Abstract
Background:
Fluoxetine (FLX), a selective serotonin reuptake inhibitor, is widely used in the treatment of neurological disorders. However, its application carries the risk of adverse reactions, including reproductive toxicities. Grape seed is highly regarded for its pharmacological activities, specifically its antioxidant properties.Objectives:
This study was designed to evaluate whether hydroalcoholic grape seed extract (GSE) could ameliorate FLX-evoked oxidative injuries in the mouse testis.Materials and Methods:
Thirty-two male mice were categorized into four groups of eight mice each. Two groups received FLX at a dose of 20 mg/kg per day over a period of six weeks by oral gavage. One of these groups received GSE at a dose of 100 mg/kg per day orally along with the FLX. An untreated control group and a GSE-only treated group were also included. Serum and testicular samples were collected 24 hours after the last treatment.Results:
Biochemical analyses demonstrated that FLX caused significant decreases in testicular antioxidant capacity and serum testosterone concentrations, as well as lipid peroxidation elevations in testicular tissue. Morphometric and histopathological studies supported the biochemical findings. Concomitant administration of GSE with FLX markedly reduced testicular damage and significantly restored all of the mentioned markers to normal values.Conclusions:
The findings suggested that oxidative stress-mediated testicular damage, arising as a result of FLX administration in mice, could be reversed by GSE.Keywords
1. Background
Depression affects approximately 350 million people worldwide, and fluoxetine (FLX), a selective serotonin reuptake inhibitor (SSRI), is the most commonly used antidepressant (1). Unfortunately, its application is accompanied by undesirable side effects, such as pulmonary, cardiovascular, hepatic, endocrine, and reproductive disorders (2-5). Laboratory evidence indicates that FLX treatment is associated with reduced male fertility and testicular hypocellularity (6, 7). It has also been found that FLX causes marked decreases in reproductive organ weights and testosterone levels (6).
Recently, efforts have been geared toward searching for more potent antioxidant agents, especially of natural origin, to counteract oxidative stress (OS)-mediated reproductive toxicity arising from drug administration. It is believed that OS development negatively impacts fertilization rates and pregnancy outcomes through the sperm membrane and DNA damage, and the prevention of OS is vital for the preservation of normal reproductive function (8). Furthermore, at the testis level, OS is involved in steroidogenesis impairment and germinal epithelium differentiation disruption (9, 10). Growing evidence demonstrates that the black grape (Vitis vinifera) and its seed possess a broad spectrum of pharmacological properties, including free-radical scavenging (11), antitumor (12), antifibrotic (13), anti-apoptotic (14), and anti-inflammatory activities (15). Moreover, recent studies have revealed that grape seed extract (GSE) has a potential reproprotective effect in male reproductive disorders (16, 17).
2. Objectives
We investigated the efficacy of GSE in terms of preventing testicular tissue injury induced by FLX in mice.
3. Materials and Methods
3.1. Extract Preparation
The seeds were detached from black grape pulp, dried in the shade at room temperature, and ground into a powder. The powdered grape seeds were mixed with aqueous ethanol (70%, v/v) at a ratio of 1:10 (w/v) for 72 hours at room temperature. The filtrate extract was evaporated by a rotary evaporator under vacuum at 40°C. The average (w/w) yield was 12% (14).
3.2. Animals
Thirty-two healthy adult male mice, weighing 30 ± 2 g, were provided by the breeding unit of the veterinary medicine faculty of Urmia university. The animals were housed in the standard animal facility (22 ± 2°C constant temperature, 50 ± 10% relative humidity, 12-hour lighting cycle), with free access to tap water and pelleted commercial laboratory animal chow during the entire course of the experiment. All experimental procedures were conducted in conformity with the criteria outlined in the guide for the care and use of laboratory animals, and were approved by the board of the faculty of veterinary medicine at Urmia university.
