Abstract
Background:
Sea urchins contain high values of antioxidant substances such as polyphenols.Objectives:
In the present study, the antioxidant activities, α-amylase inhibition, anti-inflammatory activity and the amounts of phenols and flavonoids of purple sea urchin Echinometra mathaei tissues obtained from Persian Gulf were examined.Methods:
In December 2012, the purple sea urchin E. mathaei used for this study was harvested in the intertidal zone of Qeshm Island, Hormozgan Province, Iran. In the experimental design, extract isolation of different tissues of sea urchin such as spines, shells, gonads and Aristotle’s lantern was performed with the application of n-hexane, ethyl acetate and methanol solvents. Antioxidant properties of the samples were evaluated using different antioxidant tests such as total antioxidant capacity (TAC) and reducing power. The anti-diabetic and anti-inflammatory effects of samples were examined by inhibition of α-amylase and retardation of serum albumin denaturation, respectively.Results:
With respect to antioxidant properties, the methanol extract of shell containing 40 μg/mL concentration possessed the maximum reducing power (OD = 0.475 ± 0.0049 and, ethyl acetate extract of Aristotle’s lantern at 1 mg/mL concentration presented the highest TAC (OD = 0.439 ± 0.0018). The n-hexane extract of gonad at a concentration of 1 mg/mL revealed the highest α-amylase inhibition (84.7 ± 2.92%). The ethyl acetate extract of Aristotle’s lantern at 20 μg/mL concentration possessed the highest anti-inflammatory property (69.78 ± 1.61 μg/mL). In biochemical analysis, the methanol extract of spine and ethyl acetate extract of Aristotle’ lantern had the highest phenolic (0.33 ± 0.017 mg/g) and flavonoid compounds (24.6 ± 0.72 mg/g), respectively.Conclusions:
The obtained results revealed that sea urchin extracts could be used in pharmaceutical industries. Furthermore, its application in food industries as a supplementary food can be of great value.Keywords
Antioxidant Effects Sea Urchins Phenolic Compounds Anti-Inflammatory Effects Inhibition of α-Amylase
1. Background
Sea urchins belong to the group of echinoderm marine invertebrates pertaining to sea star, brittle sea star and sea cucumbers. They have orbicular bodies coated with a strict shell and thoroughly covered with many sharp spines (1). The edible part of the sea urchin, i.e. gonad, is half-moon shaped, yellow-orange in color and constitutes 10% of the total weight (2). Gonad has the highest levels of free amino acids, i.e. up to 10/100 g of proteins (3). The taste of sea urchin roe is similar to that of caviar and oysters. Furthermore, it can be consumed either as raw or fermented products; that is why sea urchins are usually consumed by Japanese, French and also South and North Americans (1). The mentioned species is a severe grazer on seaweeds, especially on brown algae such as Laminaria species and is thus expected to accumulate a number of antioxidant compounds such as carotenoids and polyphenols (4).
These natural antioxidants capable of scavenging the free radicals play a significant role in prevention of vascular diseases and some types of cancer (5). Free radicals, which have one or more unpaired electrons in their outer orbital, consist of superoxide anion (O2•-), alkoxy (RO•), peroxyl (ROO•), hydroxyl (HO•) and nitric oxide. Oxygen-centred free radicals are, sometimes known as reactive oxygen species (ROS) (6), which attack macromolecules such as lipids, proteins, enzymes, DNA and RNA (7). Scavenging of ROS leads to a reduction of oxidative stress of organisms and prevents a number of chronic and degenerative diseases such as aging, stroke, diabetes mellitus, cancer, cardiovascular and neurodegenerative complications (8). Hence, the antioxidants capable of scavenging ROS are expected to improve these disorders (9).
