Abstract
Background:
Gentamicin is a commonly used antibiotic for the treatment of Gram-negative infections, but nephrotoxicity limits its use. Cyperus scariosus Linn. (CS) has been found to have antioxidant properties in vitro.Objectives:
The aim of this study was to evaluate the nephroprotective effects of CS in gentamicin-induced acute kidney injury (AKI).Methods:
The animals were divided into nine groups. AKI was produced with a 100 mg/kg intraperitoneal (i.p.) injection of gentamicin for 7 days. Next, α-lipoic acid 100 mg/kg i.p. served as the active control, while the test drug (CS) was given in two doses (150 mg/kg and 250 mg/kg orally) for 10 days. Distilled water, 1 ml/day orally for 7 days, served as the vehicle control. The protective and curative effects, respectively, were assessed by the administration of CS before and after the induction of AKI. The effects of CS on AKI were assessed by serological and histopathological parameters.Results:
Serum creatinine was significantly increased (P < 0.05) while 24 hours urine output, urine creatinine, and serum protein were significantly decreased (P < 0.05) in rats treated with gentamicin as compared to the control group. As the active control, α-lipoic acid showed nephroprotective effects on the urinary, serological, and histopathological parameters. C. scariosus Linn., as a preventive and curative therapy, restored urine output and altered the serological parameters non-significantly compared to the disease-control group. It also preserved the normal kidney architecture, as evidenced by histopathological parameters.Conclusions:
In our study, hydroalcoholic extract of Cyperus scariosus Linn. offered nephroprotection in the form of AKI prevention and for the treatment of established AKI.Keywords
Gentamicin Acute Kidney Injury Oxidative Stress Nephroprotective Cyperus scariosus Linn.
1. Background
Acute kidney injury (AKI) is a clinical syndrome characterized by a rapidly declining glomerular filtration rate (GFR), imbalances in electrolytes and in the acid-base level, derangement of extracellular fluid volume, and retention of nitrogenous waste products, often associated with reduced urine output (1). AKI develops rapidly, within a few hours to weeks, and is usually reversible. It can occur either in previously normal kidneys (classic AKI) or in patients with pre-existing chronic renal disease, such as glomerulonephropathies and other syndromes affecting the kidneys (‘acute-on-chronic’ renal failure).
AKI is a significant problem, affecting approximately 5% of all hospitalized patients (2) and 5% - 25% of all patients admitted to intensive care units (3, 4). A significant number of patients with AKI ultimately progress to end-stage renal disease (ESRD), resulting in dialysis and renal transplantation (5). Aminoglycoside antibiotics, including gentamicin, are commonly used in the treatment of various Gram-negative infections. A major complication with the use of this group of drugs is nephrotoxicity, accounting for 10% - 15% of all cases of acute renal failure (6). It has been proposed that aminoglycoside accumulation in renal proximal tubular epithelial cells causes membrane structural disturbances and cell death by involving reactive oxygen species (ROS) (7). ROS produce cellular injury and necrosis via several mechanisms, including peroxidation of membrane lipids, protein denaturation, and DNA damage (8). Renal accumulation of gentamicin and lysosomal phospholipidosis disrupts normal renal function and is implicated in the induction of nephrotoxicity (9, 10).
Dietary antioxidants are able to counteract reductions in the GFR and thus the severity of the tubular damage induced by gentamicin (11, 12). Cyperus scariosus R. Br. (Cyperaceae family; known locally as nagarmotha) is a delicate grass widely distributed in India, especially in Chhattisgarh, Bihar, Orissa, West Bengal, and Uttar Pradesh. Nagarmotha is also found in the eastern and southern areas of the Indo-Pak subcontinent, and in Bangladesh, South Africa, China, and the Pacific Islands; it is reported to be useful as a cordial, tonic, emmenagogue, vermifuge, diuretic, diaphoretic, and desiccant (13-15). Nagarmotha is prescribed by local herbal medicine practitioners to treat a variety of diseases, including diarrhea, epilepsy, gonorrhea, syphilis and liver damage (13-15). In one study, CS was found to contain phenols, flavonoids, and DPPH (1, 1-diphenyl-2-picrylhydrazyl) scavenger activity, all of which contribute to its antioxidant properties (16).
2. Objectives
The aim of this study was to evaluate the nephroprotective effects of a hydroalcoholic extract of CS on gentamicin-induced AKI in Wistar albino rats.