3.3. Experimental Protocol
After an acclimatization period of 14 days, the animals were divided into four groups: the control group (n = 8); the FLX-treated group (n = 8), which received a single daily oral dose of FLX 20 mg/kg/day for 42 days; the GSE-treated group (n = 8), which received a single daily oral dose of GSE 100 mg/kg/day for 42 days; and the FLX/GSE-treated group (n = 8), which received both FLX and GSE at the previously indicated dosages and times. The dosages were selected according to previously published studies (7, 14).
3.4. Sample Preparation
Twenty-four hours after the last treatment, the mice were sacrificed by cervical dislocation following anesthesia with ketamine (75 mg/kg IP). Blood samples were collected from the atrium and sera were separated and kept at -70°C until the determination of testosterone. Both testes were rapidly excised and weighed following adhesive connective tissue removal. One half of the right and left testes were fixed in 10% formalin for histopathological and morphometric evaluations, and the other halves were snap-frozen in liquid nitrogen and kept at -70°C until being homogenized for further biochemical analyses.
3.5. Determination of Serum Testosterone Concentration
Serum testosterone levels were assayed using ELISA kits (Monobind Inc., USA) according to the manufacturer’s instructions.
3.6. Biochemical Analysis of Testicular Tissue Homogenate
Assessments of the testicular antioxidant defense mechanisms were performed for the tissue homogenate by evaluating the lipid peroxide content and total antioxidant capacity (TAC). malondialdehyde (MDA), as an end-product of lipid peroxidation, was determined in the testicular homogenate based on its reaction with thiobarbituric acid to form a pink complex with a maximum absorption at 535 nm. The results were expressed as equivalents of MDA in testicular tissue homogenate in μmol/g tissue (18). TAC was measured based on assessment with the ferric reduction antioxidant power assay as previously described (19), and expressed as μmol/g of tissue samples.
3.7. Histopathological Studies
The testes were fixed in 10% formalin, dehydrated in ethyl alcohol, cleared in xylene, and embedded in paraffin wax. Five-micrometer-thick sections were cut, mounted on slides, and stained with hematoxylin and eosin for histopathological and morphometric assessments.
3.8. Morphometric Measurements
Five vertical sections from the polar and the equatorial regions were sampled for each testis, and a systematic random scheme was used to analyze the following morphometric parameters (20):
1, Germinal epithelium height (GEH) and seminiferous tubule diameter (STD): To measure GEH and STD, 200 round or nearly-round cross-sections of the seminiferous tubules were randomly chosen from each mouse. Using an ocular micrometer with light microscopy, the GEH at 4 equidistant points of each seminiferous tubule cross-section was measured, and the mean was recorded. The STDs were measured across the minor and major axes, and their means were obtained (21).
2, Tubule differentiation index (TDI): 200 cross-sections of seminiferous tubules were randomly analyzed in each mouse for the calculation of TDI, which is the percentage of seminiferous tubules containing at least three differentiated germ cells (20).
3, Sertoli cell index (SCI) and repopulation index (RI): Sixty seminiferous tubules per group were randomly examined for the calculation of SCI and RI. The ratio of the number of germ cells to the number of Sertoli cells, and the percentage of tubules populated with germ cells that had clearly reached the intermediate spermatogonial stage or later, were recorded to determine SCI and RI, respectively (19).
4, Mononuclear immune cells (MNICs) in testicular tissue: The number of MNICs was determined using the unbiased counting frame proposed by Gundersen (22).
3.9. Statistical Analyses
The results were represented as mean ± SD. The variables were analyzed with one-way analysis of variance followed by the Tukey test for post hoc comparisons, using SPSS version 18.0 (SPSS Inc., Chicago, IL, USA). The differences were considered significant when the calculated P value was < 0.05.
4. Results
4.1. Body and Testis Weight Changes
FLX treatment significantly (P < 0.05) decreased the mouse body and testis weights at the time of sacrifice compared to the normal control group. Concomitant treatment with GSE significantly abolished these decreases (P < 0.05). A significant increase in final body weight was recorded in the GSE-only treated animals in comparison with the controls (Table 1).