Examining the evolution of human diets reveals that in the Paleolithic age humans consumed a lot of antioxidants derived from the plants (10). Over the past decades, human nutrition in industrial countries had changed towards fast-food nutrition, which is rich in lipids and poor in antioxidant compounds. Increasing of pro-oxidant compounds appears to lead to increased risk of physiological disturbances. According to the nutritionists and physicians’ health recommendations, people must increase their intakes of fruits and vegetables to provide their daily dietary nutritional antioxidants. In this regard, seafood can be considered as part of a healthy diet. Seafood species such as mussels, scallops, crabs and sea urchins are seldom considered as sources of antioxidants (4). A key difference between dietary supply and physiological needs may be in the provided antioxidant nutrients. Hence, consumption of antioxidants is essential as humans are exposed to a diverse array of oxidants (10).
2. Objectives
In the present study, the antioxidant activities, α-amylase inhibition, anti-inflammatory property and total phenols and flavonoids of purple sea urchin Echinometra mathaei tissues were evaluated.
3. Methods
3.1. Materials
Butylated hydroxyltoluene (BHT), α-amylase and bovine serum albumin (BSA) were purchased from Sigma-Aldrich. All the other reagents were obtained from Merck Chemical Co. and all the chemicals used in the experiments were of analytical grade.
3.2. Collection of Sea Urchin and Preparation of Extracts
In December 2012, the purple sea urchin E. mathaei was harvested in the intertidal zone of Qeshm Island, Hormozgan province, Iran. Samples were collected and immediately transported to the laboratory and gently rinsed with filtered seawater. After dissection phase, the spines, shells, gonads and Aristotle’s lantern (mouth parts) were collected, freeze-dried in dark and then ground into a powder.
Based on the increasing polarity, dried tissues of E. mathaei were immersed in n-hexane, ethyl acetate and methanol (1:3 w/v), respectively. Extracts were obtained by cold percolation and concentrated by a rotary evaporator at 40°C. The concentrated extracts were dissolved in DMSO 99% and stored at (-30°C) in the dark.
3.3. Determination of Antioxidant Activities
3.3.1. Determination of Reducing Power
The reducing power was determined according to the procedure described by Oyaizu. The sample solution (0.4 mL), at different concentration levels (1.25, 2.5, 5, 10, 20 and 40 μg/mL), was mixed in one mL phosphate buffer (0.2 M, pH 6.6) and one mL potassium ferricyanide (1% w/v). The mixture was incubated at 50°C for 20 minutes. One mL trichloroacetic acid (TCA, 10% w/v) was added and mixed with 1 mL distilled water and 0.2 mL ferric chloride (0.1% w/v). Absorbance of this mixture was recorded by a spectrophotometer at a wavelength of 700 nm. The analyses were performed in triplicate and ascorbic acid was used as a standard (11).
3.3.2. Determination of Total Antioxidant Capacity (TAC)
Total antioxidant activities of the extracts were measured according to the method of Mitsuda et al. Sulfuric acid 0.6 M (7.45 mL), sodium sulphate (0.99 g) and ammonium molybdate (1.23 g) were mixed together in 250 mL distilled water and labelled as a total antioxidant capacity (TAC) solution. One-tenth mL of the extracts (50, 100, 250, 500 and 1000 μg/mL) was dissolved in 1 mL of TAC solution. After 15 minutes of incubation the absorbance was read at 695 nm. Ascorbic acid was used as a positive control (12).
3.3.3. Determination of Total Phenolic Content
The amounts of the phenolic compounds in the prepared extracts were determined according to the method described by Fu et al. The sample (0.15 mL, 10 mg/mL) was mixed with 0.75 mL Folin-Ciocalteu (diluted 1:10 with distilled water). After 3 minutes, 0.6 mL of saturated sodium carbonate (7.5% w/v) was added to the mixture. After mixing, the mixture was kept in the dark for 60 minutes, after which the absorbance was read at 765 nm (8). Different concentrations (0.0003 - 0.0024 mg/mL) of gallic acid were used as the standard curve. Total content of phenolic compounds in the extracts was calculated by the following formula:
C = c .v/m'
Where C is the total content of phenolic compounds (mg/g), c is the concentration of gallic acid specified by the standard curve (mg/mL), v is the volume of the extract (mL), and m′ is the weight of pure tissue (g).
