Abstract
Background:
Antioxidant compounds have been shown to slow down the development of chemically induced tumors. It has been estimated that most of human cancer cases are due to environmental factors and would be preventable if the main risks could be identified. The potential of vitamin E as a cancer preventive agent has been studied and individuals whose intake of vitamin E was above average showed a low risk of lung cancer. Vitamin E is a mixture of four lipid soluble phenols including α-, β-, γ-, and δ-tocopherol.Objectives:
In our study, to investigate the antioxidant activity of α-tocopherol-derived radicals in comparison with other phenoxyl radicals, a number of substituted phenols were synthesized, most of which contained t-butyl groups in the 2- and 6-positions.Materials and Methods:
All the synthesized compounds were recrystallized and the nuclear magnetic resonance (NMR) spectra were run on Bruker GRYOSPES WM 360 machine; the IR spectra were also run on a Perkin Elmer 1430 ratio recording infrared spectrophotometer. Microanalyses were carried out on a Perkin Elmer 4000 CHN analyzer; all the melting points were measured by an electrothermal melting point apparatus, which were uncorrected.Results:
Five synthesized vitamin E analogues, including 2, 6-di-t-butyl-4-nitrophenol, 2, 6-di-t-butyl-4-formylphenol, 2, 6-di-t-butyl-4aldoximephenol, 2, 6-di-t-butyl-4-cyanophenol, and 2, 4, 6-trimethoxyphenol, were analyzed by NMR, infrared (IR), and microanalysis, and their melting point were measured by the electrothermal melting point apparatus.Conclusions:
In this study, five vitamin E analogues were synthesized to compare their efficiencies with vitamin E as an antioxidant to be able to interfere in free radical chain reactions and terminate the propagation of free radicals. The efficacies of all five synthesized vitamin E analogues in terms of antioxidant activity and their kinetic study will be investigated in our other study.Keywords
1. Background
Many chemical carcinogens are able to generate free radicals by enzymatic reactions (1, 2). The relationship between cancer and free radicals has been studied (3, 4). Antioxidant compounds have been shown to slow down the development of chemically induced tumors (5-8). It has been estimated that the most of human cancer cases are due to environmental factors and would be preventable if the main risks could be identified (9, 10). The role of diet is important and a number of studies have suggested that absence of certain dietary compounds, notably antioxidants, contribute to increasing the risk of malignancy (11-14). There has been much interest in vitamin E, a biologically active phenol. Studies in animals have shown that vitamin E can prevent the growth of tumors induced in animals by chemical carcinogens (15-17). The potential of vitamin E as a cancer preventive agent has been studied and individuals whose intakes of vitamin E were above average showed a low risk of lung cancer (18). Vitamin E acts as a radical scavenger which inhibits peroxidation (19). It has been shown that in vitro, the oxidation of low density lipoprotein takes place only after complete consumption of vitamin E (20).
Biological membranes may be damaged by oxidation through chain reactions that generate lipid peroxyl radicals. Oxidation of the membrane results in impaired biological function and may lead to cell death (21-23). Vitamin E is the most important lipid-soluble biological antioxidant which scavenges peroxyl radicals and inhibits lipid peroxidation in vivo (24).
Vitamin E is a mixture of four lipid soluble phenols designated as α-, β-, γ-, and δ-tocopherol (Figure 1).
The Structure of Vitamin E Analogues
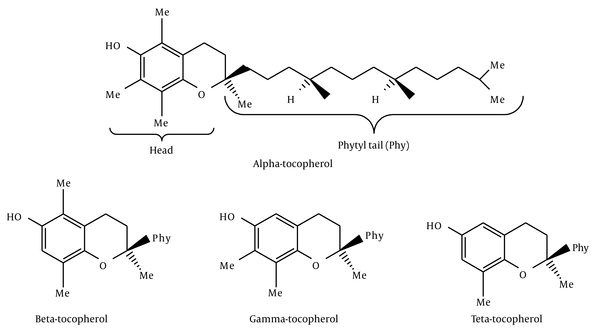
Tocopherols have terpenoid structure and they have a vitamin character because they do not originate in animal tissues. Vitamin E is a naturally occurring preservative found in vegetable oil, especially in wheat germ oil (25, 26). Vitamin E is a very important biological antioxidant and it is generally accepted that its most effective component is α-tocopherol.