Effect of Fluoxetine and Grape Seed Extract on Body and Testis Weights, Testosterone Levels, and Testicular Oxidative Stress Markers (n = 8)a,b
Control | GSE | FLX | FLX + GSE | |
---|---|---|---|---|
Final Body Weight, g | 34.22 ± 0.24 | 38.05 ± 0.14 | 23.84 ± 1.60 | 33.62 ± 0.10 |
Testis Weight, g | 0.114 ± 0.0014 | 0.118 ± 0.0014 | 0.94 ± 0.0020 | 0.115 ± 0.0007 |
Testosterone, ng/mL | 7.59 ± 0.077 | 7.32 ± 0.042 | 4.11 ± 0.040 | 5.27 ± 0.035 |
MDA, μmol/g Tissue | 220.04 ± 24.02 | 213.96 ± 20.87 | 477.86 ± 11.43 | 366.36 ± 28.23 |
TAC, mmol/g Tissue | 1.31 ± 0.014 | 1.30 ± 0.014 | 0.73 ± 0.140 | 1.08 ± 0.007 |
4.2. Serum Testosterone Levels
FLX administration resulted in markedly decreased (P < 0.05) serum testosterone levels compared to the control mice. Treatment with GSE in combination with FLX significantly (P < 0.05) restored the FLX-induced decreases in serum testosterone levels, indicating the protective role of GSE on testicular androgenic disorders resulting from FLX exposure (Table 1).
4.3. Testicular Oxidative Stress Markers
In the current study, oral administration of FLX significantly (P < 0.05) increased the testicular MDA content parallel to reduced testicular TAC levels, compared to the control animals. Co-administration of GSE restored both testicular MDA and TAC to levels that were statistically significantly different (P < 0.05) from those of the FLX-alone group (Table 1).
4.4. Testicular Histopathology
Examination of the testicular sections revealed that both the control and the GSE-only mice testes had normal histoarchitecture, featuring seminiferous tubules lined with a thick layer of uniformly arranged spermatogenic cells at different stages of maturation (Figure 1A and B, respectively). In contrast, the FLX-treated mice showed drastic morphologic changes in the normal testicular architecture, as evidenced by spermatogenic cell degeneration, disrupted Sertoli cells, intraepithelial vacuolization, tubular lumens filling with fibrinoid debris from shredded cells, and widened interstitial spaces with inflammatory cell infiltration and edematous fluid accumulation (Figure 1C). Remarkably, simultaneous administration of GSE in the FLX-treated mice greatly improved the FLX-induced lesions in the testicular tissues, although detachment of germ cells from the basement membrane was observed in few seminiferous tubules (Figure 1D).
Effect of Fluoxetine and Grape Seed Extract on Testicular Histopathology
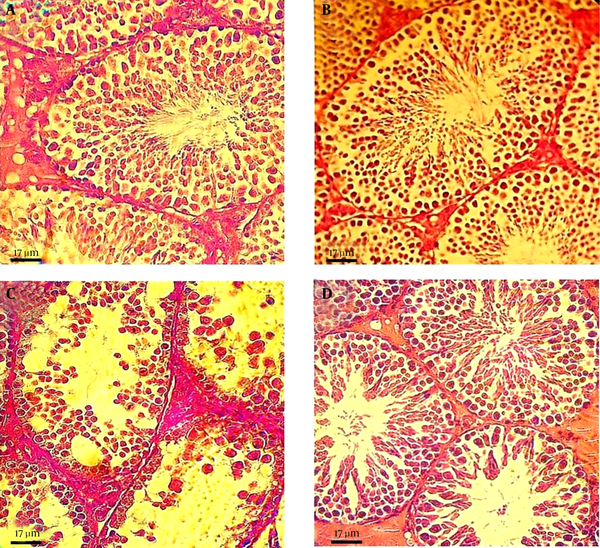
4.5. Histomorphometric Studies
In parallel to the observed histopathological alterations, FLX significantly (P < 0.05) reduced GEH, TDI, RI, and SCI and increased MNIC infiltration in testicular tissues. Notably, administration of GSE along with FLX significantly (P < 0.05) restored these morphometric changes toward normalcy (Table 2).