3.3.4. Determination of Total Flavonoid Content
Total flavonoids were determined according to the method of Zou et al. A diluted sample (0.5 mL, 1 mg/mL) was mixed with 2 mL of distilled water. After 3 minutes, 0.15 mL of 5% NaNO2 solution and 0.15 mL of 10% AlCl3 solution were added and allowed to stand for 6 minutes. Then, 2 mL of 4% NaOH solution was added to the mixture. Immediately, 0.2 mL of water was added until the final volume became 5 mL. Next, the mixture was allowed to stand for another 15 minutes, after which the absorbance was read at 510 nm (6). Different concentrations (12/5, 25, 50, and 100 µg/mL) of BHT were used to draw the standard curve.
3.4. Determination of α-Amylase Inhibition
The inhibition of α-amylase was carried out with some modifications of Ademiluyi et al.’s method. In this colorimetric method, starch and 3, 5-dinitrosalicylic acid (DNSA) were used as the substrate and reagent, respectively. The standard curve of maltose, as a product, enables researchers to calculate the amounts of maltose released during α-amylase activity. The enzyme solution (4 unit/mL) was prepared by mixing 0.001 g of porcine pancreatic α-amylase (EC 3.2.1.1) in sodium phosphate buffer (pH = 6.9, 20 mM), which had 6.7 mM sodium chloride. The extracts were dissolved in DMSO and the extracted starch solution was mixed. The reagent (DNSA) solution possessed 96 mM 3, 5-dinitrosalicylic acid (20 mL) and 5.31 M potassium tartrate sodium in 2 M sodium hydroxide (8 mL) and deionized water (12 mL).
0.56 mL of the extracted-starch solution and 0.04 mL enzyme were mixed in a tube and incubated at 37°C for 15 minutes. Next, 0.6 mL of the color reagent (DNSA) was added, and the test tube was placed in the water bath (85°C) for 15 minutes, after which the absorbance of the samples was determined at 540 nm (13). Additional blanks were prepared to correct the background absorbance. The control demonstrated 100% enzyme activity and substituted extracts with DMSO. One unit of the enzyme activity was defined as the amount of required enzyme to release one micromole of maltose from starch per minute. Acarbose was used as a positive control. All tests were performed in triplicate. The inhibition percentage of α-amylase was assessed by the following formula:
I α-amylase% = ((∆AControl - ∆Asample) / ∆Acontrol) × 100
∆A control = Atest - Ablank
∆Asample = Atest - Ablank
3.5. Determination of Anti-Inflammatory Effect by Inhibition of Protein Denaturation
The test was performed by modifying the method described by Mizushima et al. The reaction mixture contained 0.05 mL of different extracts at various concentrations (1.25, 2.5, 5, 10 and 20 μg/mL) and 0.45 mL of the bovine serum albumin (BSA, 0.1%, pH = 6.3). The mixture was incubated at 37°C for 20 minutes and then incubated at 57°C for 20 minutes. After cooling the mixture, the turbidity was measured by spectrophotometer at 660 nm (14). The percentage inhibition of protein denaturation was calculated as follows:
The percentage inhibition = ((A- As) /A) × 100
Where A is the absorbance of the control, and As is the absorbance of reaction solution. Aspirin was used as a positive control.
3.6. Statistical Analysis
The data was expressed as the mean ± SD of three replicates. Analysis was performed using SPSS 19 and Excel 2010. One-way analysis of variance (ANOVA) and Duncan’s new multiple-rang test were used to determine the possible differences amongst the means. P values ≤ 0.05 were considered as significant differences.
4. Results
Reducing power of different extracts of sea urchin is presented in Figure 1A N-hexane extract of gonad, methanol extract of shell and ethyl acetate extracts of spine and Aristotle’s lantern revealed the highest reducing power (Figure 1A). Significant differences were observed at P < 0.05.