2. Objectives
In our study, to investigate the stabilization of α-tocopherol-derived radicals in comparison to other phenoxyl radicals, a number of substituted phenols were synthesized, most of which contained t-butyl groups in 2- and 6-positions.
3. Materials and Methods
Chemical reagents were of analytical grade, purchased from Merck Co. (Germany) and all the synthesized compounds were recrystallized. The nuclear magnetic resonance (NMR) spectra were run on Bruker GRYOSPES WM 360 machine and the infrared (IR) spectra were run on a Perkin Elmer 1430 ratio recording infrared spectrophotometer. Microanalyses were carried out on a Perkin Elmer 4000 CHN analyzer; all the melting points were measured by an electrothermal melting point apparatus, and were uncorrected.
3.1. Preparation of Vitamin E Analogues
3.1.1. 2, 6-Di-t-Butyl-4-Nitrophenol
Fifteen milliliters of a 1:1 (v/v) mixture of nitric acid and acetic acid was added dropwise during 20 minutes to a stirred solution of 25 g of 2, 6-di-t-butylphenol in cyclohexane (75 mL). Barnes et al. (27) made the solution of 2, 6-di-t-butylphenol in acetic acid instead of cyclohexane. During the reaction, the solution was kept at 12 - 18°C. The solid 2, 6-di-t-butyl-4-nitrophenol was filtered, washed with water, and dried (Figure 2).
Vitamin E Analogues
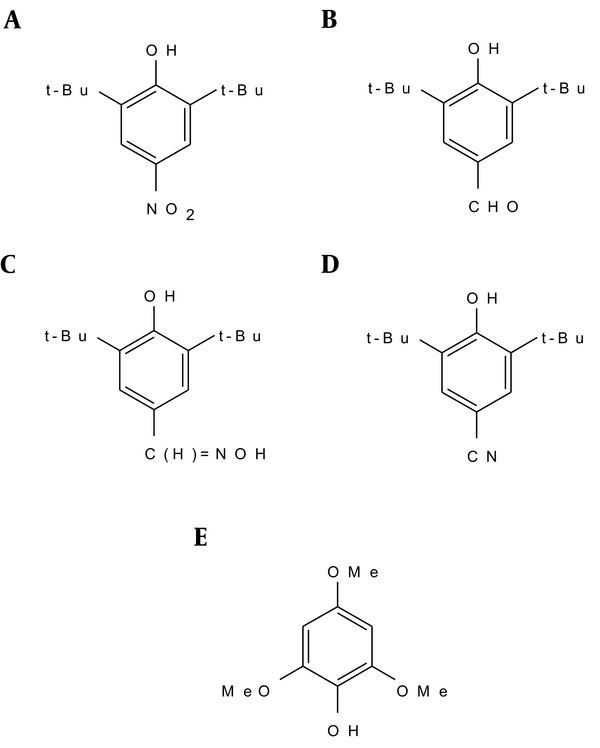
3.1.2. 2, 6-di-t-Butyl-4-Formylphenol
2, 6-di-t-butyl-4-methylphenol (80 g) was dissolved in glacial acetic acid (800 mL) and the solution was diluted with water (200 mL). T-butyl alcohol was used as the solvent instead of acetic acid by Coppinger et al. (28), in their preparation of 2, 6-di-t-butyl-4-formylphenol. Bromine (37 mL, 2 equivalents) was added dropwise with mechanical stirring over two hours. The suspension was diluted with ice water (500 mL). The mixture was kept at 0°C for several hours and was then filtered. The pale yellow product was washed with 50% acetic acid and water and was dried under reduced pressure (Figure 2).