3. Methods
All of the experiments were performed after approval from the Institutional Animal Ethics Committee (IAEC), Government Medical College, Bhavnagar, Gujarat, India (Approval No: IAEC 36/2014) and in accordance with the guidelines issued by the Committee for the Purpose of Control and Supervision of Experiments on Animals (CPCSEA), Ministry of Environment and Forest, Government of India. Wistar albino rats (both sexes, weight 250 ± 50 g) were procured from the central animal house of the institute. They were housed in standard transparent polycarbonate cages and kept under a 12-hour light/dark cycle with controlled room temperature (25 ± 2°C). The animals were allowed to acclimatize to the laboratory conditions for at least one week before starting the experiments. They were provided a standard laboratory diet and were handled under the guidelines of Good Laboratory Practice (GLP).
3.1. Drugs and Chemicals
Dried hydroalcoholic extract of CS root was procured from Kuber Impex, Indore, India. To obtain fresh solution for dosing, the dried extract powder was dissolved in distilled water and 50 mg/mL of solution was prepared. Gentamicin solution vials were procured from Sir Takhatsinhji General Hospital, Bhavnagar (Nirma Limited, Sachana, Gujarat, India; batch no. 5A40015) and α-lipoic acid was procured from Sigma Chemical Company (St. Louis, MO, USA). It was dissolved in ground nut oil and 50 mg/ml of solution was prepared before the dosing.
3.2. Experimental Design and Procedure
Seventy-two rats were randomly assigned (using a trial version of Rando software version 1.2) to nine equal groups as follows:
Group I (Normal control): received distilled water in doses of 1 mL/day orally for 7 days.
Group II (Disease control): received gentamicin intraperitoneally (i.p.) in doses of 100 mg/kg for 7 days.
Group III (Test control): received hydroalcoholic extract of CS in doses of 250 mg/kg orally for 10 days.
Group IV (Active control - Preventive): received 100 mg/kg of α-lipoic acid i.p. for 10 days and gentamicin 100 mg/kg i.p. started on the 4th day (the first dose of α-lipoic acid was considered day 1) and given up to 10th day.
Group V (Active control - Curative): received 100 mg/kg of gentamicin i.p. for 7 days, followed by 100 mg/kg of α-lipoic acid i.p. for 10 days.
Groups VI and VII (Preventive): received CS orally in doses of 150 and 250 mg/kg, respectively, for 10 days; gentamicin 100 mg/kg i.p. was started on the 4th day and given up to 10th day.
Groups VIII and IX (Curative): received CS orally 150 and 250 mg/kg, respectively, for 10 days after i.p. injections of gentamicin 100 mg/kg for 7 days.
3.3. Outcome Measures
3.3.1. Urinary Parameters
On day 0, rats were kept in metabolic cages (B.I.K. Industries, Mumbai, India) with water and rations available ad libitum, and a 24-h urine collection was done to obtain baseline urinary parameters. At the end of the study period (7th day in groups I and II; 10th day in groups III, IV, VI, and VIII; and 17th day in groups V, VIII, and IX), a 24-hour urine collection was again performed to measure urine output and urinary creatinine.
3.3.2. Biochemical Parameters
The animals were anesthetized with ketamine (75 mg/kg) and xylazine (10 mg/kg) and blood was collected through the retro-orbital plexus from the inner canthus of the eye using a capillary tube, into plain and EDTA vacuum containers. The serum was separated by centrifugation and used for the estimation of blood urea, serum creatinine, electrolytes (sodium and potassium), liver function tests (serum glutamic oxaloacetic transaminase [SGOT] with the UV kinetic method, serum glutamic pyruvic transaminase [SGPT], and alkaline phosphatase [ALP] with the PMP-AMP kinetic method), serum proteins (Biuret method), serum bilirubin (modified Jendrassik and Groff method), and hemoglobin.
The animals were sacrificed according to the norms for the didactic scientific practice of animal vivisection. The kidneys were removed, and one kidney from each animal was kept in 10% (v/v) formaldehyde for further histopathological analysis while the other kidney was used for the antioxidant estimation.
3.3.3. Antioxidant Estimation in Kidney Tissue Homogenate Preparation
3.3.3.1. Kidney Homogenate Preparation
The kidneys were weighed and cross-chopped with a surgical scalpel into fine slices, then chilled in cold 0.25 M sucrose solution and quickly blotted with filter paper. The tissue was minced, then homogenized in ice-cold 10 mM Tris HCL (10% [w/v], 0.1 M, pH 7.4) with 25 strokes of a tight Teflon pestle of glass homogenizer at a speed of 2,500 rpm. The prolonged homogenization was designed to disrupt the cells in order to release the soluble proteins, leaving the nonsoluble particles as sediment.
