Abstract
Background:
Celecoxib is a selective cyclo-oxygenase-2 inhibitor recommended orally to treat arthritis and osteoarthritis. It is a highly lipophilic, poorly soluble drug with oral bioavailability of around 40%. Long term oral administration of celecoxib causes serious gastrointestinal side effects.Objectives:
The current study aimed to assess the skin permeation of celecoxib by a transdermally applied liposomal formulation.Materials and Methods:
Liposomes were prepared by thin film method using soya lecithin and cholesterol. Physicochemical characteristics of the liposomes such as, particle size, drug encapsulation efficiency were determined. Also, drug release and in vitro skin permeability through rat skin were evaluated using Franz diffusion cells.Results:
The results showed that the maximum drug encapsulation efficiency was 43.24%. Drug release profile showed that 81.25% of the drugs released in the first 24 hours of the experiment. Fluxes (Jss), permeability coefficient (P), diffusivity coefficient (D) parameters of the optimum liposomal formulation were significantly higher than those of saturated aqueous solution of celecoxib. The decrease of lecithin increased values. Particle sizes of the formulations ranged from 0.117 to 1.123 µm. Jss, Dapp and P parameters in L - 8 formulations were 29.18, 60.95, and 3.21 times higher than those of saturated water solution of celecoxib, respectively. The results of vesicles characterization indicated the potential application of celecoxib loaded liposome as carrier system.Conclusions:
In conclusion, the components such as lecithin and cholesterol, and vortex time in liposomal formulations have an essential role in the physicochemical properties and celecoxib permeability through rat skin.Keywords
1. Background
Human skin is an important target site for application of drugs. Permeation of drugs through the skin is the basis of transdermal and topical delivery (1). Drug permeation across different skin layers is affected by various factors such as physicochemical properties of the drug vehicle, and formulation components. Transdermal drug delivery has some benefits such as controlled drug delivery avoidance of the first pass metabolism, continuous drug delivery, and facilitation of drug localization at target site (1). Celecoxib (CXB) is a highly selective COX-2 inhibitor and primarily inhibits the isoform of cyclooxygenase, thus causes inhibition of prostaglandin production which involves in inflammatory response (2). The drug is more selective for COX-2 (IC50 = 0.04 µM) inhibition if compared with COX-1 (IC50 = 15 µM) (3). It binds with its polar sulfonamide side chain to a hydrophilic side pocket region close to the active COX-2 binding site (2). Non-specific- non –Steroidal anti-inflammatory drugs(NSAIDs) generally inhibits both cyclooxygenase 1 and 2 (COX-1 and COX-2) which are important for the regulation of homeostasis in many tissues. The inhibition of COX-1 activity results in a number of side effects such as gastro-sensitivity (4) and interference with platelet function (5). CXB is highly effective in the treatment of osteoarthritis and rheumatoid arthritis when compared to other NSAIDS, for example naproxen and diclofenac (6, 7). CXB is also used to treat pain and inflammation associated with ankylosing spondylitis, as well as menstrual cramps and colonic polyps (2, 8). The amide group present in its structure is weakly acidic with a pKa value of about 11. Therefore, CXB is insoluble at physiological pH. This is no liquid CXB formulation, and its only available oral dosage form is capsule (8). Although the absorption of CXB provided in capsule is delayed by food, the drug systemic exposure increases by 3 - 5 folds (9). CXB exhibits poor flow properties and compressibility (10). The drug is highly hydrophobic and is almost completely absorbed after oral administration. However, much of the drug is metabolized by liver during its first passage through portal circulation (11). CXB has the high volume of tissue distribution of 455 ± 166 liters, indicating an extensive permeation into a number of organs (12, 13). Since it bounds to plasma protein at the rate of 97% (14), oral CXB formulations require to be administered at high daily doses, thereby increasing concerns about cardiovascular side effects (15). Thus the problems associated with oral administration made us design the novel drug delivery system for CXB with an alternate route of administration. Novel drug delivery carriers such as liposomes are very versatile to suit the delivery of various drug molecules (16). Liposomes selected for the encapsulation of CXB in liposomes may reduce the drug associated side effects by reducing the availability of the drug in systemic circulation and increasing its accumulation in the sites of inflammation, possibly by extra vascularization through the gaps formed between the endothelial cells of the vasculature. The current work includes the preparation of CXB liposomes by examining the influence of various formulation and process parameters. Further various characteristics such as the rate of drug encapsulation, vesicle size, vesicle size distribution, drug leakage profile, and in vitro drug release pattern were assessed in an attempt to design various liposomal formulations of celecoxib for topical and transdermal application. The present study was an attempt to design various liposomal formulations of celecoxib for topical and transdermal application. Here, in vitro permeation of celecoxib from liposomal formulations was evaluated and, then compared with aqueous saturated solution of celecoxib.