3.1.3. 2, 6-di-t-Butyl-4 Aldoxime Phenol
The oxime was prepared from crude 2, 6-di-t-butyl-4-formylphenol by refluxing 2,6-di-t-butyl-4-formylphenol (30 g, 0.128 mol) with hydroxylamine hydrochloride (17.6 g, 0.253 mol), pyridine (40.3 g) and ethanol (75.6 mL) for three hours at 100°C, with stirring, while the reaction was in progress. After cooling, the product was shaken with H2O (150 mL), ether (20 mL), and NaCl (10 g). Muller et al. (29) prepared the oxime this way, but they did not separate it. The oxime-containing phase (upper layer) was evaporated down to give a colorless oil, which was dissolved in petroleum-ether [80 - 100°C] (130 mL), and concentrated by removing the solvent under reduced pressure until it became syrupy, but did not crystallize (Figure 2).
Recrystallization from petroleum-ether (60 - 80°C) gave a semi-solid material. This was dissolved in hot ethanol, and then diluted with water (dropwise) until the solution became cloudy. One further drop of ethanol was added, and after cooling, colorless crystals of the oxime separated and dried under vacuum at 98°C for 12 hours.
3.1.4. 2, 6-di-t-Butyl-4-Cyanophenol
A solution of 2, 6-di-t-butyl-4 aldoxime phenol (12.45 g, 0.05 mol) in 25 mL of acetic anhydride was heated on a steam bath for two hours. Then, the solution was poured into 1 M sodium bicarbonate (500 mL) and was stirred until the hydrolysis of the acetic anhydride was complete. The brown precipitate was extracted with 2 × 75 mL of methylene chloride, washed with sodium bicarbonate, and dried over sodium sulphate. Concentration by removing the solvent under reduced pressure afforded a brown solid material. The product was dissolved in ether and extracted with 1 M NaOH. The alkaline extract was acidified with 3 M hydrochloric acid and extracted with ether (Figure 2).
3.1.5. 2, 4, 6-Trimethoxyphenol
2, 4, 6-Trimethoxybenzaldehyde (0.981 g) and hydrogen peroxide (27.5%, 0.79 g) together with a catalytic amount of H2SO4 (98%, 0.1 mL) in methanol (10 mL) was stirred under argon atmosphere at room temperature for two hours. The solution was filtered and diluted with ether (30 mL). Extraction by 30 mL NaOH (2 M), followed by acidification of the aqueous layer with hydrochloric acid and extraction with 2 × 30 mL ether, gave a liquid product after evaporation of the solvent. This was dissolved in CH2Cl2 and purified by column chromatography (SiO2) (Figure 2).
4. Results
4.1. 2, 6-di-t-Butyl-4-Nitrophenol
The synthesized 2, 6-di-t-butyl-4-nitrophenol was then recrystallized three times from petroleum ether (40 - 60°C), giving 2, 6-di-t-butyl-4-nitrophenol as colorless needles (8.9 g, 29%), m.p. 154 - 155°C. δH (CDCl3) 1.46 (18 H, s, t-Bu), 5.91 (1 H, s, OH), and 8.10 (2 H, s, Harom). Found: C, 66.9; H, 8.4; N, 5.2. Calculated for C14H21NO3: C, 66.9; H, 8.4; N, 5.6%. The IR spectrum of 2, 6-di-t-butyl-4-nitrophenol in Nujol showed a sharp non-hydrogen bonded hydroxyl absorption at 3518 cm-1, and NO2 at 1595 cm-1.
4.2. 2, 6-di-t-Butyl-4-Formylphenol
Recrystallization of 2, 6-di-t-butyl-4-formylphenol from petroleum-ether (80 - 100°C), afforded pale yellow crystals, m.p. 189 - 190°C. δH (CDCl3) 1.46 (18 H, s, t-Bu), 5.82 (1 H, s, OH), 7.71 (2 H, s, Harom) and 9.83 (1 H, s, CHO). Found: C, 76.6; H, 9.5. Calculated for C15H22O2: C, 76.9; H, 9.5%. The IR spectrum of 2, 6-di-t-butyl-4-formylphenol in Nujol showed a hydroxyl absorption at 3400 cm-1, H-CO at 2715 cm-1 and C = O at 1652 cm-1.