The tissue homogenate was then centrifuged at 5,000 rpm at 4°C using a compact, high-speed, refrigerated centrifuge (Kubota 6500, Japan). The obtained supernatant was analyzed for levels of superoxide dismutase (SOD) by the method of Misra and Fridovich (17), glutathione (GSH) by the method of Morton et al. (18), and the marker of lipid peroxidation of malondialdehyde (MDA) by the method of Slater and Sawyer as described below(19).
3.3.3.2. Superoxide Dismutase (SOD)
Before starting the procedure, all reagents were kept refrigerated and added in the cold condition. For the procedure, 0.5 mL of tissue homogenate was diluted with 0.5 mL of distilled water. 0.25 ml ethanol and 0.15 mL chloroform were added. Blank was prepared similarly without tissue homogenate. The mixture was shaken for 1 minute and centrifuged at 2000 rpm for 20 minutes. The supernatant was separated. 0.5 mL of this supernatant was taken in another test tube and 1.5 ml of carbonate buffer and 0.5 mL of EDTA were added. Blank was similarly prepared. The reaction was initiated by the addition of 0.4 mL of epinephrine and change in optical density/min was measured at 480 nm and noted for 3 minutes at an interval of 30 seconds. It was expressed as U/mg protein. 50% inhibition of epinephrine to adrenochrome transition by enzyme was taken as enzyme unit.
3.3.3.3. Glutathione
To 1 ml of sample 1 mL of 10% TCA was added. The precipitated fraction was centrifuged and to 0.5 mL of supernatant, 2 mL of DTNB reagent was added. The final volume was made up to 3 mL with phosphate buffer. The color developed was read at 412 nm. The amount of glutathione was expressed as µg of GSH /mg protein.
3.3.3.4. Lipid Peroxidation or Malondialdehyde Formation
First, 2 mL of tissue homogenate was mixed with 2 mL of 20% trichloroacetic acid and a blank was prepared by mixing 3 mL of distilled water with 3 mL of 20% trichloroacetic acid. Both solutions were kept on ice for 15 minutes. The precipitates were separated by centrifugation at 2,000 rpm for 10 minutes. Next, 2 mL of supernatant from the test solution and 3 mL of supernatant from the blank were mixed with 2 mL of 0.67% thiobarbituric acid, respectively. These mixtures were heated for 10 minutes then cooled for 5 minutes, and absorbance was read at 535 nm. The values were expressed as nanomoles (nM) of MDA formed per gram of tissue.
3.4. Histopathological Analysis
The kidneys were preserved in 10% (v/v) formalin, processed, and embedded in paraffin wax, then cut into 5 - 6 μm sections and stained with hematoxylin and eosin (H & E) and analyzed by light microscopy to evaluate for AKI. Histopathological observations for necrosis, epithelial cell desquamation (ECD), flat epithelial degeneration (FED), casts, and edema were graded from grade 0 to 4 according to severity of the changes. Grade 0 indicated no injury and +1, +2, +3, and +4 indicated 1% - 25%, 26% - 50%, 51% - 75%, and 76% - 100% injury, respectively.
3.5. Statistical Analysis
All values were expressed as mean ± standard error of mean (SEM). Serum potassium levels between the groups was compared by one-way ANOVA followed by Tukey’s multiple-comparisons test. Blood urea, serum creatinine, urine creatinine, electrolytes, SGPT, SGOT, ALP, serum proteins, serum bilirubin, SOD, GSH, MDA and histopathological parameters were analyzed with the Kruskal-Wallis test, followed by Dunn’s multiple-comparisons test. GraphPad InStat 3.0 (demo version, GraphPad Software, CA, USA) was used for statistical analysis. P values of < 0.05 were considered statistically significant.
4. Results
Out of 72 rats, nine (12.5%) expired after the injection of gentamicin. We acquired the minimum of six evaluable animals per group after the occurrence of this mortality in the present study.
4.1. Effect of C. scariosus Linn. on Urinary Parameters
After administration of gentamicin for 7 days, 24-h urine output and urine creatinine were significantly reduced as compared to baseline values (P < 0.05); 150 mg/kg and 250 mg/kg of CS as preventive and curative therapies increased the 24-h urine output as compared to baseline levels, but could not achieve a statistically significant level. No effects on urine creatinine levels were found for the test drug. These observations are similar to the effect of α-lipoic acid on urinary parameters (Table 1).
