Abstract
Background:
Recently, medicinal plants have become the focus of more attention due to their potentially effective roles in the prevention and treatment of many diseases.Objectives:
The aim of this research was to examine the effects of Cinnamomum zeylanicum (CZ) on lipid profile and histological changes due to LDL receptor (LDL-R) gene expression in hamsters fed a high cholesterol diet.Methods:
Thirty-two male hamsters were allocated into four groups (eight in each group under four weeks of treatment) as follows: group ND were fed a standard normal diet; group HCD were fed a normal diet supplemented with high cholesterol; group HCD + CZ2 were fed a high cholesterol diet (HCD) along with 2% CZ; and group HCD + CZ8 were fed an HCD diet along with 8% CZ. After the treatment process, blood samples were taken from all of studied animals, and sera were prepared for lipid profile assays. Liver LDL-R gene expression was assayed using the real-time polymerase chain reaction (PCR) method. Liver histological changes were determined using the hematoxylin and eosin staining method.Results:
A significant reduction was observed in serum triglycerides (TG), total cholesterol (TC), low-density lipoprotein cholesterol (LDL-c), very-low-density lipoprotein cholesterol (VLDL-c), aspartate transaminase (AST), and alanine transaminase (ALT) levels in the HCD + CZ8 group, while serum high density lipoprotein cholesterol (HDL-c) levels increased when compared with the HCD group (P < 0.001). The HCD group demonstrated decreased LDL-R gene expression in the animals’ livers, whereas treatment with CZ up-regulated its expression. The scores for hepatic steatosis in the HCD + CZ8 group were diminished significantly.Conclusions:
The administration of CZ improved dyslipidemia in hypercholesterolemic animals, probably by increasing LDL-R gene expression in the liver; consequently, it can be concluded that CZ can reverse hepatic steatosis. Therefore, CZ was determined as having a protective role against hypercholesterolemia.Keywords
Cholesterol Hypercholesterolemia LDL Receptors LDL Cinnamomum zeylanicum
1. Background
Hypercholesterolemia is a common disorder in both developing and developed countries. Disturbances in lipid metabolism in such cases leads to increases in low-density lipoprotein cholesterol (LDL-c) and decreases in high-density lipoprotein cholesterol (HDL-c). Hypercholesterolemic patients are predisposed to diabetes, atherosclerosis, and coronary artery disease (1). Deposition of LDL-derived cholesterol in the intima of susceptible arteries results in the development of atherosclerosis. The LDL receptor (LDL-R) transfers the cholesterol-carrying lipoprotein particles into the cells, and binds the lipoproteins to the outer cell membrane and releases them into the endosome (2).
Lowering plasma lipoprotein levels, especially LDL-c, is important for reducing the risk of hyperlipidemic-induced complications. Moreover, high levels of HDL-c are involved in the transposition of cholesterol to the particle centers; this process enhances the removal of lipids from the arterial wall and is protective against atherosclerotic lesions (3). Also, uptake of cholesterol from the macrophage to the HDL is mediated by the ABC transmembrane transporters that diminish foam cell formation (4).
Recently, the use of herbal medicine has attracted significant interest among patients and researchers. Low triglyceride (TG) and LDL-c levels or high HDL-c levels, generally desirable health outcomes, are known to result from using some plant materials (5). More specifically, there are some reports indicating that using some herbal medicines can lower serum TC levels and therefore prevent hyperlipidemic complications (5-8).
A group of medicines which is known as the statin family inhibits ß-hydroxy-ß-methylglutaryl-coenzyme-A reductase (HMG-CoA reductase), a key enzyme in cholesterol metabolism regulation. HMG-CoA reductase is a rate-limiting enzyme in the pathway of cholesterol biosynthesis; furthermore, it is also involved in LDL receptor (LDL-R) regulation. There are, however, some reports showing that there may be side-effects for this group of medicines; for example, they have been shown to cause elevation in serum transaminase activity as well as increased intolerance or resistance to these drugs. Still, many researchers have suggested that increasing the expression of the LDL-R gene could reduce the serum cholesterol levels by increasing uptake of the LDL particles (8-12). For these reasons, researchers and patients have been interested in discovering hypolipidemic agents with minimal or no side-effects.
Cinnamomum zeylanicum (CZ from the Lauraceae family), which originates from the island of Sri Lanka southeast of India, has been used for its anti-diabetic (13-15), anti-inflammatory, anti-arthritic (16, 17), antipyretic, analgesic (18), antifungal (19), antioxidant (20), antimicrobial (21), and anti-Helicobacter pylori (22) properties, and also acts as a lipid-lowering agent (8, 23). Different parts of the plant (bark, roots, and leaves) and its essential oils are used for medicinal purposes (24).
