Abstract
Background:
Activation of polyol pathway, due to increased aldose reductase (ALR2) activity, is one of the several mechanisms that have been involved in the development of secondary complications of diabetes. Therefore, ALR2 inhibition has been recognized as a potential therapeutic goal in the prevention and treatment of various secondary complications of diabetes, such as cataract.Objectives:
In this study, we aimed to evaluate the inhibitory effects of hydro alcoholic Capparis spinosa extract (CSE) on ALR2 and sorbitol accumulation in red blood cells (RBCs).Materials and Methods:
The hydroalcoholic extract of Capparis spinosa (C. spinosa) was prepared and its inhibitory effects were evaluated by using the partial purified bovine lens ALR2 and its specificity was checked by comparing with its inhibitory effect on aldehyde reductase (ALR1), the other member of aldo-keto reductases family. Because RBC sorbitol accumulation is strongly correlated with the blood glucose level, the effect of CSE on sorbitol accumulation in human RBCs, preincubated with high serum glucose concentration, was determined.Results:
The CSE inhibited ALR2 activity, with an IC50 of 0.54 mg/mL and it was seven and a half times greater than that of ALR1 inhibition, indicating the specificity of CSE for ALR2, rather than ALR1. Also, CSE, at a concentration of 0.54 mg/mL, could reduce sorbitol accumulation in erythrocytes, by 46.8%.Conclusions:
These results demonstrated that CSE, mainly through inhibition of ALR2, could prevent or delay the diabetic complications, like cataract formation, as a result of sorbitol accumulation.Keywords
Aldehyde Reductase Capparis Diabetes Complications Sorbitol Cataract Erythrocytes
1. Background
The World Health Organization estimated the number of people with diabetes is increasing and it will rise to 333 million, by 2025 (1). With the increasing prevalence of diabetes, its complications also rise. Particularly, prolonged chronic hyperglycemia may play an important role in the pathogenesis of diabetic complications, via several mechanisms, including increased activation of aldose reductase (ALR2) in the polyol pathway, advanced glycation end-products (AGEs), AGE receptor overexpression, activation of protein kinase C isoforms, activation of the glucosamine pathway and excessive oxidative stress. As a result, they can lead to various complications, such as cataract, retinopathy, nephropathy and neuropathy (2), representing the main causes of morbidity and mortality, in diabetic patients (3).
Polyol is a two-step metabolic pathway. First, in the presence of NADPH, glucose is converted to sorbitol by ALR2 and then sorbitol is converted to fructose, by sorbitol dehydrogenase, in the presence of NAD+. It is one of the most attractive candidate mechanisms to explain the pathogenesis of diabetic complications (4).
In cells, possessing ALR2, in which the glucose uptake takes place in an insulin-independent mechanism, under normoglycemic conditions, the flux through the pathway is limited by the relatively low cellular glucose concentration and the low affinity of ALR2 for glucose. Glucose, under these conditions, is profoundly metabolized by hexokinase. In hyperglycemia conditions, in these tissues, glucose levels are elevated, hexokinase is saturated and glucose metabolization by ALR2 will be increased (5).
In normal conditions, polyol pathway is a minor pathway of glucose utilization, accounting for less than 3% of glucose in the serum. However, in high serum glucose concentrations, more than 30% of glucose enters in this pathway and results in the accumulation of sorbitol, in tissues. When sorbitol accumulates, it causes oxidative and osmotic stresses on cells, by drawing more water into the cells, followed by tissue injury (6).
Aldehyde reductase (ALR1) is one of the aldo-keto reductase (AKR) supper family members that is closely related to ALR2 and is known to play a role in the detoxification of reactive aldehydes. Since multiple aldose reductase inhibitors (ARIs) inhibit both ALR2 and ALR1, so far, an ALR2 specific inhibitor has not been approved by clinical trials (7-9). Clinical studies show that natural therapies, especially plants containing flavonoids, by AR inhibitory and antioxidant effects, can prevent or slow down the diabetic complications (10).
The Capparis spinosa (C. spinosa) fruit, belonging to the Capparidaceae family, is a native Mediterranean perennate spontaneous shrub, which is also cultivated. In addition, it grows in the hot and dry climates, such as West and Central Asia, the Mediterranean region, and various parts of Iran (11, 12).