Comparison of Urinary Parameters Between Study Groups at Baseline and End of the Experimenta,b
Days | Group I: Normal Control (n = 8) | Group II: Disease Control (n = 6) | Group III: Test Control (n = 8) | Group IV: Active Control - Preventive (n = 8) | Group V: Active Control - Curative (n = 6) | Group VI: Preventive – Low-Dose C. scariosus (n = 8) | Group VII: Preventive - high-dose C. scariosus (n = 6) | Group VIII: Curative - Low-Dose C. scariosus (n = 6) | Group IX: Curative - High-Dose C. scariosus (n = 7) |
---|---|---|---|---|---|---|---|---|---|
Urine output, mL/d | |||||||||
Baseline | 9.88 ± 1.9 | 18.58 ± 2.3 | 6.56 ± 1.5 | 7.25 ± 1.0 | 7.17 ± 0.9 | 9.13 ± 2.1 | 11.43 ± 3.2 | 8.16 ± 0.6 | 7.29 ± 1.0 |
End of experiment | 10.81 ± 2.2 | 5.43 ± 1.1 | 6.63 ± 0.9 | 18.44 ± 3.3 | 13.42 ± 3.4 | 12 ± 4.1 | 20 ± 5.6 | 16.5 ± 3.8 | 15.43 ± 3.4 |
P Value | 0.6645 | 0.0313c | 0.9730 | 0.0075 | 0.0625c | 0.5469c | 0.2188c | 0.0849 | 0.07 |
Urine creatinine, mg/dL | |||||||||
Baseline | 68.04 ± 14.4 | 70.8 ± 24.6 | 55.25 ± 13.0 | 7.83 ± 3.5 | 6.74 ± 2.3 | 23.34 ± 6.9 | 37.67 ± 21.2 | 29.83 ± 8.9 | 22.46 ± 14 |
End of experiment | 48.29 ± 9.5 | 23.26 ± 5.2 | 25.56 ± 13.6 | 6.86 ± 2.1 | 5.24 ± 1.5 | 12.49 ± 6.9 | 7.97 ± 2.1 | 6.37 ± 2.7 | 3.03 ± 0.6 |
P Value | 0.1731 | 0.0313c | 0.2500c | 0.8036 | 0.5664 | 0.2500c | 0.7422 | 0.0631c | 0.2969c |
4.2. Effect of C. scariosus Linn. on Biochemical Markers
After injection of gentamicin for 7 days, serum creatinine and protein levels significantly increased and decreased, respectively, as compared to the normal control group (P < 0.01) (Table 2). Blood urea, serum sodium, and potassium levels were altered after gentamicin administration for 7 days, but could not achieve statistical significance (Table 2). Administration of CS in 150 mg/kg and 250 mg/kg doses as preventive and curative therapies restored the altered kidney parameters caused by gentamicin. However, statistical significance was achieved only with serum potassium levels when 150 mg/kg of CS was given as a curative therapy and 250 mg/kg CS was given as preventive and curative therapies (Table 2).
Study Group | Blood Urea, mg/dL | Serum Creatinine, mg/dL | Serum Sodium, mEq/L | Serum Potassiumb, mEq/L | Serum Protein, g/dL |
---|---|---|---|---|---|
Group I: normal control (n = 8) | 33.5 ± 2.5 | 0.54 ± 0.03 | 140.29 ± 1.04 | 4.94 ± 0.2 | 7.05 ± 0.2 |
Group II: disease control (n = 6) | 124.83 ± 41.8 | 2.88 ± 0.7c | 131.67 ± 4.9 | 7.02 ± 1.7 | 5.57 ± 0.2c |
Group III: test control (n = 8) | 38 ± 2.9 | 0.41 ± 0.02 | 140 ± 0.9 | 5.04 ± 0.2 | 7.37 ± 0.3 |
Group IV: active control - preventive (n = 8) | 40.75 ± 4.7 | 0.71 ± 0.1 | 134 ± 1.8 | 4.44 ± 0.2d | 5.45 ± 0.1 |
Group V: active control - curative (n = 6) | 19.5 ± 1.6d | 0.6 ± 0.02 | 135.67 ± 0.3 | 4.73 ± 0.2 | 5.53 ± 0.1 |
Group VI: preventive - low-dose C. scariosus (n = 8) | 58 ± 10.9 | 0.95 ± 0.2 | 138 ± 1.3 | 4.67 ± 0.3 | 7 ± 0.2 |
Group VII: preventive - high-dose C. scariosus (n = 6) | 70 ± 15.0 | 1.12 ± 0.2 | 140.17 ± 1.1 | 4.33 ± 0.1d | 6.73 ± 0.1 |
Group VIII: curative - low-dose C. scariosus (n = 6) | 38.33 ± 4.8 | 0.6 ± 0.05 | 141.83 ± 1.2 | 3.93 ± 0.1d | 6.57 ± 0.2 |
Group IX: curative - high-dose C. scariosus (n = 7) | 38.71 ± 5.9 | 0.66 ± 0.1 | 138.57 ± 1.7 | 4.02 ± 0.1d | 6.53 ± 0.2 |
P Value | 0.0007 | < 0.0001 | 0.0037 | 0.0125 | < 0.0001 |
Other biochemical parameters, including SGPT, SGOT, serum bilirubin, ALP, and hemoglobin, were not affected.