A, Extracts of Echinometra matheai, Which Possessed More Reducing Power, Compared With Vit C as a Standard; B, Extracts of Echinometra matheai, Which Possessed More Total Antioxidant Capacities, Compared With Vit C as a Standard
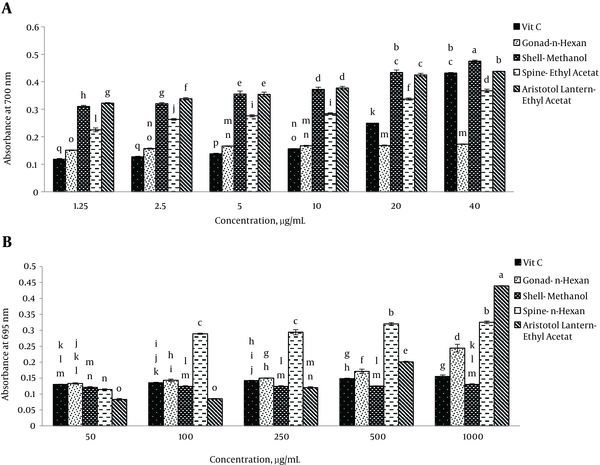
In comparison with ascorbic acid as a standard, the mentioned extracts could reduce high concentrations of ferric ions. It was observed that methanol extract of the shell at 40 μg/mL possessed the maximum reducing power, which was more than that of ascorbic acid as a standard. Ascorbic acid had the minimum reducing power at 2.5 and 1.25 μg/mL. Significant differences were observed at P < 0.05.
Total antioxidant capacities of different extracts of sea urchin are provided in Figure 1B. Generally, identical to ascorbic acid as a standard, the presented extracts had high total antioxidant capacities. N-hexane extracts of gonad and spine, methanol extract of shell and ethyl acetate extract of Aristotle’s lantern revealed the highest total antioxidant capacities (Figure 1B). Significant differences were observed at P < 0.05.
Ethyl acetate extract of Aristotle’s lantern at 1 mg/mL had more total antioxidant capacity than ascorbic acid as a standard (Figure 1B). Ethyl acetate extract of Aristotle’s lantern showed the lowest total antioxidant capacity. Significant differences were observed at P < 0.05. It can be stated that total antioxidant capacity depends on the concentrations of sea urchin extracts.
Total phenolic contents of sea urchin were determined using the Folin-Ciocalteu reagent, which relying on the transfer of electrons from phenolic compounds to the Folin-Ciocalteu reagent in the alkaline medium. This technique is an applicable method, which is used widely (8). Table 1 presents total phenolic contents, which varied from 0.0044 ± 0.0003 mg/g (methanol extract of gonad) to 0.3256 ± 0.0171 mg/g (methanol extract of spine). As Table 1 presents total flavonoid contents varied from 0.47 ± 0.27 mg/g (ethyl acetate extract of shell) to 24.62 ± 0.72 mg/g (ethyl acetate extract of Aristotle’s lantern).
Sample | Phenolic Content, mg GAE/g | Flavonoid Content, mg BHT/g |
---|---|---|
Methanol extract of gonad | 0.0044 ± 0.0003 | - |
Methanol extract of shell | 0.1781 ± 0.017 | - |
Methanol extract of spine | 0.3256 ± 0.017 | - |
Methanol extract of aristotles lantern | 0.0227 ± 0.001 | - |
Ethyl acetate extract of gonad | - | 3.9 ± 0.41 |
Ethyl acetate extract of shell | - | 0.47 ± 0.27 |
Ethyl acetate extract of spine | - | 4.81 ± 0.32 |
Ethyl acetate extract of aristotles lantern | - | 24.6 ± 0.72 |
In this study, porcine pancreatic α-amylase was used to evaluate the anti-diabetic properties of extracts at different concentrations. A-amylase inhibition at different concentrations of sea urchin extracts (1 and 2 mg/mL) was determined. N-hexane extract of the gonad (84.7 ± 2.9), methanol extract of the shell (74.7 ± 0.64) and n-hexane extract of the spine showed the maximum inhibition of α-amylase, respectively (Figure 2). Significant differences were observed at P < 0.05.