Effect of Fluoxetine and Grape Seed Extract on Testicular Morphometric Parameters (n = 8)a,b
Control | GSE | FLX | FLX + GSE | |
---|---|---|---|---|
STD, μm | 200.40 ± 1.40 | 195.78 ± 2.07 | 187.62 ± 0.82 | 193.45 ± 1.48 |
GEH, μm | 79.27 ± 0.35 | 79.63 ± 0.51 | 56.14 ± 0.50 | 79.80 ± 0.54 |
TDI, % | 89.21 ± 1.18 | 88.81 ± 1.03 | 47.51 ± 1.45 | 73.25 ± 0.35 |
RI, % | 91.24 ± 1.02 | 88.35 ±0.49 | 65.65 ± 0.35 | 74.87 ± 0.60 |
SCI, % | 24.64 ± 0.50 | 24.01 ± 0.41 | 12.72 ± 0.24 | 21.51 ± 0.40 |
MNIC Number, mm2 | 5.16 ± 0.16 | 5.00 ± 0.14 | 14.20 ± 0.14 | 10.65 ± 0.21 |
5. Discussion
Epidemiological studies indicate that nearly 6% of people aged 18 years and older have had a period of depression during the previous year (1). Unfortunately, the use of FLX, a well-known and widely prescribed antidepressant drug, is accompanied by undesirable side effects, including reproductive dysfunction (5-7). In agreement with previous reports, the current work demonstrated that FLX administration reduces body and testis weight, and has injurious effects on the testicular tissue (23, 24).
It has been shown that SSRI drugs have inhibitory actions on alimentary ingestion and that they reduce intestinal villus height (25, 26). Thus, the marked reduction in body weight that occurs with FLX can be explained by decreased intestinal absorption, leading to the diminishment of necessary nutrients. On the other hand, it is known that testicular weight largely depends on the population of differentiated spermatogenic cells (27). Therefore, FLX-induced significant testicular weight loss can be attributed to testicular hypocellularity and spermatogenesis suppression, as well as to the reduced availability of androgens, as confirmed by our findings.
In the present study, a significant decline in serum testosterone concentrations was recorded in the FLX-treated mice, confirming previous reports that SSRIs are anti-androgenic agents that induce markedly reduced serum testosterone levels through interference with the endocrine system (28).
Spermatogenesis is a highly complicated, regular cytodifferentiation process, and Sertoli cells are specialized somatic cells that interact directly with developing germ cells (GCs) throughout this process (29). Moreover, it is well-documented that the optimum level of testosterone is necessary for ordinary spermatogenesis and GC structural development, as well as the normal histophysiology of the seminiferous tubules (30). In support of these facts, it seems that FLX-evoked disruption of testosterone-dependent GC maturation events can result in defective spermatogenesis, leading to considerable alterations in testicular histopathology and histomorphometric parameters.
It has been established that male GCs are highly predisposed to OS-related toxicity due to the unique structure of their plasma membranes, which is rich in polyunsaturated fatty acids (31). Consistent with the above findings, the current study confirmed that FLX administration is associated with elevations in testicular lipid peroxidation, parallel to decreased levels of TAC. Furthermore, such toxic biochemical stresses were reflected microscopically, which showed that FLX dramatically depleted the testicular germinal epithelium, in agreement with previous studies in laboratory animals (23, 24).
The current study demonstrated that GSE provides promising protection against FLX-induced gonadotoxicity via reversing OS, suggesting that GSE as an adjuvant therapy can partially antagonize the deleterious effects of FLX on testicular tissue. Recently, more attention has been paid to the beneficial effects of antioxidants and naturally occurring substances against drug-induced toxicity, especially when free-radical overproduction is involved. GSE, as a powerful free-radical scavenger, has recently received much attention due to its broad spectrum of pharmacological properties against OS (32). Many of the reported therapeutic effects of GSE reside in its high content of flavonoids and phenolic acids (14). Recently, a substantial body of growing evidence has suggested that GSE inhibits oxidative injury through modulation of antioxidant enzyme system expression and the suppression of nitrosative/oxidative stress (33, 34). Moreover, it has been shown that GSE increases intracellular vitamin C levels (35), blocks cell-death signaling (36), and modulates oxidative DNA damage (11). Consistent with our findings, it has been found that GSE has a protective effect against OS-induced testicular damage in cisplatin-treated rabbits and rats (17, 37). It has also been reported that GSE, as a potent antioxidant, can help to prevent OS-related testicular injury and dysfunction following experimental testicular torsion-detorsion and arsenic treatments in rats and mice, respectively (17, 38).