4.3. Effect of C. scariosus Linn. on Biomarkers of Oxidative Stress
Antioxidant parameters, including SOD, GSH, and MDA levels, were non-significantly reduced in the disease control group compared to the normal controls. As a preventive therapy, 150 mg/kg of CS significantly increased SOD levels (P < 0.05) compared to the disease control group (Table 3).
Study Group | Super Oxide Dismutase, Unit/g of Tissue | Glutathione, Unit/g of Tissue | Malondialdehyde, Unit/g of Tissue |
---|---|---|---|
Group I: normal control (n = 8) | 6.26 ± 2.9 | 22.13 ± 2.4 | 4.74 ± 2.5 |
Group II: disease control (n = 6) | 1.46 ± 0.6 | 9.38 ± 5.0 | 3.71 ± 1.4 |
Group III: test control (n = 8) | 14.56 ± 5.7 | 16.63 ± 6.4 | 5.85 ± 2.4 |
Group IV: active control - preventive (n = 8) | 10.43 ± 3.7 | 21.18 ± 5.2 | 1.5812 ± 0.2 |
Group V: active control - curative (n = 6) | 5.22 ± 2.1 | 14.33 ± 4.6 | 1.28 ± 0.1 |
Group VI: preventive - low-dose C. scariosus (n = 8) | 31.66 ± 6.6c | 23.38 ± 8.7 | 1.34 ± 0.2 |
Group VII: preventive - high-dose C. scariosus (n = 6) | 2.50 ± 1.4 | 14.57 ± 3.9 | 2.35 ± 0.5 |
Group VIII: curative - low-dose C. scariosus (n = 6) | 9.74 ± 3.7 | 68.67 ± 46.4 | 1.21 ± 0.1 |
Group IX: curative - high-dose C. scariosus (n = 7) | 10.3 ± 6.4 | 65.71 ± 40.5 | 4.38 ± 3.4 |
P Value | 0.0158 | 0.7672 | 0.0205 |
4.4. Effect of C. scariosus Linn. on Histopathological Parameters
Gentamicin administration for 7 days caused a significant increase in necrosis, epithelial cell desquamation, and cast formation in the kidneys (P < 0.05) (Table 4). Some flat epithelial degeneration and edema were also noted in the disease control group. CS as a preventive and curative therapy resulted in less kidney damage compared to the disease control group, and normal architecture of the kidney was maintained (Figure 1).
Study Group | Necrosis | Epithelial Cell Desquamation | Flat Epithelial Degeneration | Cast | Edema |
---|---|---|---|---|---|
Group I: normal control (n = 8) | 0 | 0 | 0 | 0 | 0 |
Group II: disease control (n = 6) | 1.83 ± 0.5b | 1.67 ± 0.2b | 0.67 ± 0.4 | 2.17 ± 0.3b | 1.33 ± 0.6 |
Group III: test control (n = 8) | 0 | 0c | 0 | 0c | 0 |
Group IV: active control - preventive (n = 8) | 0c | 0c | 0 | 0c | 0 |
Group V: active control - curative (n = 6) | 0.5 ± 0.2 | 0c | 0.33 ± 0.2 | 0c | 0.5 ± 0.3 |
Group VI: preventive - low-dose C. scariosus (n = 8) | 0.88 ± 0.4 | 0.63 ± 0.4c | 0.25 ± 0.2 | 1 ± 0.4 | 1.75 ± 0.3 |
Group VII: preventive – high-dose C. scariosus (n = 6) | 0.57 ± 0.4 | 0c | 0 | 0.57 ± 0.4c | 0.57 ± 0.4 |
Group VIII: curative - low-dose C. scariosus (n = 6) | 0c | 0.17 ± 0.2c | 0.5 ± 0.5 | 0.17 ± 0.2c | 0.17 ± 0.2 |
Group IX: curative – high-dose C. scariosus (n = 7) | 0.29 ± 0.3 | 0c | 0.29 ± 0.2 | 0c | 0.71 ± 0.4 |
Histopathological Images of Kidney Sections After H & E Staining Under Light Microscopy (10 ×)
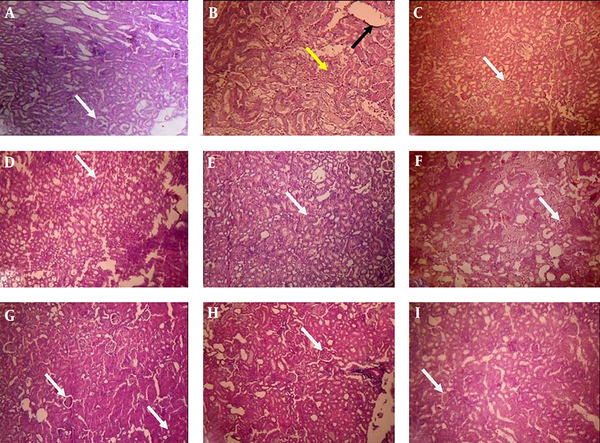
5. Discussion
In the present study, we evaluated the nephroprotective effects of a hydroalcoholic extract of CS in a gentamicin-induced AKI model. The gentamicin-induced nephrotoxicity model is an important and validated model used to study the nephroprotective potential of drugs and phytopharmaceuticals. Gentamicin-induced AKI is considered to be due to interactions between cationic aminoglycosides and membrane anionic phospholipids, and it is the first cytotoxic step in kidney injury (11, 12). Gentamicin is filtered through the