The results showed that, n-hexane extract of the gonad at 1 mg/mL substrate possessed the highest α-amylase inhibition. At 2 mg/mL concentration of the substrate, ethyl acetate extract of Aristotle’s lantern revealed the lowest α-amylase inhibition (Figure 2). Significant differences were observed at P < 0.05.
A-Amylase Inhibition of the Echinometra matheai Extracts
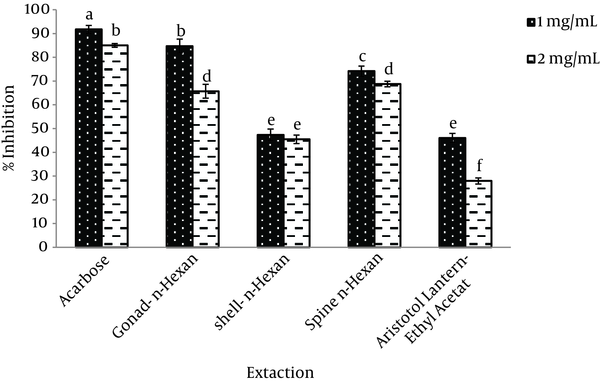
Different extracts of sea urchin tissues exhibited significant anti-inflammatory properties at different concentrations. Generally, these extracts had similar anti-inflammatory properties like that of aspirin. The lower IC50 indicated the higher anti-inflammatory ability (Table 2). The highest anti-inflammatory properties were detected in the methanol extract of gonad, ethyl acetate extract of shell and Aristotle’s lantern as well as n-hexane extracts of spine. Methanol extract of gonad at 1.25 μg/mL concentration showed inflammatory effects identical to that of aspirin (Figure 3).
IC50 Values (μg/mL) of Sea Urchin Extracts in Proteinase Inhibition Assay
Samples | IC50 of Proteinase Inhibition μg/mL |
---|---|
Aspirin | 2.25 ± 0.14a |
Methanol extract of gonad | 3.06 ± 0.25b |
Methanol extract of shell | NA |
Methanol extract of spine | NA |
Methanol extract of aristotles lantern | NA |
Ethyl acetate extract of gonad | 4.56 ± 0.12b |
Ethyl acetate extract of shell | NA |
Ethyl acetate extract of spine | NA |
Ethyl acetate extract of aristotles lantern | 3.35 ± 0.06c |
n-hexane extract of gonad | 4.71 ± 0.21c |
n-hexane extract of shell | 2.48 ± 0.09a |
n-hexane extract of spine | 2.70 ± 0.15a,b |
n-hexane extract of aristotles lantern | 5.28 ± 0.45c |
Anti-Inflammatory Effects of the Echinometra matheai Extracts
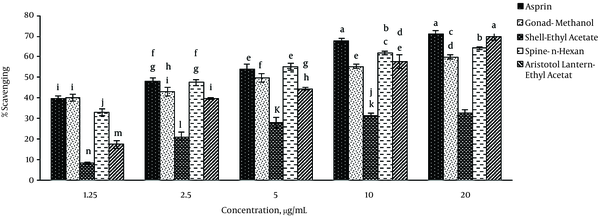
The ethyl acetate extract of Aristotle’s lantern at 20 μg/mL concentration possessed the highest anti-inflammatory activity similar to that of aspirin as a standard (P < 0.05). Ethyl acetate extract of the shell revealed the lowest anti-inflammatory effect. Anti-inflammatory potential was found to increase by increasing the concentrations of sea urchin extracts, regardless of the nature of preparation.