2. Objectives
The current study aimed to prepare liposome containing celecoxib and evaluate the factors that can affect their physicochemical properties.
3. Materials and Methods
3.1. Materials
Soya lecithin and cholesterol were purchased from Acros (USA) and Merck (Germany), respectively. Celecoxib was kindly donated by Exir pharmaceutical Co., Iran. All of the solvents were of the analytical grade. Dialysis bag was purchased from the Teb Azma Co. (Tehran, Iran). Minitab 16 software was used to generate and evaluate the experimental design, and evaluate the effect of variables on responses.
3.2. Animal Model
Male adult Wistar rats, weighing 150-170 g and aged 10-12 weeks, were purchased from Animal Research Center, Jundishapur University of Medical Sciences, Ahvaz, Iran. The hair on the abdominal skin was removed with an electric clipper, taking care not to damage the skin. The rats were anaesthetized with ether prior to scarifying. Abdominal full-thickness skin was removed and any extraneous subcutaneous fats cleaned from the dorsal side using cooled pure acetone solution in 4ºC. Whole skin thickness was measured using a digital micrometer (AAOC, France) (17).
3.3. Methods
3.3.1. Celecoxib Assay
The amount of Celecoxib was measured by ultra violet (UV) spectrophotometer apparatus (BioWaveII, WPA) at 252 nm.
3.3.2. Preparation of Liposome
Several parameters influence the final properties of liposomes. Major variables in the liposome properties include cholesterol and lecithin amounts and vortex time. Full factorial design was used concerning three variables at two levels for formulations. Eight different formulations with low and high values of cholesterol (190 and 380 mg), lecithin (410 and 820 mg), and vortex time (5 and 15 minutes) were used to prepare liposomal formulations.
Liposomes were prepared by thin film method. Briefly, different concentrations of soya lecithin and cholesterol (Table 1) were dissolved in the chloroform-methanol (1:1) and 5 mg celecoxib was added to the solution, then the mixture was evaporated in a rotary evaporator when the thin film was formed in the round-bottoms flask, it was hydrated with phosphate buffer (pH 7.4). The suspension was agitated by vortex for 30 minutes and then sonicated for one hour (18-20).
Compositions of the Selected Liposomes Containing Celecoxib
Formulation, No. | Celecoxib, mg | Lecithin, mg | Cholesterol, mg | Vortex Time, min |
---|---|---|---|---|
L - 1 | 50 | 820 | 380 | 15 |
L - 2 | 50 | 820 | 380 | 5 |
L - 3 | 50 | 820 | 190 | 15 |
L - 4 | 50 | 820 | 190 | 5 |
L - 5 | 50 | 410 | 380 | 15 |
L - 6 | 50 | 410 | 380 | 5 |
L - 7 | 50 | 410 | 190 | 15 |
L - 8 | 50 | 410 | 190 | 5 |
3.3.3. Particle Size Determination
The average diameter and polydispersity index (PDI) of liposomes were determined using a Particle Sizer Qudix, ScatterO Scope I system (Korea) at 25ºC (18).
3.3.4. Encapsulation Efficiency (EE)
Encapsulation efficiency was determined as the ratio of celecoxib encapsulated in liposome to the original amount of celecoxib added. Free celecoxib was separated from liposome by centrifugation using ultracentrifuge (optima L-100 xp, USA) at 20,000 rpm, and 25ºC for 30 minutes. To determine drug efficiency of liposome, lipid vesicles were destroyed using 100% Triton X-100 (Baker analyzed, USA). Concisely, 100 µL of liposomal suspension was added to 100 µL of 100% Triton X-100 and vortexed for five minutes to ease lysis of the liposomal encapsulated celecoxib. The encapsulation efficacy was calculated by the following formula:

Where Qe was the amount of encapsulated celecoxib and Qt the amount of celecoxib present in 100 µL of liposomes (21).