glomeruli into tubules and binds with anionic phospholipids, such as phosphatidylinositol or phospholipdylserine, in the brush borders of the proximal tubular cells. Then, it is reabsorbed actively via pinocytosis into tubular cells and taken up by lysosomes, and thereafter produces phospholipidosis and apoptosis in proximal tubular cells (20, 21). It is manifested clinically as nonoliguric renal failure, with a slow rise in serum creatinine, blood urea nitrogen, and hypoosmolar urinary output developing after several days of treatment (11). In the present study, 100 mg/kg of gentamicin administered i.p. for 7 days produced kidney injury, which was evidenced by increased blood urea, serum creatinine, and serum potassium, as well as decreased urine output (P < 0.05), urine creatinine, serum protein, and sodium levels. A study by Hussain et al. in 2012 also showed elevated levels of urea and creatinine in plasma as well as urine, severe proximal tubular necrosis, and renal failure with a 100 mg/kg dose of gentamicin, which is similar to our results (22). Some studies suggest that aminoglycoside antibiotics are able to stimulate the formation of ROS, such as superoxide anions, as well as GSH and membrane lipid peroxidation, which may be directly responsible for its injurious effect on the kidneys (7). Superoxide (O2-), hydrogen peroxide (H2O2), and hydroxyl radicals increase with gentamicin treatment. H2O2 and O2- induce mesangial cell contraction, alter the filtration surface area in the glomeruli, and modify the ultrafiltration coefficient, ultimately decreasing urine output and thus GFR (11, 12). Oxidative parameters, such as SOD and GSH levels, were reduced with gentamicin treatment in our study, which indicates the generation of oxidative stress in accordance with the abovementioned mechanism, but they did not reach statistical significance. However, MDA (an end-product of lipid peroxidation) was not increased in our study. Gentamicin caused marked damage to the kidney architecture, which was confirmed by histopathological parameters, including necrosis, epithelial cell desquamation, and cast formation. Some flat epithelial degeneration and edema were also noted.
Several agents that scavenge or interfere with the production of ROS have been successfully used to ameliorate gentamicin-induced nephropathy (23). CS is a traditional herbal plant used as a cordial, tonic, emmenagogue, vermifuge, diuretic, diaphoretic, and desiccant (13-15). In our study, administration of 150 mg/kg and 250 mg/kg doses of hydroalcoholic CS extract as a preventive and curative therapy restored urinary parameters, such as urine output and creatinine, while also inhibiting increases in biochemical parameters, such as blood urea, serum creatinine, serum protein, serum sodium, and serum potassium. In the CS-treated groups, antioxidant parameters such as SOD and GSH levels were increased, while MDA was decreased, though not significantly, indicating that it may have antioxidant properties. The CS-treated group showed no or minimal necrosis, epithelial cell desquamation, or cast formation, while maintaining normal renal architecture, demonstrating the nephroprotective action offered by CS. However, flat epithelial degeneration and edema were reversed to a lesser extent. Thus, gross histopathological scrutiny demonstrated a protective effect of this extract on the structural anatomy of the kidney.