4.3. 2, 6-di-t-Butyl -4 Aldoxime Phenol
The yield for 2, 6-di-t-butyl -4 aldoxime phenol was 14 g (44%), m.p.135 - 136°C. δH (CDCl3) 1.46 (18 H, t-Bu), 5.45 (1 H, s, OH), 7.27 (1 H, s, C = NOH), 7.40 (2 H, s, Harom), 8.08 (1 H, s, HC = NOH). The 1HNMR spectrum in D2O resulted in the exchange of protons by deuterium cations to give ArO-D and HC = NOD. The disappearance of the peaks at 5.45, 7.27 PPM indicated that these peaks were due to ArO-H and HCNO-H, respectively. The IR spectrum of 2, 6-di-t-butyl-4 aldoxime phenol in Nujol showed a hydroxyl absorption at 3300 cm-1 (phenol) and 3559 cm-1 (CH = NOH).
4.4. 2, 6-di-t-Butyl-4-Cyanophenol
The solvent was removed from the ether extract and the product was dried under vacuum and recrystallized from petroleum-ether (60 - 80°C), m.p. 144 - 145°C. δH (CDCl3) 1.44 (18 H, s, t-Bu), 5.75 (1 H, s, OH), and 7.47 (2 H, s, Harom). Found: C, 76.0; H, 9.0; N, 5.7. Calculated for C15H21NO: C, 77.9; H, 9.2, N, 6.1%. The IR spectrum of 2, 6-di-t-butyl-4-cyanophenol in Nujol showed a hydroxyl absorption at 3490 cm-1 and nitrile absorption at 2222 cm-1.
4.5. 2, 4, 6-Trimethoxyphenol
The phenol was obtained from the second and third fractions and after evaporation of the solvent the product was recrystallized from petroleum-ether as yellow needles, m.p. 64 - 66°C, δH (CDCl3) 3.75 (3 H, s, p-OCH3), 3.85 (6 H, s, o-OCH3), 5.08 (1 H, s, OH) and 6.17 (2 H, s, Harom). Found: C, 58.5; H, 6.5. Calculated for C9H12O4: C, 58.7; H, 6.6%. The IR spectrum for 2, 4, 6-trimethoxyphenol in Nujol shoed a hydroxyl absorption at 3434 cm-1, but because the methoxy peak was covered by the Nujol peak, another IR sample of 2, 4, 6-trimethoxyphenol in KBr was run, which showed hydroxyl absorption at 3434 cm-1 and methoxy absorption at 2844 cm-1.
5. Discussion
2, 6-Di-t-butyl-4-nitrophenol was obtained by electrophilic substitution involving N+O2.
In preparation of 2, 6-di-t-butyl-4-formyl phenol, it can be suggested that electrophilic addition of Br+ followed by loss of a proton leads to quinine.
Hydration of the methylene quinone gives out the phenol. Electrophilic addition of the second Br+ leads to methanol quinone followed by protonation, and deprotonation of quinone gives the formyl phenol (Figure 3).
The Pathway From 2, 6-di-t-Butyl-4-Methylphenol to 2, 6-di-t-Butyl-4-Formylphenol
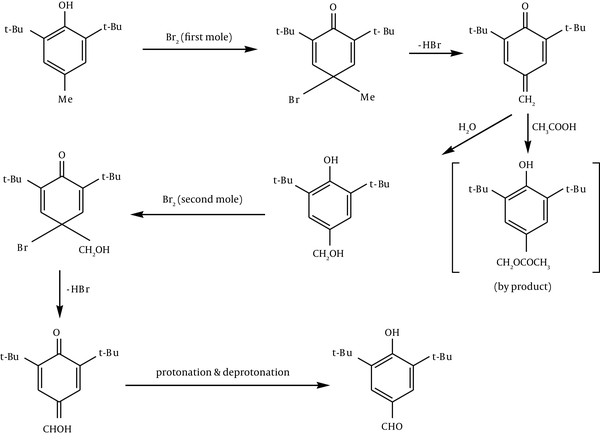
In preparation of 2, 6-di-t-butyl-4-aldoximephenol, hydroxylamine condenses with the aldehyde group to give the oxime.