In conclusion, this study provided evidence that GSE could protect the mouse testis from oxidative injury related to FLX through oxidant-antioxidant system management. Verification of GSE’s therapeutic efficacy in human subjects merits further investigation.
Acknowledgements
References
-
1.
Pilania M, Bairwa M, Kumar N, Khanna P, Kurana H. Elderly depression in India: An emerging public health challenge. Australas Med J. 2013;6(3):107-11. [PubMed ID: 23589734]. https://doi.org/10.4066/AMJ.2013.1583.
-
2.
de Kerviler E, Tredaniel J, Revlon G, Groussard O, Zalcman G, Ortoli JM, et al. Fluoxetin-induced pulmonary granulomatosis. Eur Respir J. 1996;9(3):615-7. [PubMed ID: 8730028].
-
3.
Graudins A, Vossler C, Wang R. Fluoxetine-induced cardiotoxicity with response to bicarbonate therapy. Am J Emerg Med. 1997;15(5):501-3. [PubMed ID: 9270390].
-
4.
Inkielewicz-Stepniak I. Impact of fluoxetine on liver damage in rats. Pharmacol Rep. 2011;63(2):441-7. [PubMed ID: 21602599].
-
5.
Muller JC, Imazaki PH, Boareto AC, Lourenco EL, Golin M, Vechi MF, et al. In vivo and in vitro estrogenic activity of the antidepressant fluoxetine. Reprod Toxicol. 2012;34(1):80-5. [PubMed ID: 22522098]. https://doi.org/10.1016/j.reprotox.2012.04.001.
-
6.
Bataineh HN, Daradka T. Effects of long-term use of fluoxetine on fertility parameters in adult male rats. Neuro Endocrinol Lett. 2007;28(3):321-5. [PubMed ID: 17627270].
-
7.
Silva J, Lins A, Amorim J, Pinto CF, Deiró TBJ, Oliveira JRM, et al. Neonatal administration of fluoxetine decreased final sertoli cell number in Wistar rats. Int. J. Morphol. 2008;26(1):51-62.
-
8.
Agarwal A, Durairajanayagam D, du Plessis SS. Utility of antioxidants during assisted reproductive techniques: an evidence based review. Reprod Biol Endocrinol. 2014;12:112. [PubMed ID: 25421286]. https://doi.org/10.1186/1477-7827-12-112.
-
9.
Hales DB, Allen JA, Shankara T, Janus P, Buck S, Diemer T, et al. Mitochondrial function in Leydig cell steroidogenesis. Ann N Y Acad Sci. 2005;1061:120-34. [PubMed ID: 16469751]. https://doi.org/10.1196/annals.1336.014.
-
10.
Aitken RJ, Roman SD. Antioxidant systems and oxidative stress in the testes. Oxid Med Cell Longev. 2008;1(1):15-24. [PubMed ID: 19794904].
-
11.
Balu M, Sangeetha P, Murali G, Panneerselvam C. Modulatory role of grape seed extract on age-related oxidative DNA damage in central nervous system of rats. Brain Res Bull. 2006;68(6):469-73. [PubMed ID: 16459205]. https://doi.org/10.1016/j.brainresbull.2005.10.007.
-
12.
Singh RP, Tyagi AK, Dhanalakshmi S, Agarwal R, Agarwal C. Grape seed extract inhibits advanced human prostate tumor growth and angiogenesis and upregulates insulin-like growth factor binding protein-3. Int J Cancer. 2004;108(5):733-40. [PubMed ID: 14696100]. https://doi.org/10.1002/ijc.11620.