Certain parts of the capper plant, such as the flower buds, fruits, roots, and seeds, can be used as a drug or a cosmetic. The fruits are widely consumed raw or pickled. In traditional medicine, this plant is used as a diuretic and as way a treatment for gout, rheumatism and liver disease. Different studies have reported the analgesic, anti-hepatotoxic, anti-allergic, and hypoglycemic, hypolipidemic and anti-allergic effects (12-17).
Compounds of C. spinosa fruits include alkaloids, lipids, polyphenols, glucosinolates, and flavonoids, such as kaempferol, rutin, and quercetin (12). Furthermore, the C. spinosa fruit extract has glucose and lipids lowering effects (12-14).
In phytotherapy, the effect of the C. spinosa extract (CSE) on inhibiting aldose reductase, has never been demonstrated experimentally.
2. Objectives
The objective of this study was to evaluate the inhibitory effects of CSE on ALR2. The effects of CSE were compared with a positive control, sorbinil.
3. Materials and Methods
The nicotinamide adenine dinucleotide phosphate-oxidase (NADPH), nicotinamide adenine dinucleotide (NAD+), sorbinil, sorbitol, sorbitol dehydrogenase, DL-glyceraldehyde isoforms and Kreb’s-ringer bicarbonate buffer were purchased from Sigma-Aldrich (St. Louis, MO, USA). The D-Glucose, 2-mercaptoethanol, EDTA and sucrose were purchased from Merck KGaA, Darmstadt, Germany. All other chemicals and solvents were of analytical grade and were obtained from local companies.
3.1. Aldose Reductase and Aldehyde Reductase Purification
Crude ALR2 was prepared from bovine lens. Bovine eyes were collected on the day the animals were slaughtered in the slaughterhouse (Ahvaz, Iran). All stages were performed on ice, at 4°C. Immediately, lenses (200 g) were removed, from the eyes, and stored at -80°C. The lenses were homogenized in five volumes of 50 mM potassium phosphate buffer pH 7.2, containing 2-mercaptoethanol (5 mM) in homogenizer. The homogenate was centrifuged at 7500 g for 40 minutes, then the precipitate, containing cellular debris, and insoluble material, were removed and the resulting supernatant was used for precipitation, by ammonium sulfate, in concentrations of 40%, 50% and 75%, as described elsewhere (18). The precipitated enzyme was dissolved, in 5 mL of 50 mM potassium phosphate buffer pH 7.2, and dialyzed overnight, against the same buffer. After a stage of dialysis, the purified enzyme was concentrated against solid sucrose and, to remove sucrose, ammonium sulfate and other proteins, column chromatography (Sephadex G-25, GE Healthcare Life Sciences, Little Chalfont, United Kingdom) was applied. Cellulose (2.5 by 50 cm) was equilibrated and washed with the same buffer, until the absorbance of the elute, at 280 nm, was less than 0.1. The ALR1 was partially purified from bovine cortex kidney, following the previously described methods, except using diethylaminoethyl-cellulose-52 resin, to the removed material, and then centrifuged (19). The supernatant was used as the source of ALR1, for the inhibition studies.
3.2. Preparation of Hydro Alcoholic Capparis Spinosa Extract
Specimens of C. spinosa fruits were freshly collected from the Ilam region of South west of Iran. The plant was authenticated by The Department of Botany, Faculty of Agriculture, Shahid Chamran University, Ahvaz, Iran. The collected plant samples were dried, at room temperature and shade, then were powdered finely. The powdered samples were placed in a Soxhlet extraction apparatus, containing 70% hydroalcoholic solvent, for up to 6 hours, then were filtrated by Whatman No.1 filter paper (Sigma-Aldrich GmbH, Buchs, Switzerland). Then, the extract was evaporated, freeze-dried and stored under desiccation, at 4°C, and maintained for the inhibition studies (20).
3.3. Electrophoresis and Staining
To confirm the enzyme purity, sodium dodecyl sulfate polyacrylamide gel electrophoresis (SDS-PAGE), and Coomassie Blue staining were used.