Dehydration of the oxime leads to formation of cyanophenol.
It can be suggested for the preparation of 2, 4, 6-tri-methoxy phenol that electrophilic addition of methanol to the aldehyde leads to hemiacetal.
Nucleophilic addition of hydrogen peroxide gives peroxyhemiacetal. Then protonation of peroxyhemiacetal followed by simultaneous dehydration and aryl migration gives the cation (aryl migration occurs to avoid the formation of an electron-deficient oxygen atom). After rearrangement, hydration leads to the ortho ester of the carboxylic acid. Loss of formic acid from the ortho ester provides phenol (Figure 4).
Mechanism of 2, 4, 6-Trimethoxyphenol Formation From 2, 4, 6-Trimethoxybenzaldehyde
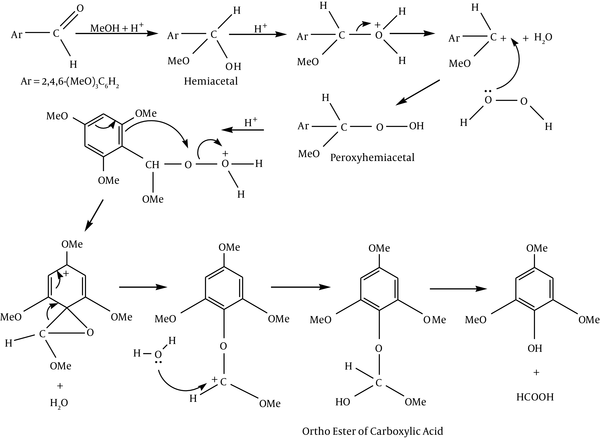
Autoxidation can cause damage to lipids and proteins. Antioxidant compounds have been shown to slow down the development of chemically induced tumors (30). There has been much interest in vitamin E as a radical scavenger which inhibits peroxidation. Vitamin E is a biologically active phenol which can easily convert to free radical and act as radical scavenger. Vitamin E radical is a phenoxyl radical which is more stable in comparison with an alkoxyl radical. This stability is due to the delocalization of the unpaired electron to the ortho and para position of the benzene ring.
The main point of our study was finding a compound much more reactive than phenol or vitamin E in terms of radical scavenger. For this purpose, we synthesized five vitamin E analogues to compare their efficiencies with vitamin E as an antioxidant to be able to interfere in the free radical chain reaction and terminate the propagation of free radicals. The efficacy of all five synthesized vitamin E analogues in terms of antioxidant activity and their kinetic study will be investigated in our other study.
Acknowledgements
References
-
1.
Samra ZQ, Pervaiz S, Shaheen S, Dar N, Athar MA. Determination of oxygen derived free radicals producer (xanthine oxidase) and scavenger (paraoxonase1) enzymes and lipid parameters in different cancer patients. Clin Lab. 2011;57(9-10):741-7. [PubMed ID: 22029190].
-
2.
Floyd RA. Free radicals and cancer. M. Dekker; 1982.
-
3.
Nogues MR, Giralt M, Cervello I, Del Castillo D, Espeso O, Argany N, et al. Parameters related to oxygen free radicals in human skin: a study comparing healthy epidermis and skin cancer tissue. J Invest Dermatol. 2002;119(3):645-52. [PubMed ID: 12230508]. https://doi.org/10.1046/j.1523-1747.2002.00077.x.
-
4.
Pryor WA. Free Radicals and Cancer. New York: Academic Press; 1980.
-
5.