3.3.5. Drug Release Study
Franz diffusion cells (area 3.4618 cm2) with a cellulose membrane were used to determine the release rate of celecoxib from different liposomal formulations. The cellulose (molecular weight G12000) membrane was first hydrated in distilled water solution at 25ºC for 24 hours. The membrane was then fixed between the donor and receptor compartments of the cell. The receptor chamber contained 22 mL of methanol-phosphate buffer pH 7.4 (2:1) and was continually stirred using a magnet stirrer (300 rpm) at 37ºC. Two milliliters of the sample was withdrawn from each batch at definite time intervals (0.5, 1, 2, 3, 4, 5, 6, 24) and replaced with the same amount of buffer phosphate to maintain skin condition. The release concentrations of celecoxib were determined by UV visible spectrophotometer (BioWaveII, WPA) at 252 nm. The results were plotted as cumulative released drug percent versus time (22). Drug release from liposomal formulations was explained by fitting on kinetic models in which commonly used models such as zero order, first order, second order, 3/2 root of mass, linear and log Wagner, Hixson-Crowell, Weibull, Korsmeyer-Peppas, Higuchi models, and the model with higher r2 were selected (15).
3.3.6. In Vitro Skin Permeation Studies
In vitro skin permeation studies were carried out using vertical glass diffusion cells fabricated in house with an effective diffusion area of approximately 3.46 cm (23). The volume of the receptor compartment was 22 mL. Whole skin sample, hydrated prior to use, was mounted between the donor and receptor compartments of the cell with the stratum corneum facing the donor medium. A 5 mg liposome sample, was placed in the donor compartment and the receptor cell was filled with methanol-phosphate buffer pH 7.4 (2:1). The diffusion cell was mounted and clamped in a water bath 37 ± 0.50ºC. The receptor chamber was stirred continuously with the help of a magnetic bead at 300 rpm. At predetermined time intervals (0.5, 1, 2, 3, 4, 5, 6, 24, 28, 32, 48, 52, and 72 hours), a 2 mL sample was withdrawn from the receptor medium and immediately replaced with an equivalent volume of fresh receptor medium to the maintained sink condition. The samples were filtered and the permeated amounts of CXB were analyzed by UV spectroscopy method at 252 nm.
3.3.7. Data Analysis of Skin Permeation and Statistics
The cumulative amount of Celecoxib permeated per unit skin area was calculated and plotted against time. The skin permeation rate at steady state (Jss) was determined from the linear portion of the slope of the permeation curve. A one-way analysis of variance (ANOVA) was used to see any significant differences and P < 0.05 was the level of significance with 95% confidence intervals. All statistical analyses were conducted using SPSS software (version 16.0). All the experiments were repeated three times and data were expressed as the mean value ± SD. Different permeability parameters were measured through permeation studies that include flux (Jss), permeability coefficient (P), lag time (Tlag) and diffusivity coefficient (D). Since the skin thickness (h) does not show the real pathway for drug permeation then diffusivity coefficient is defined as appearance D.P and D parameters were calculated from the equations (Jss = P × C0) and (D = h2/6 Tlag), respectively. The enhancement ratio (ER) was calculated to find the relative enhancement in the permeability parameters.
Amount of liposome formulations was measured based on the control (drug saturated solution) permeability parameters. The enhancement ratio was estimated according to the equation (Enhancement Ratio (ER) = permeability parameter amount of formulation/ permeability parameter amount of control).
4. Results
The encapsulation of different formulations is shown in Table 2 and Figure 1. The percent of CXB release from the liposome formulation was found from 34.36 to 81.25. The 24-hour cumulative amount of celecoxib that had permeated through the cellulose membrane (%) was plotted as a function of time (hour). In the current study, L-7 and L-4 had the highest and lowest accumulative release percent, respectively. Table 3 shows release percent and kinetic of release from celecoxib-loaded liposomes. Multivariate regression was used to analyze the correlation between independent variables and the drug released from liposomes. Analysis of variance showed no significant correlation between release percentage value of celecoxib and independent variables (P > 0.05).
The release profile of liposomes was evaluated by fitting the experimental data to equations describing different kinetic models (Figure 2). Linear regression analyses were made for zero-order (Mt/M0 = kt), first - order (ln (M0 - Mt) = kt), Higuchi (Mt/M0 = (kt)1/2), Log Wagner, Linear Wagner Second root of mass, Three - Seconds root of mass, Peppas and Weibul kinetics. Comparing the amounts of the released celecoxib after 24 hours indicated that the L - 7 formulation release kinetic model follows Firstequation. Release kinetic models of different liposomal formulations are shown in Table 3.