3.4. Aldolase Reductase Activity Assay
The ALR2 activity was assayed, according to the method described by Hayman and Kinoshita (21). Briefly, bovine lens ALR2 activity was assayed spectrophotometrically, by measuring the decrease in the absorption of NADPH, at 340 nm, over a 5 minutes period, by using glyceraldehyde, as substrate. Various concentrations of CSE were added to 1 mL cuvettes, containing equal units of prepared bovine lens ALR2, 50 mM potassium phosphate buffer (pH 6.2, 0.4 mM lithium sulfate, 2-mercaptoethanol (5 mM)), with or without 10 mM of the DL-glyceraldehydes, as the substrate. Sorbinil, as a positive control of ALR2, was dissolved in dimethyl sulfoxide (DMSO), at 1 mg/mL, and various concentration of the preparations were added to the standard tubes, where the final concentration of DMSO was not greater than 1% (22). Appropriate blanks were employed in corrections. The assay mixture was incubated at 37°C and the reaction was initiated by the addition of 0.1 mM of NADPH, at 37°C. The change in the absorbance at 340 nm, due to NADPH oxidation, was followed on an Ultrospec 2100 pro UV/Visible spectrophotometer (GE Healthcare Life Sciences, Little Chalfont, United Kingdom) in quartz cuvettes.
3.5. Aldehyde Reductase Activity Assay
The activity of ALR1 was measured spectrophotometrically, by monitoring the oxidation of NADPH at 340 nm and 37°C, using glyceraldehyde, as substrate. The assayed mixture in 1 mL contained 50 mM sodium phosphate buffer (pH 7.2), 0.2 mM lithium sulfate, 5 mM 2-mercaptoethanol, 10 mM DL-glyceraldehyde, 0.1 mM NADPH, and various concentrations of C. spinosa (2).
3.6. Inhibition Studies
For inhibition studies, concentrated stocks of hydroalcoholic CSE from fruit were prepared in hydro alcoholic 70% solution and sorbinil was prepared in DMSO, as a positive control ALR2 inhibitor. Various concentrations of the hydroalcoholic CSE were added to the assay mixture (of ALR2 and ALR1) and incubated for 5 minutes, before initiating the reaction by NADPH, as described above. The percentage of inhibition with extracts was calculated and activity of ALR2, in the absence of inhibitor, was considered as 100%. The IC50 was determined via plotting concentration of CSE versus inhibition percentage. These stages were repeated three times, for each concentration.
3.7. Red Blood Cell Incubation and Sorbitol Estimation
Five mL of blood were collected from a healthy volunteer female, on overnight fasting, in tubes containing EDTA. The RBCs were separated by centrifugation, at 500 g, at 4°C, for 10 minutes, and washed three times, with 0.9% isotonic saline. To obtain washed erythrocytes, the upper layer of the mixture was removed. Then, 1 mL of washed RBCs were suspended in 4 mL of Krebs-ringer bicarbonate buffer, pH 7.4 (preequilibrated with 5% CO2) and incubated at 37°C and 5% CO2, for 3 hours, under normal (5 mM) and high glucose (55 mM) conditions. The effects of hydroalcoholic extract C. spinosa fruit, on sorbitol accumulation, were evaluated by incubating the RBCs in a high glucose (55 mM) environment at a concentration (0.54 mg/mL) equal to the IC50 of CSE, for ALR2 (2, 23, 24).
At the end of the incubation period, RBCs were homogenized with 1.0 mL of 0.475 M NaOH, 1.0 mL of 0.293 M ZnSO and then, the sorbitol content of the supernatant was assayed spectrofluorometry (Lumina, Thermo-Fischer Scientific, Waltham, MA, USA) (24).
3.8. Statistical Analysis
Statistical significance of the results was analyzed via Kruskal-Wallis test for unpaired data (observations). Data were presented as mean ± standard error of mean (SEM). All experiments were performed in triplicates and significance was accepted at P ≤ 0.05. The comparisons, between the percent inhibition of sorbitol accumulation, in the presence of sorbinil, as positive control and extract of C. spinosa were conducted based non-parametric Mann-Whitney tests, (P = 0.317). There was no significant difference between the extract and positive control, sorbinil.
4. Results
The SDS-PAGE and Coomassie Blue staining were carried out to check the enzyme partial purification, before and after gel filtration chromatography of ALR2 (Figure 1 Lane A and C) and, also, for bovine kidney cortex of ALR1 (Figure 1 Lane B). Samples were run on 12% SDS-PAGE and the gel was stained with Coomassie Blue. Molecular weight range of aldo-keto reductases (AKRs) supper family is reported at 35 - 40 kDa. Our results, presented in Figure 1, show that a clear band, relevant to ALR2 and ALR1, with an apparent molecular weight of about 39 kDa in SDS-PAGE, can be detected.