Zargari M, Sedighi O. Influence of Hemodialysis on Lipid Peroxidation, Enzymatic and Non-Enzymatic Antioxidant Capacity in Chronic Renal Failure Patients. Nephrourol Mon. 2015;7(4). eee28526. [PubMed ID: 26539417]. https://doi.org/10.5812/numonthly.28526.
-
6.
Kok YY, Mooi LY, Ahmad K, Sukari MA, Mat N, Rahmani M, et al. Anti-tumour promoting activity and antioxidant properties of girinimbine isolated from the stem bark of Murraya koenigii S. Molecules. 2012;17(4):4651-60. [PubMed ID: 22522395]. https://doi.org/10.3390/molecules17044651.
-
7.
Petri A, Alexandratou E, Kyriazi M, Rallis M, Roussis V, Yova D. Combination of Fospeg-IPDT and a natural antioxidant compound prevents photosensitivity in a murine prostate cancer tumour model. Photodiagnosis Photodyn Ther. 2012;9(2):100-8. [PubMed ID: 22594979]. https://doi.org/10.1016/j.pdpdt.2011.11.002.
-
8.
Mohammadi M, Alipour M, Alipour MR, Vatankhah AM. Effects of high cholesterol diet and parallel chronic exercise on erythrocyte primary antioxidant enzymes and plasma total antioxidant capacity in Dutch rabbits. Int J Endocrinol Metabol. 2006;2006(1, Winter):30-40.
-
9.
Ames BN, Magaw R, Gold LS. Ranking possible carcinogenic hazards. Science. 1987;236(4799):271-80. [PubMed ID: 3563506].
-
10.
Gold LS, Slone TH, Stern BR, Manley NB, Ames BN. Rodent carcinogens: setting priorities. Science. 1992;258(5080):261-5. [PubMed ID: 1411524].
-
11.
Eilati E, Bahr JM, Hales DB. Long term consumption of flaxseed enriched diet decreased ovarian cancer incidence and prostaglandin E(2)in hens. Gynecol Oncol. 2013;130(3):620-8. [PubMed ID: 23707669]. https://doi.org/10.1016/j.ygyno.2013.05.018.
-
12.
Bosetti C, Turati F, Dal Pont A, Ferraroni M, Polesel J, Negri E, et al. The role of Mediterranean diet on the risk of pancreatic cancer. Br J Cancer. 2013;109(5):1360-6. [PubMed ID: 23928660]. https://doi.org/10.1038/bjc.2013.345.
-
13.
Heslin MJ, Brennen MF. Diet and malignancy: five decades of rediscovery. Nutrition. 1996;12(9):653. [PubMed ID: 8998378].
-
14.
Ames BN. Prevention of mutation, cancer, and other age-associated diseases by optimizing micronutrient intake. J Nucleic Acids. 2010;2010. [PubMed ID: 20936173]. https://doi.org/10.4061/2010/725071.
-
15.
Agarwal MK, Iqbal M, Athar M. Vitamin E inhibits hepatic oxidative stress, toxicity and hyperproliferation in rats treated with the renal carcinogen ferric nitrilotriacetate. Redox Rep. 2005;10(2):62-70. [PubMed ID: 15949125]. https://doi.org/10.1179/135100005X21642.
-
16.
Niki E. Role of vitamin E as a lipid-soluble peroxyl radical scavenger: in vitro and in vivo evidence. Free Radic Biol Med. 2014;66:3-12. [PubMed ID: 23557727]. https://doi.org/10.1016/j.freeradbiomed.2013.03.022.
-
17.
Butterfield DA, Koppal T, Subramaniam R, Yatin S. Vitamin E as an antioxidant/free radical scavenger against amyloid beta-peptide-induced oxidative stress in neocortical synaptosomal membranes and hippocampal neurons in culture: insights into Alzheimer's disease. Rev Neurosci. 1999;10(2):141-9. [PubMed ID: 10658956].
-
18.