The mean particle size of formulations was from 0.1 to 1.12 µm (Table 2). The L-4 formulation had the lowest average particle size 0.117 ± 0.039 µm, and the L-6 formulation had the highest average particle size 1.12 ± 0.187 µm. Analysis of variance showed significant correlation between particle size and amount of lecithin (P < 0.05); therefore, particle size increased with decrease in the amount of lecithin. Figure 3 shows the permeation profiles of celecoxib through excised rat skins from various liposomes. The permeability parameters of various liposomal formulations are indicated in Table 4.
Loading Profile of Celecoxib Liposomal Formulations
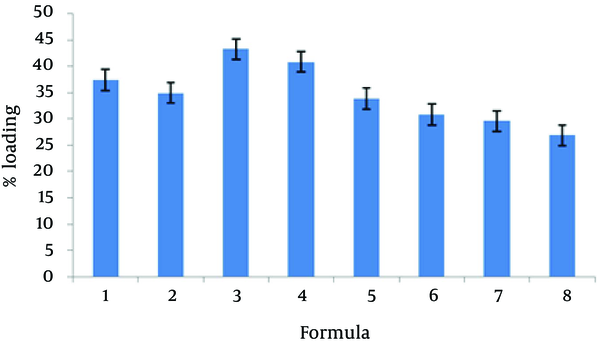
In-Vitro Release Profile of Celecoxib Liposomal Formulations
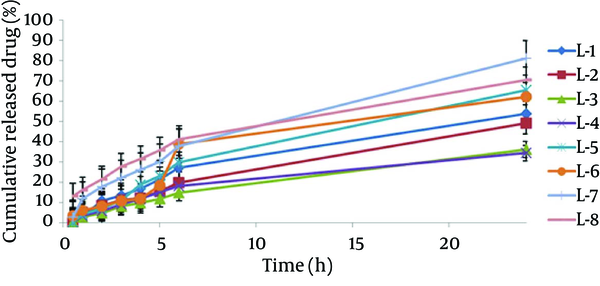
Permeation Profiles of Celecoxib From Various Liposomeal Formulations Through Excised Rat Skin
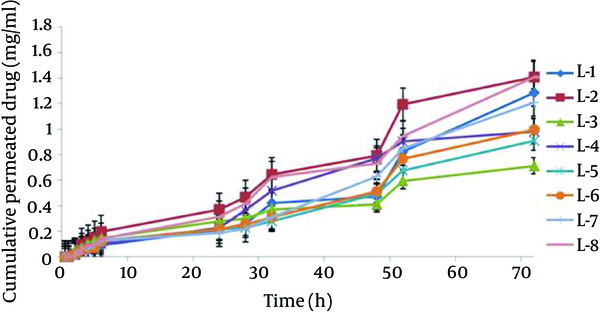
Particle Size, Polydispersity Index and Drug Loading Percent of the Selected Celecoxib Liposomal Formulations a
Formulation, No | Factorial Design Condition | Particle Size, µm | Polydispersity | Drug Loading, % |
---|---|---|---|---|
L - 1 | + + + | 0.66 ± 0.13 | 0.34 ± 0.027 | 37.37 ± 2.55 |
L - 2 | - + + | 0.816 ± 0.09 | 0.36 ± 0.03 | 34.91 ± 1.83 |
L - 3 | + - + | 0.687 ± 0.16 | 0.33 ± 0.017 | 43.24 ± 1.15 |
L - 4 | - - + | 0.117 ± 0.039 | 0.37 ± 0.054 | 40.84 ± 0.24 |
L - 5 | + + - | 0.53 ± 0.07 | 0.36 ± 0.036 | 33.83 ± 2.93 |
L - 6 | - + - | 1.123 ± 0.187 | 0.34 ± 0.047 | 30.77 ± 1.55 |
L - 7 | - - - | 0.892 ± 0.07 | 0.37 ± 0.017 | 29.54 ± 0.37 |
L - 8 | + - - | 0.897 ± 0.75 | 0.34 ± 0.009 | 26.83 ± 0.96 |
Release % (R2) | r2 | Kinetic Model Release | Release % (R24) | Formulation, No |
---|---|---|---|---|
10.78 ± 0.32 | 0.9875 | Higuchi | 53.8 ± 0.5 | L - 1 |
8.39 ± 0.28 | 0.997 | First | 49.02 ± 0.28 | L - 2 |
4.80 ± 0.17 | 0.9962 | Higuchi | 36.01 ± 0.48 | L - 3 |
5.67 ± 0.56 | 0.9858 | Log Wagner | 34.36 ± 0.95 | L - 4 |
7.08 ± 0.23 | 0.9929 | First | 65.37 ± 0.42 | L - 5 |
8.21 ± 0.15 | 0.9273 | Pepas | 62.04 ± 0.32 | L - 5 |
17.88 ± 0.5 | 0.9978 | First | 81.25 ± 0.37 | L - 7 |
21.48 ± 0.2 | 0.9915 | Higuchi | 70.39 ± 0.4 | L - 8 |
In Vitro Permeability Parameters of Celecoxib of Aqueous Saturated Solution (Control) and Various Liposome Formulations Through Excised Rat Skins a