Enzyme Purity
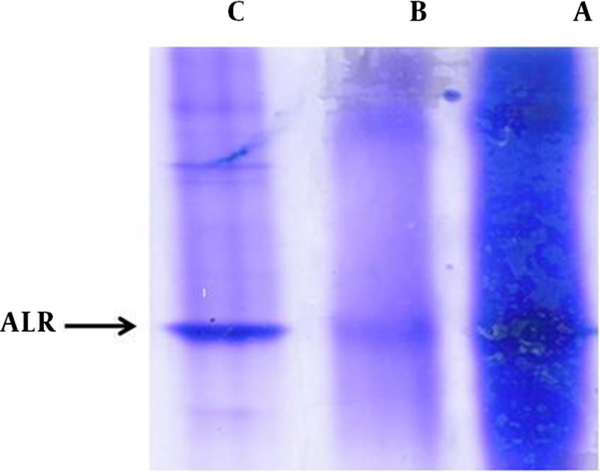
4.1. Inhibitory Effect of Capparis spinosa Extract on Aldose Reductase Activity
In the current study, through in vitro assaying, we demonstrated that CSE directly inhibits ALR2. The inhibitory activity of CSE against ALR2 was compared to the positive control, sorbinil. As shown in Figure 2, hydroalcoholic extracts of C. spinosa fruit inhibited partial purified ALR2, from bovine lens, with an IC50 value of 0.54 mg/mL.
Inhibition of Aldose Reductase by Hydroalcoholic of C. spinosa Extract
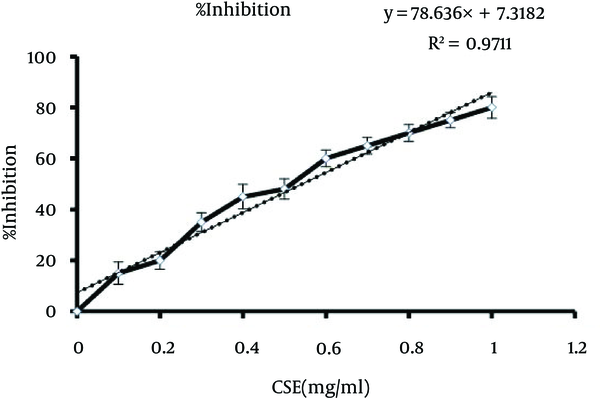
4.2. Inhibitory Effect of Capparis spinosa Extract on Aldehyde Reductase Activity
Both ALR2 and ALR1 are belonging to the AKR family and they show high similarities, in catalyzing aldehydes in an NDPH-dependent reaction. Therefore, it is important to examine CSE specificity for ALR1, as well. To do that, the inhibitory effect of CSE on ALR1 was determined, by using the partially purified ALR1, from bovine kidney. The CSE inhibited ALR1, although its IC50 value was seven and a half times greater than that of ALR2, as shown in Table 1.
4.3. Reduction of Sorbitol Accumulation in Erythrocytes
To check the inhibitory effect of CSE on sorbitol accumulation related to ALR2 activity, freshly prepared RBCs were incubated with glucose, under normal (5 mM) and high glucose (55 mM) condition. Incubation of RBCs with 55 mM glucose resulted in a 34.8% increase of sorbitol accumulation, compared with control (P = 0.05) and was significant, Figure 3 A. If the sorbitol accumulation in the control group is to be considered to 100%, this would translate into a 46.8% reduction of sorbitol accumulation, when the RBCs were treated with 0.54 mg/mL of C. spinosa fruit extract, Figure 3 B.
The Inhibitory Effect of Capparis spinosa Extract on Sorbitol Accumulation in Relation to Aldose Reductase, Under Normal and High Glucose Conditions, in Comparison to the Control.
