org/10.1080/14756366.2016.1222583.
-
28.
Anwar F, Mehmood T, Qadir R, Riaz M; Mahrye. Phenolics profiling and biological activities of different solvent extracts from aerial parts of wild thyme (Thymus vulgaris L.). J Food Measure Charact. 2021;16(1):610-8. https://doi.org/10.1007/s11694-021-01185-1.
-
29.
Nieto G. A Review on Applications and Uses of Thymus in the Food Industry. Plants (Basel). 2020;9(8). [PubMed ID: 32751488]. [PubMed Central ID: PMC7464319]. https://doi.org/10.3390/plants9080961.
-
30.
Zakrzewski A, Purkiewicz A, Jakuć P, Wiśniewski P, Sawicki T, Chajęcka-Wierzchowska W, et al. Effectiveness of various solvent-produced thyme (Thymus vulgaris) extracts in inhibiting the growth of Listeria monocytogenes in frozen vegetables. Nutr Food Sci J. 2022;29:26-34. https://doi.org/10.1016/j.nfs.2022.09.004.
-
31.
Andarwulan N, Cahyarani Puspita N, Średnicka-Tober D; Saraswati. Antioxidants Such as Flavonoids and Carotenoids in the Diet of Bogor, Indonesia Residents. Antioxidants (Basel). 2021;10(4). [PubMed ID: 33920414]. [PubMed Central ID: PMC8069321]. https://doi.org/10.3390/antiox10040587.
-
32.
Masyita A, Mustika Sari R, Dwi Astuti A, Yasir B, Rahma Rumata N, Emran TB, et al. Terpenes and terpenoids as main bioactive compounds of essential oils, their roles in human health and potential application as natural food preservatives. Food Chem X. 2022;13:100217. [PubMed ID: 35498985]. [PubMed Central ID: PMC9039924]. https://doi.org/10.1016/j.fochx.2022.100217.
-
33.
Bakare OO, Fadaka AO, Akanbi MO, Akinyede KA, Klein A, Keyster M. Evaluation of selected carotenoids of Lycopersicon esculentum variants as therapeutic targets for ‘Alzheimer’s disease: an in silico approach. BMC Mol Cell Biol. 2021;22(1). https://doi.org/10.1186/s12860-021-00386-2.
-
34.
Lai Shi Min S, Liew SY, Chear NJY, Goh BH, Tan WN, Khaw KY. Plant Terpenoids as the Promising Source of Cholinesterase Inhibitors for Anti-AD Therapy. Biol. 2022;11(2). https://doi.org/10.3390/biology11020307.