5. Discussion
N-hexane extract of gonad, methanol extract of shell and ethyl acetate extracts of spine and Aristotle’s lantern had the highest reducing power. In the biochemical analysis, the methanol extract of spine and ethyl acetate extract of Aristotle lantern had the highest phenolic and flavonoid compounds, respectively. The n-hexane extract of gonad at 1 mg/mL showed the highest α-amylase inhibition. The ethyl acetate extract of Aristotle lantern at 20 μg/mL possessed the highest anti-inflammatory property.
In the present study, the antioxidant properties of different extracts of sea urchin were evaluated with consideration of reducing power, total antioxidant capacity and determination of phenolic and flavonoid compounds. The reducing power assay was used to evaluate the reduction of Fe+3 by sea urchin extracts. Due to its easy application, this method is widely used for appraisal of antioxidant capacity (8). Increasing the absorbance of the reaction mixture indicates an increase of the reducing power. In the reducing power assay, the yellow color of the test solution changes to several shades of green and blue, depending on the reducing power of each extract (5). The results of the previous studies (5, 8) showed that all the tested extracts could reduce iron (III). Furthermore, they reported that reducing power of the extracts depended on their concentrations. Hence, the reducing power could mainly be imputed to the bioactive compounds associated with antioxidant activity (5). Our previous study on the shell and spine pigments of purple sea urchin E. mathaei showed that reducing power of both pigments extractions (shell and spine) increased as the concentrations increased (15). Results from previous studies have shown that dicholoromethan extract of sea cucumber Holoturia leucospilota increases absorbance with increasing dose dependent manner (16).
Naturally, there are a number of antioxidant compounds that act as radical scavengers and lipid peroxidation inhibitors. Usage of natural antioxidants such ad polyphenols in general and flavonoid in particular can be considered as relevant components to reduce the risk of developing coronary heart diseases, neurological disorders and certain types of cancers (17). The obtained results showed that total phenols of purple sea urchin E. mathaei tissue extracts are similar to those of gonads (53 mg GAE/100g) and digestive tracts (123 mg GAE/100 g) of green sea urchin centrotus droebachiensis and shells (1.5 mg GAE/g) of red-brown sea urchin Psammechinus miliaris (18). Phenolic compounds may account for antioxidant properties such as reducing power (5), which are caused by hydroxyl groups of these molecules (19, 20).
Some antioxidant compounds could inhibit α-amylase. With consideration of the mentioned point, the inhibition of α-amylase was determined by different extracts of sea urchin in the present study. As the review of literature reveals, it is the first time that the inhibition of α-amylase by these extracts has been reported. The results of the present study demonstrate that delayed starch hydrolysis by inhibition of α-amylase leads to the decline of these extracts in the glucose concentration (21).
The observed mechanism of sea urchin extract exhibited its hypoglycaemic effect, which leads to decrease of postprandial blood glucose level. A-amylase inhibition may depend on the concentrations of extracts and types of bioactive compounds in these extracts (22).
Moreover, it is reported that diets rich of antioxidant compounds decrease the incidence of oxidant-linked diseases such as diabetes (23). Various studies have demonstrated that extracts with strong antioxidant activity, which inhibit free radicals such as superoxide and hydroxyl can be effective in inhibition of α-amylase (24-26).
Over the recent years, attention has mainly been devoted to specify antioxidant and anti-inflammatory compounds due to their potential usage in treatment of several chronic and infectious diseases. Epidemiologically and tentative studies have implicated oxidative cellular damage arising as a result of an imbalance between free radicals generation and scavenging systems, which can cause cardiovascular diseases, cancer and aging (27). A number of studies reported the anti-inflammatory potentials of plants and vegetables (14, 27, 28); however, as the literature review reveals, it is the first time that the anti-inflammatory effect of sea urchin has been reported.
Recent studies have been demonstrated that many flavonoids and polyphenols remarkably contributed to the anti-inflammatory properties of many plants (28). Hence, the results of this study showed that flavonoids and polyphenols of sea urchin may possess anti-inflammatory activities.
In this study, the additive effects of antioxidant properties of gonad methanol extract, ethyl acetate extracts of shell and Aristotle’s lantern and n-hexane extract of spine were observed.