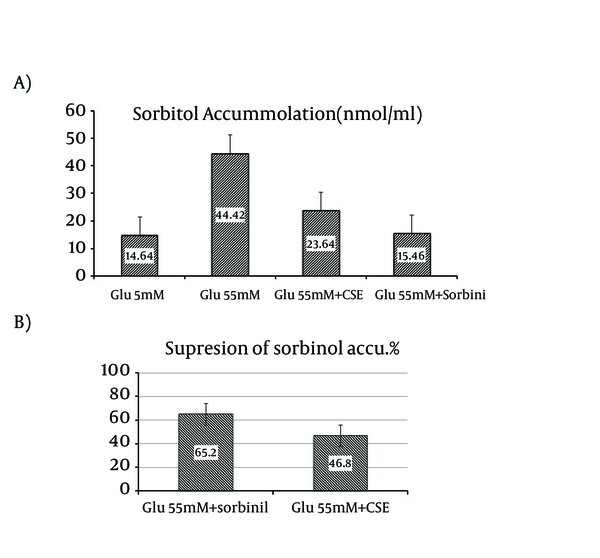
5. Discussion
In the present study, we have selected C. spinosa for its anti-diabetic importance documented in traditional medicine. The plant samples extracted in hydroalcoholic solvent were further evaluated for the ALR2 inhibition.
Multiple studies have shown a strong relationship between the increased ALR2 activity and risk of secondary complications of diabetes (25). Although a wide variety of synthetic compounds have been found to inhibit ALR2 and were studied in experimental models, only several drugs have reached to clinical trial stage. Two chemical classes of ALR2 inhibitors have been tested in phase III trials. Carboxylic acidic inhibitors, such as zopolrestat, ponalrestat, and tolerestat have poor tissue penetration and do not exert very potent in vivo effects. Spiroimide inhibitors, such as sorbinil, penetrate tissues more efficiently, while unfortunately generating allergic skin reaction and kidney toxicity (26-28).
Several studies of the in vitro and, also, experimental animal models (in vivo) have shown that compounds that inhibit ALR2 could be effective in preventing and treatment the complications of diabetes (22). Therefore, one of the mechanisms for the design of antidiabetic drugs is to select plants or compounds that inhibit the ALR2 enzyme.
In phytotherapy, the inhibitory effect of fruit CSE on ALR2 has never been demonstrated, experimentally. Therefore, we investigated fruit CSE for its inhibitory potential against bovine lens ALR2. In the present study, we selected C. spinosa for having antidiabetic properties in traditional medicine. The plant sample was extracted in hydroalcoholic solvent and then evaluated for the ALR2 inhibition.
Clinical studies confirmed that the diabetic complications were prevented or slowed down by natural therapies, particularly plants that contain a large amount of flavonoids. These flavonoids have multiple antioxidant and ALR2 inhibitory properties (10).
Several studies have surveyed the inhibitory effects the purified compounds from plants on the ALR2 enzyme. The IC50 of the pure compounds for enzyme was very small (averaging several µM), which indicates a strong inhibitory effect on the enzyme. On the contrary, plant extracts have a high value of IC50, because they have effective components along with impurities. Therefore, it is not reasonable to compare the IC50 of pure compounds, with impure extract. Nevertheless, if the active ingredient of CSE inhibits the enzyme, its pure form can be used for designing drugs for the prevention and treatment of diabetes complications, which do not have the side effects of synthetic compounds.
As a consequence, there is a need for developing and evaluating novel ARIs, considering efficacy, selectivity and safety issues. In the present study, we have evaluated the ARI potential of the CSE. Furthermore, the specificity of CSE towards ALR2 was evaluated, using ALR1. It has been reported that the mammalian kidney is rich in ALR1, while low in ALR2 (29). Further, we investigated the specificity toward ALR2, as compared with other members of the AKR family. The primary structure of ALR2 has high similarities with ALR1. Therefore, we studied the specificity of CSE, with the related AKR member, ALR1. The CSE inhibited ALR1 and the IC50 value was seven and a half times higher than for ALR2 (4.05 ± 2 mg/mL), indicating its relative selectivity for ALR2. The relatively poor selectivity for ALR2, versus ALR1, might be one of the reasons for not being successful in clinical trials.
Activation of ALR2 in RBC, leads to the accumulation of sorbitol at the level of the lens, retina, nerve and kidney (30). There is a direct correlation between ALR2 activity and sorbitol levels in erythrocyte. Therefore, we measured the accumulation of sorbitol in RBC, under high glucose conditions. Also, the decrease in the accumulation of intracellular sorbitol in RBC in ex vivo condition, by CSE substantiated the in vivo efficacy. Incubation of RBC with 55 mM glucose resulted in the accumulation of sorbitol to 34.8% higher levels than the control, while in the presence of CSE, intracellular sorbitol reduced significantly. These results substantiate the inhibition of ALR2, indicating the significance of C. spinosa fruit extract in preventing the accumulation of intracellular sorbitol.