Menkes MS, Comstock GW, Vuilleumier JP, Helsing KJ, Rider AA, Brookmeyer R. Serum beta-carotene, vitamins A and E, selenium, and the risk of lung cancer. N Engl J Med. 1986;315(20):1250-4. [PubMed ID: 3773937]. https://doi.org/10.1056/NEJM198611133152003.
-
19.
Delfan B, Zarei F, Iravani S, Ebrahimzadeh F, Adineh A, Sepahvand R, et al. Vitamin E and Omega-3, 6 and 9 Combinations Versus Vitamin E in the Treatment of Mastodynia. Jundishapur J Natr Pharm Prod. 2015;10(2). https://doi.org/10.17795/jjnpp-18659.
-
20.
Traber MG, Atkinson J. Vitamin E, antioxidant and nothing more. Free Radic Biol Med. 2007;43(1):4-15. [PubMed ID: 17561088]. https://doi.org/10.1016/j.freeradbiomed.2007.03.024.
-
21.
Marouf BH, Zalzala MH, Al-Khalifa II, Aziz TA, Hussain SA. Free radical scavenging activity of silibinin in nitrite-induced hemoglobin oxidation and membrane fragility models. Saudi Pharm J. 2011;19(3):177-83. [PubMed ID: 23960757]. https://doi.org/10.1016/j.jsps.2011.03.006.
-
22.
Sargis RM, Subbaiah PV. Protection of membrane cholesterol by sphingomyelin against free radical-mediated oxidation. Free Radic Biol Med. 2006;40(12):2092-102. [PubMed ID: 16785023]. https://doi.org/10.1016/j.freeradbiomed.2006.02.005.
-
23.
Konovalova GG, Lisina MO, Tikhaze AK, Lankin VZ. A complex of antioxidant vitamins effectively inhibits free-radical oxidation of LDL phospholipids in blood plasma and membrane structures of the liver and myocardium. Bull Exp Biol Med. 2003;135(2):143-6. [PubMed ID: 12802419].
-
24.
Alessi M, Paul T, Scaiano JC, Ingold KU. The contrasting kinetics of peroxidation of vitamin E-containing phospholipid unilamellar vesicles and human low-density lipoprotein. J Am Chem Soc. 2002;124(24):6957-65. [PubMed ID: 12059219].
-
25.
Leenhardt F, Fardet A, Lyan B, Gueux E, Rock E, Mazur A, et al. Wheat germ supplementation of a low vitamin E diet in rats affords effective antioxidant protection in tissues. J Am Coll Nutr. 2008;27(2):222-8. [PubMed ID: 18689553].
-
26.
Ge Y, Yan H, Hui B, Ni Y, Wang S, Cai T. Extraction of natural vitamin E from wheat germ by supercritical carbon dioxide. J Agric Food Chem. 2002;50(4):685-9. [PubMed ID: 11829628].
-
27.
Barnes TJ, Hickinbottom WJ. 196. 4-Nitro-2,6-di-t-butylphenol and its thermal decomposition. J Chem Soc. 1961:953. https://doi.org/10.1039/jr9610000953.
-
28.
Coppinger GM, Campbell TW. Reaction between 2,6-Di-t-butyl-p-cresol and Bromine. J Am Chem Soc. 1953;75(3):734-6. https://doi.org/10.1021/ja01099a067.
-
29.
Muller E, Rieker A, Ley K, Mayer R, Scheffler K. Über Sauerstoffradikale, XII. Das 4-Cyan-2.6-di-tert.-butyl-phenoxyl-(1). Chemische Berichte. 1959;92(9):2278-93. https://doi.org/10.1002/cber.19590920946.
-
30.
Kovalchuk A, Aladedunye F, Rodriguez-Juarez R, Li D, Thomas J, Kovalchuk O, et al. Novel antioxidants are not toxic to normal tissues but effectively kill cancer cells. Cancer Biol Ther. 2013;14(10):907-15. [PubMed ID: 23917379]. https://doi.org/10.4161/cbt.25935.