-
13.
Hemmati AA, Nazari Z, Samei M. A comparative study of grape seed extract and vitamin E effects on silica-induced pulmonary fibrosis in rats. Pulm Pharmacol Ther. 2008;21(4):668-74. [PubMed ID: 18547852]. https://doi.org/10.1016/j.pupt.2008.04.004.
-
14.
Yonguc GN, Dodurga Y, Adiguzel E, Gundogdu G, Kucukatay V, Ozbal S, et al. Grape seed extract has superior beneficial effects than vitamin E on oxidative stress and apoptosis in the hippocampus of streptozotocin induced diabetic rats. Gene. 2015;555(2):119-26. [PubMed ID: 25445279]. https://doi.org/10.1016/j.gene.2014.10.052.
-
15.
Park JS, Park MK, Oh HJ, Woo YJ, Lim MA, Lee JH, et al. Grape-seed proanthocyanidin extract as suppressors of bone destruction in inflammatory autoimmune arthritis. PLoS One. 2012;7(12). eeee51377. [PubMed ID: 23251512]. https://doi.org/10.1371/journal.pone.0051377.
-
16.
Bayatli F, Akkus D, Kilic E, Saraymen R, Sonmez MF. The protective effects of grape seed extract on MDA, AOPP, apoptosis and eNOS expression in testicular torsion: an experimental study. World J Urol. 2013;31(3):615-22. [PubMed ID: 23475212]. https://doi.org/10.1007/s00345-013-1049-8.
-
17.
Zhao YM, Gao LP, Zhang HL, Guo JX, Guo PP. Grape seed proanthocyanidin extract prevents DDP-induced testicular toxicity in rats. Food Funct. 2014;5(3):605-11. [PubMed ID: 24504493]. https://doi.org/10.1039/c3fo60486a.
-
18.
Najafi G, Nejati V, Shalizar Jalali A, Zahmatkesh E. Protective role of royal jelly in oxymetholone-induced oxidative injury in mouse testis. Iran J Toxicol. 2014;8(25):1073-80.
-
19.
Jalali AS, Hasanzadeh S, Malekinejad H. Achillea millefolium inflorescence aqueous extract ameliorates cyclophosphamide-induced toxicity in rat testis: stereological evidences. Chin J Nat Med. 2012;10(4):247-54.
-
20.
Shalizar Jalali A, Hasanzadeh S. Crataegus monogyna fruit aqueous extract as a protective agent against doxorubicin-induced reproductive toxicity in male rats. Avicenna J Phytomed. 2013;3(2):159-70. [PubMed ID: 25050270].
-
21.
Jalali AS, Hasanzadeh S, Malekinejad H. Crataegus monogyna aqueous extract ameliorates cyclophosphamide-induced toxicity in rat testis: stereological evidences. Acta Med Iran. 2012;50(1):1-8. [PubMed ID: 22267371].
-
22.
Gundersen HJG. Notes on the estimation of the numerical density of arbitrary profiles: the edge effect. J microsc. 1977;111(2):219-23.
-
23.
Sakr SA, Mahran HA, El-Deeb MM. Ameliorative effect of curcumin on fluoxetine-induced reproductive toxicity and oxidative stress in male albino rats. Oxid Antioxid Med Sci. 2013;2(1):29-35.
-
24.
Aggarwal A, Jethani SL, Rohatgi RK, Kalra J. Effects of fluoxetine on testis of albino rats–a histological assessment. Int J Sci Eng Res. 2012;3(7):1-5.
-
25.
Simansky KJ. Serotonergic control of the organization of feeding and satiety. Behav Brain Res. 1996;73(1-2):37-42. [PubMed ID: 8788474].
-
26.
Morrison JL, Riggs KW, Rurak DW. Fluoxetine during pregnancy: impact on fetal development. Reprod Fertil Dev. 2005;17(6):641-50. [PubMed ID: 16263070].
-
27.