In conclusion, for the first time, we demonstrate experimentally that CSE is able to inhibit ALR2 from bovine lens, in vitro setting. This inhibition seems to be more specific for ALR2 compared to ALR1. Suppression of intracellular sorbitol accumulation in erythrocytes suggests that CSE could prevent or delay diabetic complications, such as cataract, through the ALR2 inhibition. Also, these findings provide the directions for searching the recognition, separating and purifying effective compounds of CSE that can be considered for designing and development of new drugs, for the prevention and treatment of diabetic complications. Therefore, supplementary in vivo studies (for example in animal models) are required to complete these findings.
Acknowledgements
References
-
1.
King H, Aubert RE, Herman WH. Global burden of diabetes, 1995-2025: prevalence, numerical estimates, and projections. Diabetes Care. 1998;21(9):1414-31. [PubMed ID: 9727886].
-
2.
Saraswat M, Muthenna P, Suryanarayana P, Petrash JM, Reddy GB. Dietary sources of aldose reductase inhibitors: prospects for alleviating diabetic complications. Asia Pac J Clin Nutr. 2008;17(4):558-65. [PubMed ID: 19114390].
-
3.
Brownlee M. Biochemistry and molecular cell biology of diabetic complications. Nature. 2001;414(6865):813-20. [PubMed ID: 11742414]. https://doi.org/10.1038/414813a.
-
4.
Lorenzi M. The polyol pathway as a mechanism for diabetic retinopathy: attractive, elusive, and resilient. Exp Diabetes Res. 2007;2007:61038. [PubMed ID: 18224243]. https://doi.org/10.1155/2007/61038.
-
5.
Tomlinson DR, Willars GB, Carrington AL. Aldose reductase inhibitors and diabetic complications. Pharmacol Therapeut. 1992;54(2):151-94. https://doi.org/10.1016/0163-7258(92)90031-t.
-
6.
Bhatnagar A, Srivastava SK. Aldose reductase: congenial and injurious profiles of an enigmatic enzyme. Biochem Med Metab Biol. 1992;48(2):91-121. [PubMed ID: 1419150].
-
7.
Srivastava SK, Petrash JM, Sadana IJ, Ansari NH, Partridge CA. Susceptibility of aldehyde and aldose reductases of human tissues to aldose reductase inhibitors. Curr Eye Res. 1982;2(6):407-10. [PubMed ID: 6820339].
-
8.
Spite M, Baba SP, Ahmed Y, Barski OA, Nijhawan K, Petrash JM, et al. Substrate specificity and catalytic efficiency of aldo-keto reductases with phospholipid aldehydes. Biochem J. 2007;405(1):95-105. [PubMed ID: 17381426]. https://doi.org/10.1042/BJ20061743.
-
9.
Hyndman DJ, Flynn TG. Sequence and expression levels in human tissues of a new member of the aldo-keto reductase family. Biochim Biophys Acta. 1998;1399(2-3):198-202. [PubMed ID: 9765596].
-
10.
Lim SS, Jung SH, Ji J, Shin KH, Keum SR. Synthesis of flavonoids and their effects on aldose reductase and sorbitol accumulation in streptozotocin-induced diabetic rat tissues. J Pharm Pharmacol. 2001;53(5):653-68. [PubMed ID: 11370705].
-
11.
Sultan AO, Çelik TA. Genotoxic and Antimutagenic Effects of Capparis spinosaL. on theAllium cepaL. Root Tip Meristem Cells. Caryologia. 2009;62(2):114-23. https://doi.org/10.1080/00087114.2004.10589676.
-
12.
Rahmani R, Mahmoodi M, Karimi M, Hoseini F, Heydari R, Salehi M. Effect of Hydroalcoholic Extract of Capparis Spinosa Fruit on Blood Sugar and Lipid Profile of Diabetic and normal Rats. Zahedan J Res Med Sci. 2013;15(11):34-8.
-
13.
Eddouks M, Lemhadri A, Michel JB. Hypolipidemic activity of aqueous extract of Capparis spinosa L. in normal and diabetic rats. J Ethnopharmacol. 2005;98(3):345-50. [PubMed ID: 15814271]. https://doi.org/10.1016/j.jep.2005.01.053.
-
14.
Eddouks M, Lemhadri A, Michel JB. Caraway and caper: potential anti-hyperglycaemic plants in diabetic rats. J Ethnopharmacol. 2004;94(1):143-8. [PubMed ID: 15261975]. https://doi.org/10.1016/j.jep.2004.05.006.