We used 100 mg/kg of α-lipoic acid, a naturally occurring dithiol compound, as an active control drug. This has been described as a potent biological antioxidant (24). In the present study, α-lipoic acid resulted in restoration of all urinary and biochemical parameters, markers of oxidative stress, and histopathological parameters in both the preventive and the curative groups.
In vitro evaluation of CS was found to have a total phenolic content of 66.7 ± 23.1 mg/g, a total flavonoid content of 73.3 ± 11.5 mg/g, and an IC50 value for DPPH scavenging activity of 7.9 ± 0.4 μg/mL (16). The phenolic compounds present in CS are effective hydrogen donors, making them good antioxidants (25). The high antioxidant potential of flavonoids is attributable to their capacity to scavenge harmful ROS and other free radicals that originate from various cellular activities and lead to oxidative stress (26). CS roots contain compounds such as patchoulanol and isopatchoulenone as major components of its essential oil (27). The phenol, flavonoid content, and DPPH scavenging activity, as well as the major essential oils present in CS, all contribute to its antioxidant properties, which may be the reason for its nephroprotective activity in the present study. A study by Kalim et al. in 2010 also identified DPPH, hydroxyl ion, and superoxide scavenging properties (28).
The results of the present study show possible nephroprotection offered by hydroalcoholic extract of CS at 150 mg/kg and 250 mg/kg doses for the prevention of AKI, as well as for the treatment of established kidney injuries. Further studies with higher doses and larger sample sizes should be performed to confirm the nephroprotective effects of this plant and to evaluate the applicability of CS in humans.
5.1. Conclusions
Hydroalcoholic extract of C. scariosus Linn. in doses of 150 and 250 mg/kg was found to be effective for prevention as well as for treatment of gentamicin-induced AKI, which might be attributed to its antioxidant properties.
Acknowledgements
References
-
1.
Nissenson AR. Acute renal failure: definition and pathogenesis. Kidney Int Suppl. 1998;66:S7-10. [PubMed ID: 9573567].
-
2.
Hou SH, Bushinsky DA, Wish JB, Cohen JJ, Harrington JT. Hospital-acquired renal insufficiency: a prospective study. Am J Med. 1983;74(2):243-8. [PubMed ID: 6824004].
-
3.
de Mendonca A, Vincent JL, Suter PM, Moreno R, Dearden NM, Antonelli M, et al. Acute renal failure in the ICU: risk factors and outcome evaluated by the SOFA score. Intensive Care Med. 2000;26(7):915-21. [PubMed ID: 10990106].
-
4.
Uchino S. The epidemiology of acute renal failure in the world. Curr Opin Crit Care. 2006;12(6):538-43. [PubMed ID: 17077683]. https://doi.org/10.1097/01.ccx.0000247448.94252.5a.
-
5.
Hilton R. Acute renal failure. BMJ. 2006;333(7572):786-90. [PubMed ID: 17038736]. https://doi.org/10.1136/bmj.38975.657639.AE.
-
6.
Homes HD, Weinberg JM. Toxic nephropathies. In: Brenner BM, Rector F, editors. Kidney. Philadelphia: Saunders Co; 1986. p. 1491-533.
-
7.
Kadkhodaee M, Khastar H, Faghihi M, Ghaznavi R, Zahmatkesh M. Effects of co-supplementation of vitamins E and C on gentamicin-induced nephrotoxicity in rat. Exp Physiol. 2005;90(4):571-6. [PubMed ID: 15769882]. https://doi.org/10.1113/expphysiol.2004.029728.
-
8.
Cuzzocrea S, Mazzon E, Dugo L, Serraino I, Di Paola R, Britti D, et al. A role for superoxide in gentamicin-mediated nephropathy in rats. Eur J Pharmacol. 2002;450(1):67-76. [PubMed ID: 12176111].
-
9.
Sandhu JS, Sehgal A, Gupta O, Singh A. Aminoglycoside nephrotoxicity revisited. J Indian Acad Clin Med. 2007;8(4):331-3.
-
10.
Watanabe A, Nagai J, Adachi Y, Katsube T, Kitahara Y, Murakami T, et al. Targeted prevention of renal accumulation and toxicity of gentamicin by aminoglycoside binding receptor antagonists. J Control Release. 2004;95(3):423-33. [PubMed ID: 15023454]. https://doi.org/10.1016/j.jconrel.2003.12.005.
-
11.
Ali BH, Bashir AK. Effect of superoxide dismutase treatment on gentamicin nephrotoxicity in rats. Gen Pharmacol. 1996;27(2):349-53. [PubMed ID: 8919655].