The mentioned results are indicative of new sources of antioxidants, anti-diabetic and anti-inflammatory compounds obtained from the marine. In conclusion, the present study demonstrates that tissue extracts of sea urchin possess different bioactive compounds. From the researchers’ perspective, sea urchin extract can be used as a supplement in food and pharmaceutical industries.
Acknowledgements
References
-
1.
Amarowicz R, Synowiecki J, Shahidi F. Chemical composition of shells from red (Strongylocentrotus franciscanus) and green (Strongylocentrotus droebachiensis) sea urchin. Food Chem. 2012;133(3):822-6.
-
2.
Zhou DY, Qin L, Zhu BW, Wang XD, Tan H, Yang JF, et al. Extraction and antioxidant property of polyhydroxylated naphthoquinone pigments from spines of purple sea urchin Strongylocentrotus nudus. Food Chem. 2011;129(4):1591-7.
-
3.
Amarowicz R, Synowiecki J, Shahidi F. Sephadex LH-20 separation of pigments from shells of red sea urchin (Strongylocentrotus franciscanus). Food Chem. 1994;51(2):227-9.
-
4.
Mamelona J, Pelletier E, Girard-Lalancette K, Legault J, Karboune S, Kermasha S. Antioxidants in digestive tracts and gonads of green urchin (Strongylocentrotus droebachiensis). J Food Compos Analysis. 2011;24(2):179-83.
-
5.
Ferreira ICFR, Baptista P, Vilas-Boas M, Barros L. Free-radical scavenging capacity and reducing power of wild edible mushrooms from northeast Portugal: Individual cap and stipe activity. Food Chem. 2007;100(4):1511-6.
-
6.
Zou Y, Lu Y, Wei D. Antioxidant activity of a flavonoid-rich extract of Hypericum perforatum L. in vitro. J Agric Food Chem. 2004;52(16):5032-9. [PubMed ID: 15291471]. https://doi.org/10.1021/jf049571r.
-
7.
Duan XJ, Zhang WW, Li XM, Wang BG. Evaluation of antioxidant property of extract and fractions obtained from a red alga, Polysiphonia urceolata. Food Chem. 2006;95(1):37-43.
-
8.
Fu L, Xu BT, Xu XR, Gan RY, Zhang Y, Xia EQ, et al. Antioxidant capacities and total phenolic contents of 62 fruits. Food Chem. 2011;129(2):345-50.
-
9.
Shankarlal S, Prabu K, Natarajan E. Antimicrobial and antioxidant activity of Purple sea Urchin Shell (Salmacis virgulata L. Agassiz and Desor 1846). Am Eur J Sci Res. 2011;6(3):178-81.
-
10.
Ratnam DV, Ankola DD, Bhardwaj V, Sahana DK, Kumar MN. Role of antioxidants in prophylaxis and therapy: A pharmaceutical perspective. J Control Release. 2006;113(3):189-207. [PubMed ID: 16790290]. https://doi.org/10.1016/j.jconrel.2006.04.015.
-
11.
Oyaizu M. Studies on products of browning reaction--antioxidative activities of products of browning reaction prepared from glucosamine. Japan J Nutr. 1986.
-
12.
Mistuda H, Yuasumoto K, Iwami K. Antioxidation action of indole compounds during the autoxidation of linoleic acid. EIYO TO SHOKURYO. 1996;19:210-4.
-
13.
Ademiluyi AO, Oboh G, Boligon AA, Athayde ML. Effect of fermented soybean condiment supplemented diet on α-amylase and α-glucosidase activities in Streptozotocin-induced diabetic rats. J Function Foods. 2014;9:1-9.
-
14.
Mizushima Y, Kobayashi M. Interaction of anti-inflammatory drugs with serum proteins, especially with some biologically active proteins. J Pharm Pharmacol. 1968;20(3):169-73. [PubMed ID: 4385045].
-
15.