Formulation, No | Jss, mg/cm2 h | Tlag, h | Dapp, cm2/h | P (cm/h) | ERflux | ERD | ERp |
---|---|---|---|---|---|---|---|
Control | 0.0033 ± 0.00028 | 5.765 ± 0.098 | 0.00007 ± 0.0000027 | 0.007 ± 0.825 | |||
L - 1 | .095 ± .011 | 0.12 ± 0.09 | 0.061 ± 0.031 | 0.017 ± 0.11 | 9.49 ± 28.11 | 1034.24 ± 876.18 | 0.013 ± 0.021 |
L - 2 | 0.0929 ± 0.058 | 1.43 ± 0.81 | 0.0082 ± 0.012 | 0.026 ± 0.016 | 17.69 ± 28.07 | 183.29 ± 117.14 | 0.020 ± 0.031 |
L - 3 | 0.041 ± 0.024 | 0.331 ± 0.194 | 0.0032 ± 0.0027 | 0.0092 ± 0.0049 | 7.35 ± 12.41 | 39.23 ± 46.18 | 0.0060 ± 0.011 |
L - 4 | 0.117 ± 0.089 | 0.47 ± 0.45 | 0.0027 ± 0.0021 | 0.028 ± 0.021 | 27.21 ± 35.55 | 30.04 ± 39.04 | 0.025 ± 0.034 |
L - 5 | 0.118 ± 0.085 | .341 ± .229 | 0.0038 ± 0.0037 | 0.034 ± 0.025 | 25.96 ± 35.85 | 56.61 ± 54.76 | 0.031 ± 0.042 |
L - 6 | 0.0214 ± 0.0067 | 0.565 ± 0.108 | 0.0012 ± 0.0002 | 0.0069 ± 0.0022 | 4.06 ± 9.82 | 4.12 ± 18.09 | 0.0027 ± 0.0083 |
L - 7 | 0.093 ± 0.086 | 0.146 ± 0.121 | 0.0109 ± 0.011 | 0.0815 ± 0.0417 | 28.47 ± 29.82 | 163.63 ± 155.71 | 0.035 ± 0.038 |
L - 8 | 0.0963 ± 0.039 | 0.222 ± 0.133 | 0.0042 ± 0.0025 | 0.036 ± 0.014 | 11.84 ± 29.18 | 36.17 ± 60.95 | 0.329 ± 0.225 |
5. Discussion
Celecoxib is a new anti-inflammatory drug that acts on cyclooxygenase-2 and also has antitumor properties. Liposomes are used as drug carriers for a wide range of drugs and are important in pharmaceutical field due to decrease of side effects. Liposome incorporates hydrophilic drugs through an aqueous core or entraps hydrophobic drugs using phospholipid bilayers which surround the aqueous core (18). Liposomes as bimolecular phospholipid bilayers are capable of encapsulating hydrophobic, hydrophilic, and amphiphilic drugs. Liposomes by diffusing into the stratum corneum, disrupting the bilayer fluidity in the stratum corneum, loosening the lipid structure of the stratum corneum, and providing impaired barrier function of these layers to the drug act as penetration enhancers.
According to the current study results, the best loading drug was 43.24% belonging to L-3 formulation. Many other studies indicated that the liposomes were suitable systems for loading drugs, for example, the mean encapsulation efficiency of diclofenac sodium via conventional liposomes was 42.62 ± 3.62% (22).