-
12.
Anjaneyulu M, Chopra K. Effect of irbesartan on the antioxidant defence system and nitric oxide release in diabetic rat kidney. Am J Nephrol. 2004;24(5):488-96. [PubMed ID: 15353911]. https://doi.org/10.1159/000080722.
-
13.
Kirtikar KR, Basu BD. Indian medicinal plants. Indian Medicinal Plants. 1918.
-
14.
Watt G. A dictionary of the economic products of India. Cambridge University Press; 2014.
-
15.
Said HM. Diseases of the liver: Greco-Arab concepts. 1982.
-
16.
Chawda HM, Mandavia DR, Parmar PH, Baxi SN, Tripathi CR. Hypolipidemic activity of a hydroalcoholic extract of Cyperus scariosus Linn. root in guinea pigs fed with a high cholesterol diet. Chin J Nat Med. 2014;12(11):819-26. [PubMed ID: 25480512]. https://doi.org/10.1016/S1875-5364(14)60123-0.
-
17.
Misra HP, Fridovich I. The role of superoxide anion in the autoxidation of epinephrine and a simple assay for superoxide dismutase. J Biol Chem. 1972;247(10):3170-5. [PubMed ID: 4623845].
-
18.
Morton JP, Maclaren DP, Cable NT, Campbell IT, Evans L, Bongers T, et al. Elevated core and muscle temperature to levels comparable to exercise do not increase heat shock protein content of skeletal muscle of physically active men. Acta Physiol (Oxf). 2007;190(4):319-27. [PubMed ID: 17488245]. https://doi.org/10.1111/j.1748-1716.2007.01711.x.
-
19.
Slater TF, Sawyer BC. The stimulatory effects of carbon tetrachloride and other halogenoalkanes on peroxidative reactions in rat liver fractions in vitro. General features of the systems used. Biochem J. 1971;123(5):805-14. [PubMed ID: 4399399].
-
20.
Suzuki S, Takamura S, Yoshida J, Shinzawa Y, Niwa O, Tamatani R. Comparison of gentamicin nephrotoxicity between rats and mice. Comp Biochem Physiol C Pharmacol Toxicol Endocrinol. 1995;112(1):15-28. [PubMed ID: 8564786].
-
21.
Servais H, Ortiz A, Devuyst O, Denamur S, Tulkens PM, Mingeot-Leclercq MP. Renal cell apoptosis induced by nephrotoxic drugs: cellular and molecular mechanisms and potential approaches to modulation. Apoptosis. 2008;13(1):11-32. [PubMed ID: 17968659]. https://doi.org/10.1007/s10495-007-0151-z.
-
22.
Hussain T, Gupta RK, Sweety K, Eswaran B, Vijayakumar M, Rao CV. Nephroprotective activity of Solanum xanthocarpum fruit extract against gentamicin-induced nephrotoxicity and renal dysfunction in experimental rodents. Asian Pac J Trop Med. 2012;5(9):686-91. [PubMed ID: 22805718]. https://doi.org/10.1016/S1995-7645(12)60107-2.
-
23.
Reddy DRCS, Kumar GS, Swamy BMV, Kumar KP. Protective effect of Cissampelos pareira linn. Extract on gentamicin-induced nephrotoxicity and oxidative damage in rats. Pharm J. 2014;6(4):59.
-
24.
Goraca A, Huk-Kolega H, Piechota A, Kleniewska P, Ciejka E, Skibska B. Lipoic acid–biological activity and therapeutic potential. Pharmacol Rep. 2011;63(4):849-58.
-
25.
Rice-Evans CA, Miller NJ, Bolwell PG, Bramley PM, Pridham JB. The relative antioxidant activities of plant-derived polyphenolic flavonoids. Free Radic Res. 1995;22(4):375-83. [PubMed ID: 7633567].
-
26.
Bors W, Heller W, Michel C, Saran M. Flavonoids as antioxidants: determination of radical-scavenging efficiencies. Methods Enzymol. 1990;186:343-55. [PubMed ID: 2172711].
-
27.
Chopra RN, Nayar SL, Chopra IC. Glossary of Indian medicinal plants. New Delhi; 1956. Available from: http://kbd.kew.org/kbd/detailedresult.do?id=17974.
-
28.
Kalim MD, Bhattacharyya D, Banerjee A, Chattopadhyay S. Oxidative DNA damage preventive activity and antioxidant potential of plants used in Unani system of medicine. BMC Complement Altern Med. 2010;10:77. [PubMed ID: 21159207]. https://doi.org/10.1186/1472-6882-10-77.