Soleimani S, Yousefzadi M, Rezadoost H, Bioki N. Identification and antioxidant of polyhydroxylated naphthoquinone pigments from sea urchin pigments of Echinometra mathaei. Med Chem Res. 2016;25(7):1-8.
-
16.
Soltani M, Baharara J. Antioxidant and antiprolifereative capacity of dichloromethane extract of holoturia leucospilota sea cucumber. Int J Data Min Bioinform. 2014:1-9.
-
17.
Ashraf MA, Maah M, Yusoff I. Estimation of antioxidant phytochemicals in four different varieties of durian (Durio zibethinus murray) fruit. Middle East J Sci Res. 2010;6(5):465-71.
-
18.
Powell C, Hughes AD, Kelly MS, Conner S, McDougall GJ. Extraction and identification of antioxidant polyhydroxynaphthoquinone pigments from the sea urchin, Psammechinus miliaris. LWT Food Sci Technol. 2014;59(1):455-60. https://doi.org/10.1016/j.lwt.2014.05.016.
-
19.
Kuwahara R, Hatate H, Chikami A, Murata H, Kijidani Y. Quantitative separation of antioxidant pigments in purple sea urchin shells using a reversed-phase high performance liquid chromatography. LWT Food Sci Technol. 2010;43(8):1185-90. https://doi.org/10.1016/j.lwt.2010.03.005.
-
20.
Kuwahara R, Hatate H, Yuki T, Murata H, Tanaka R, Hama Y. Antioxidant property of polyhydroxylated naphthoquinone pigments from shells of purple sea urchin Anthocidaris crassispina. LWT Food Sci Technol. 2009;42(7):1296-300. https://doi.org/10.1016/j.lwt.2009.02.020.
-
21.
Dewanjee S, Maiti A, Sahu R, Dua TK, Mandal V. Effective Control of Type 2 Diabetes through Antioxidant Defense by Edible Fruits of Diospyros peregrina. Evid Based Complement Alternat Med. 2011;2011:675397. [PubMed ID: 19584081]. https://doi.org/10.1093/ecam/nep080.
-
22.
Ponnusamy S, Ravindran R, Zinjarde S, Bhargava S, Ravi Kumar A. Evaluation of traditional Indian antidiabetic medicinal plants for human pancreatic amylase inhibitory effect in vitro. Evid Based Complement Alternat Med. 2011;2011. [PubMed ID: 20953430]. https://doi.org/10.1155/2011/515647.
-
23.
Jo S, Ka E, Lee H, Apostolidis E, Jang HD, Kwon YI. Comparison of antioxidant potential and rat intestinal a-glucosidases inhibitory activities of quercetin, rutin, and isoquercetin. Int J Appl Res Nat Prod. 2009;2(4):52-60.
-
24.
Jakus V. The role of free radicals, oxidative stress and antioxidant systems in diabetic vascular disease. Bratislavske lekarske listy. 2000;101(10):541-51.
-
25.
Li W, Dai RJ, Yu YH, Li L, Wu CM, Luan WW, et al. Antihyperglycemic effect of Cephalotaxus sinensis leaves and GLUT-4 translocation facilitating activity of its flavonoid constituents. Biol Pharm Bull. 2007;30(6):1123-9. [PubMed ID: 17541165].
-
26.
Ojewole JA. Hypoglycaemic effect of Clausena anisata (Willd) Hook methanolic root extract in rats. J Ethnopharmacol. 2002;81(2):231-7. [PubMed ID: 12065156].
-
27.
Rahman MA, Islam MS. Antioxidant, antibacterial and cytotoxic effects of the phytochemicals of whole Leucas aspera extract. Asian Pac J Trop Biomed. 2013;3(4):273-9. [PubMed ID: 23620850]. https://doi.org/10.1016/S2221-1691(13)60062-3.
-
28.
Sakat S, Juvekar AR, Gambhire MN. In vitro antioxidant and anti-inflammatory activity of methanol extract of Oxalis corniculata Linn. Int J Pharm Pharm Sci. 2010;2(1):146-55.