The current study analysis of variance showed significant correlation between released celecoxib percent after two hours (R2) and the amount of lecithin and cholesterol (P < 0.05). Therefore, with decrease in the amount of lecithin and cholesterol, R2 increased (Equation 1).

The current study analysis of variance showed significant correlation between particle size and the amount of lecithin. Therefore, particle size increased with increase in the amount of lecithin, (P < 0.05). Increase in the amount of lecithin increased loading percentage (P < 0.05). In permeability studies, the correlation between P and the amount of lecithin was significant and indirect (P < 0.05). Hence, decrease in the amount of lecithin increased P parameters .The Jss of celecoxib from L-8 was 0.0963 ± 0.039 mg cm-2 h-1, 29.18 times higher than those of saturated aqueous solution of celecoxib. Jss, Dapp and P parameters in L-8 formulation were 29.18, 60.95, and 3.21 times higher than those of saturated water solution of celecoxib, respectively. In conclusion, the amount of components in liposome formulation plays an essential role in the physicochemical properties and celecoxib permeability through rat skin. Also, liposome containing celecoxib would be a suitable means to protect and permeate the drug.
Multivariate regression was applied for the analysis of correlation between independent variables and liposomes skin permeability parameters. Linear equations which shows for flux (Jss), Tlag, Dapp and P are:




Analysis of variance indicated that correlation between independent variables and Tlag , Dapp and Jss parameters of celecoxib formulations were not significant (P > 0.05) and, analysis of variance showed significant correlation between independent variables and P parameter of celecoxib formulations (P < 0.05). On the basis of Equation 3, the correlation between P parameters was significant with the amount of lecithin in liposomal formulations, therefore, decrease in the amount of lecithin increased P parameters.
Acknowledgements
References
-
1.
Hadgraft J, Lane ME. Skin permeation: the years of enlightenment. Int J Pharm. 2005;305(1-2):2-12. [PubMed ID: 16246513]. https://doi.org/10.1016/j.ijpharm.2005.07.014.
-
2.
Rao P, Knaus EE. Evolution of nonsteroidal anti-inflammatory drugs (NSAIDs): cyclooxygenase (COX) inhibition and beyond. J Pharm Pharm Sci. 2008;11(2):81s-110s. [PubMed ID: 19203472].
-
3.
Seedher N, Bhatia S. Solubility enhancement of Cox-2 inhibitors using various solvent systems. AAPS PharmSciTech. 2003;4(3):E33. [PubMed ID: 14621965]. https://doi.org/10.1208/pt040333.
-
4.
Schoen RT, Vender RJ. Mechanisms of nonsteroidal anti-inflammatory drug-induced gastric damage. Am J Med. 1989;86(4):449-58. [PubMed ID: 2648824].
-
5.
Schafer AI. Effects of nonsteroidal antiinflammatory drugs on platelet function and systemic hemostasis. J Clin Pharmacol. 1995;35(3):209-19. [PubMed ID: 7608308].
-
6.
Simon LS, Lanza FL, Lipsky PE, Hubbard RC, Talwalker S, Schwartz BD, et al. Preliminary study of the safety and efficacy of SC-58635, a novel cyclooxygenase 2 inhibitor: efficacy and safety in two placebo-controlled trials in osteoarthritis and rheumatoid arthritis, and studies of gastrointestinal and platelet effects. Arthritis Rheum. 1998;41(9):1591-602. [PubMed ID: 9751091]. https://doi.org/10.1002/1529-0131(199809)41:9<1591::AID-ART9>3.0.CO;2-J.
-
7.
Baboota S, Shakeel F, Ahuja A, Ali J, Shafiq S. Design, development and evaluation of novel nanoemulsion formulations for transdermal potential of celecoxib. Acta Pharm. 2007;57(3):315-32. [PubMed ID: 17878111]. https://doi.org/10.2478/v10007-007-0025-5.
-
8.
Ventura CA, Giannone I, Paolino D, Pistara V, Corsaro A, Puglisi G. Preparation of celecoxib-dimethyl-beta-cyclodextrin inclusion complex: characterization and in vitro permeation study. Eur J Med Chem. 2005;40(7):624-31. [PubMed ID: 15935897]. https://doi.org/10.1016/j.ejmech.2005.03.001.
-
9.