-
15.
Brevard H, Brambilla M, Chaintreau A, Marion J-P, Diserens H. Occurrence of elemental sulphur in capers (Capparis spinosa L.) and first investigation of the flavour profile. Flavour Fragrance J. 1992;7(6):313-21. https://doi.org/10.1002/ffj.2730070605.
-
16.
Sharaf M, el-Ansari MA, Saleh NA. Quercetin triglycoside from Capparis spinosa. Fitoterapia. 2000;71(1):46-9. [PubMed ID: 11449469].
-
17.
Sharaf M, El-Ansari MA, Saleh NAM. Flavonoids of four Cleome and three Capparis species. Biochem Systemat Ecol. 1997;25(2):161-6. https://doi.org/10.1016/s0305-1978(96)00099-3.
-
18.
Halder AB, Crabbe MJ. Bovine lens aldehyde reductase (aldose reductase). Purification, kinetics and mechanism. Biochem J. 1984;219(1):33-9. [PubMed ID: 6426471].
-
19.
Daly AK, Mantle TJ. Purification and characterization of the multiple forms of aldehyde reductase in ox kidney. Biochem J. 1982;205(2):373-80. [PubMed ID: 6753832].
-
20.
Aghel N, Rashidi I, Mombeini A. Hepatoprotective activity of Capparis spinosa root bark against CCl4 induced hepatic damage in mice. Iranian J Pharmaceut Res. 2010;6(4):285-90.
-
21.
Hayman S, Kinoshita JH. Isolation and Properties of Lens Aldose Reductase. J Biol Chem. 1965;240:877-82. [PubMed ID: 14275148].
-
22.
Puppala M, Ponder J, Suryanarayana P, Reddy GB, Petrash JM, LaBarbera DV. The isolation and characterization of beta-glucogallin as a novel aldose reductase inhibitor from Emblica officinalis. PLoS One. 2012;7(4). eee31399. [PubMed ID: 22485126]. https://doi.org/10.1371/journal.pone.0031399.
-
23.
Reddy GB, Muthenna P, Akileshwari C, Saraswat M, Petrash JM. Inhibition of aldose reductase and sorbitol accumulation by dietary rutin. Current Science. 2011;101(9):1191-7.
-
24.
Shinohara R, Ohta Y, Yamauchi M, Ishiguro I. Improved fluorometric enzymatic sorbitol assay in human blood. Clin Chim Acta. 1998;273(2):171-84. [PubMed ID: 9657347].
-
25.
Halder N, Joshi S, Gupta SK. Lens aldose reductase inhibiting potential of some indigenous plants. J Ethnopharmacol. 2003;86(1):113-6. [PubMed ID: 12686449].
-
26.
Mylari BL, Armento SJ, Beebe DA, Conn EL, Coutcher JB, Dina MS, et al. A novel series of non-carboxylic acid, non-hydantoin inhibitors of aldose reductase with potent oral activity in diabetic rat models: 6-(5-chloro-3-methylbenzofuran-2-sulfonyl)-2H-pyridazin-3-one and congeners. J Med Chem. 2005;48(20):6326-39. [PubMed ID: 16190759]. https://doi.org/10.1021/jm050462t.
-
27.
A randomized trial of sorbinil, an aldose reductase inhibitor, in diabetic retinopathy. Sorbinil Retinopathy Trial Research Group. Arch Ophthalmol. 1990;108(9):1234-44. [PubMed ID: 2119168].
-
28.
Dagher Z, Park YS, Asnaghi V, Hoehn T, Gerhardinger C, Lorenzi M. Studies of rat and human retinas predict a role for the polyol pathway in human diabetic retinopathy. Diabetes. 2004;53(9):2404-11. [PubMed ID: 15331552].
-
29.
Terubayashi H, Sato S, Nishimura C, Kador PF, Kinoshita JH. Localization of aldose and aldehyde reductase in the kidney. Kidney Int. 1989;36(5):843-51. [PubMed ID: 2515341].
-
30.
Morrison AD, Clements Jr RS, Winegrad AI. Effects of elevated glucose concentrations on the metabolism of the aortic wall. J Clin Invest. 1972;51(12):3114-23. [PubMed ID: 4640951]. https://doi.org/10.1172/JCI107138.