Katoh C, Kitajima S, Saga Y, Kanno J, Horii I, Inoue T. Assessment of quantitative dual-parameter flow cytometric analysis for the evaluation of testicular toxicity using cyclophosphamide- and ethinylestradiol-treated rats. J Toxicol Sci. 2002;27(2):87-96. [PubMed ID: 12058451].
-
28.
Safarinejad MR. Evaluation of endocrine profile and hypothalamic-pituitary-testis axis in selective serotonin reuptake inhibitor-induced male sexual dysfunction. J Clin Psychopharmacol. 2008;28(4):418-23. [PubMed ID: 18626269]. https://doi.org/10.1097/JCP.0b013e31817e6f80.
-
29.
Mruk DD, Cheng CY. Sertoli-Sertoli and Sertoli-germ cell interactions and their significance in germ cell movement in the seminiferous epithelium during spermatogenesis. Endocr Rev. 2004;25(5):747-806. [PubMed ID: 15466940]. https://doi.org/10.1210/er.2003-0022.
-
30.
Rizk SM, Zaki HF, Mina MA. Propolis attenuates doxorubicin-induced testicular toxicity in rats. Food Chem Toxicol. 2014;67:176-86. [PubMed ID: 24593989]. https://doi.org/10.1016/j.fct.2014.02.031.
-
31.
Kasahara E, Sato EF, Miyoshi M, Konaka R, Hiramoto K, Sasaki J, et al. Role of oxidative stress in germ cell apoptosis induced by di(2-ethylhexyl)phthalate. Biochem J. 2002;365(Pt 3):849-56. [PubMed ID: 11982482]. https://doi.org/10.1042/BJ20020254.
-
32.
Ding Y, Dai X, Jiang Y, Zhang Z, Bao L, Li Y, et al. Grape seed proanthocyanidin extracts alleviate oxidative stress and ER stress in skeletal muscle of low-dose streptozotocin- and high-carbohydrate/high-fat diet-induced diabetic rats. Mol Nutr Food Res. 2013;57(2):365-9. [PubMed ID: 23161660]. https://doi.org/10.1002/mnfr.201200463.
-
33.
Roychowdhury S, Wolf G, Keilhoff G, Bagchi D, Horn T. Protection of primary glial cells by grape seed proanthocyanidin extract against nitrosative/oxidative stress. Nitric Oxide. 2001;5(2):137-49. [PubMed ID: 11292363]. https://doi.org/10.1006/niox.2001.0335.
-
34.
Puiggros F, Llopiz N, Ardevol A, Blade C, Arola L, Salvado MJ. Grape seed procyanidins prevent oxidative injury by modulating the expression of antioxidant enzyme systems. J Agric Food Chem. 2005;53(15):6080-6. [PubMed ID: 16028999]. https://doi.org/10.1021/jf050343m.
-
35.
Kabir F, Tow WW, Hamauzu Y, Katayama S, Tanaka S, Nakamura S. Antioxidant and cytoprotective activities of extracts prepared from fruit and vegetable wastes and by-products. Food Chem. 2015;167:358-62. [PubMed ID: 25148998]. https://doi.org/10.1016/j.foodchem.2014.06.099.
-
36.
Ray SD, Parikh H, Hickey E, Bagchi M, Bagchi D. Differential effects of IH636 grape seed proanthocyanidin extract and a DNA repair modulator 4-aminobenzamide on liver microsomal cytochrome 4502E1-dependent aniline hydroxylation. Mol Cell Biochem. 2001;218(1-2):27-33. [PubMed ID: 11330834].
-
37.
Yildirim NC, Kandemir FM, Benzer F. Beneficial effects of grape seed extract against cisplatin-induced testiculer damage in rabbits. Digest J Nanomater Biostruct. 2011;6(1):155-9.
-
38.
Li SG, Ding YS, Niu Q, Xu SZ, Pang LJ, Ma RL, et al. Grape Seed Proanthocyanidin Extract Alleviates Arsenic-induced Oxidative Reproductive Toxicity in Male Mice. Biomed Environ Sci. 2015;28(4):272-80. [PubMed ID: 25966753]. https://doi.org/10.3967/bes2015.038.