Paulson SK, Vaughn MB, Jessen SM, Lawal Y, Gresk CJ, Yan B, et al. Pharmacokinetics of celecoxib after oral administration in dogs and humans: effect of food and site of absorption. J Pharmacol Exp Ther. 2001;297(2):638-45. [PubMed ID: 11303053].
-
10.
Paradkar AR, Chauhan B, Yamamura S, Pawar AP. Preparation and characterization of glassy celecoxib. Drug Dev Ind Pharm. 2003;29(7):739-44. [PubMed ID: 12906331]. https://doi.org/10.1081/DDC-120021773.
-
11.
Clemett D, Goa KL. Celecoxib: a review of its use in osteoarthritis, rheumatoid arthritis and acute pain. Drugs. 2000;59(4):957-80. [PubMed ID: 10804043].
-
12.
Davies NM, McLachlan AJ, Day RO, Williams KM. Clinical pharmacokinetics and pharmacodynamics of celecoxib: a selective cyclo-oxygenase-2 inhibitor. Clin Pharmacokinet. 2000;38(3):225-42. [PubMed ID: 10749518]. https://doi.org/10.2165/00003088-200038030-00003.
-
13.
Walter MF, Jacob RF, Day CA, Dahlborg R, Weng Y, Mason RP. Sulfone COX-2 inhibitors increase susceptibility of human LDL and plasma to oxidative modification: comparison to sulfonamide COX-2 inhibitors and NSAIDs. Atherosclerosis. 2004;177(2):235-43. [PubMed ID: 15530895]. https://doi.org/10.1016/j.atherosclerosis.2004.10.001.
-
14.
Paulson SK, Kaprak TA, Gresk CJ, Fast DM, Baratta MT, Burton EG, et al. Plasma protein binding of celecoxib in mice, rat, rabbit, dog and human. Biopharm Drug Dispos. 1999;20(6):293-9. [PubMed ID: 10701700].
-
15.
Solomon SD, McMurray JJ, Pfeffer MA, Wittes J, Fowler R, Finn P, et al. Cardiovascular risk associated with celecoxib in a clinical trial for colorectal adenoma prevention. N Engl J Med. 2005;352(11):1071-80. [PubMed ID: 15713944]. https://doi.org/10.1056/NEJMoa050405.
-
16.
Pai RS, Devi KV. Lamivudine liposomes for Transdermal Delivery-Formulation, characterization, Stability, and In-vitro Evaluation. Int J Pharm Sci Nanotechnol. 2009;1:317-26.
-
17.
Moghimipour E, Salimi A, Sharifmakhmalzadeh B. Effect of the Various Solvents on the In Vitro Permeability of Vitamin B 12 through Excised Rat Skin. Trop J Pharm Res. 2013;12(5):671-7.
-
18.
Moghimipour E, Handali S. Utilization of thin film method for preparation of celecoxib loaded liposomes. Adv Pharm Bull. 2012;2(1):93-8. [PubMed ID: 24312776]. https://doi.org/10.5681/apb.2012.013.
-
19.
Chetanachan P, Akarachalanon P, Worawirunwong D, Dararutana P, Bangtrakulnonth A, Bunjop M, et al. Ultrastructural Characterization of Liposomes Using Transmission Electron Microscope. Adv Mat Res. 2008;55:709-11. https://doi.org/10.4028/www.scientific.net/AMR.55-57.709.
-
20.
Mohammadi-Samani S, Montaseri H, Jamshidnejad M. Preparation and evaluation of cyproterone acetate liposome for topical drug delivery. Iran J Pharm Sci. 2009;5(4):199-204.
-
21.
Padamwar MN, Pokharkar VB. Development of vitamin loaded topical liposomal formulation using factorial design approach: drug deposition and stability. Int J Pharm. 2006;320(1-2):37-44. [PubMed ID: 16707237]. https://doi.org/10.1016/j.ijpharm.2006.04.001.
-
22.
Moghimipour E, Salimi A, Eftekhari S. Design and characterization of microemulsion systems for naproxen. Adv Pharm Bull. 2013;3(1):63-71. [PubMed ID: 24312814]. https://doi.org/10.5681/apb.2013.011.
-
23.
Roberts MS, Cross SE. Percutaneous absorption of topically applied NSAIDS and other compounds: role of solute properties, skin physiology and delivery systems. Inflammopharmacology. 1999;7(4):339-50. [PubMed ID: 17657437]. https://doi.org/10.1007/s10787-999-0028-6.