2. Objectives
To determine the medicinal effects of CZ and its related mechanisms, we developed an animal model of hypercholesterolemia to study the therapeutic effects of CZ. The liver profiles and serum aminotransferase levels were determined in the treated and untreated animals. Moreover, histological changes and also LDL-R gene expression in the animals’ livers were studied as well. The current study aimed to examine the effects of (CZ) on lipid profiles, histological changes, and LDL-R gene expression in hamsters fed a high cholesterol diet
3. Methods
3.1. Cinnamon Powder, Animal, and Diet Preparation
CZ bark was purchased from a local market in Hamadan (west of Iran), and after confirmation by a specialist in a medicinal plant center, was powdered with an electric blender (cinnamon powder).
The experiments were conducted on 32 male golden hamsters eight weeks of age, weighing approximately 120 g. The animals were housed under a 12:12 hours light and dark cycle in a controlled animal house. They were fed with a standard pellet diet and water ad libitum. After the animals were acclimatized, they were randomly divided into four groups of eight animals each (23, 25).
The first group was used as the healthy control group, receiving only a normal chow diet (ND). The second group was fed with a high cholesterol diet (HCD) that contained the normal diet supplemented with 2% cholesterol (Sigma Company, UK) and 0.5% cholic acid dissolved in 5 ml of boiled olive oil. The third and fourth groups were fed a high cholesterol diet supplemented with 2% and 8% CZ powder, respectively (HCD + CZ2 and HCD + CZ8 groups, respectively). This treatment protocol lasted for four weeks. The protocol was approved by the local ethical committee.
3.2. Serum and Tissue Preparation
At the end of treatment period and after an overnight fasting period, all animals were euthanized using diethyl ether as an anesthetic agent. By means of cardiac puncture, blood samples were taken from all of the studied animals. The collected samples were centrifuged (2500 g for 10 minutes) for serum separation. Subsequently, the sera were separated and transported to the laboratory for biochemical analysis. Sections of each of the animal’s liver tissues were removed, washed with saline, frozen in liquid nitrogen, and maintained at -70°C for gene expression analysis using a real-time polymerase chain reaction (PCR). Another portion of the tissue was fixed using 10% buffered formalin and embedded in paraffin for histological analysis (3).
Serum triglycerides (TG), total cholesterol (TC), high-density lipoprotein cholesterol (HDL-c), aspartate transaminase (AST), and alanine transaminase (ALT) levels were measured using commercially-available spectrophotometric kits (Pars Azmun Co., Iran). Low-density lipoprotein cholesterol (LDL-c) was calculated with the Fridewald formula (LDL = TC - HDL - TG/5.0 (mg/dL)). Very-low-density lipoprotein cholesterol (VLDL-c) was calculated by the TG/5 equation according to a previously published paper (26).
3.3. Evaluation of LDL-R Gene Expression Using Real-Time PCR
Extraction of total ribonucleic acid (RNA) from the liver was performed using a commercial kit (TRIZOL, Invitrogen) according to the kit’s protocol. The purity of the RNAs was estimated using optical density ratio determination at 260/280 nm with a Nano-Drop spectrophotometer (Bio-TeK, USA). cDNA was synthesized from isolated RNA using random hexamer primer and a Thermo ScientificTM RevertAidTM First Strand cDNA Synthesis Kit (#K1622). qRT-PCR analysis was carried out on the synthesized cDNA using the SYBR Green method (SYBR Premix Ex Taq 2 Kit, TakaRa No. RR820L) on the BioRad system (26).
The characteristics of the designed primers used for real-time PCR are shown in Table 1. The primers were designed by Allele ID software (version 7.6, Premier Biosoft Corporation, USA). Glyceraldehyde 3-phosphate dehydrogenase (GAPDH), a housekeeping gene, was used as genetic reference. The primer pair’s properties are shown in Table 1.
Characteristics of Primers Used in the Amplification of Hamster LDL-R and GAPDH Genes
Gene | Primer | Sequence of Primer (5’ to 3’ Orientation) | GC Content, % | Tm, °C | Primer Length | Product Length, bp |
---|---|---|---|---|---|---|
LDLR | Forward | CTCCACTCTATCTCCAGCATT | 47.6 | 49.8 | 21 bp | 200 |
Reverse | GACAAGAGGTTTTCAGCCACCAAA | 45.8 | 60.2 | 24 bp | ||
GAPDH | Forward | TGGCCTTCCGTGTTCCTACC | 58.3 | 60 | 20 bp | 135 |
Reverse | TAGCCCAGGATGCCCTTCAG | 58.6 | 60 | 20 bp |
The characteristics of PCR amplification (35 cycle) were: denaturation at 95°C (30 seconds), annealing at 60°C (30 seconds), and extension at 72°C (45 seconds). The final step was a single extension cycle at 72°C for 5 minutes. To confirm the quality and integrity of the synthesized cDNA, agarose gel (1%) electrophoresis was run as well.
The cycle threshold (CT) was measured for the LDL-R and GAPDH genes in all samples; after calculation of ΔCT (LDL-R CT–GAPDH CT), the mean of ΔCT in each group was determined. A higher ΔCT is indicative of lower gene expression. To compare the relative differences in gene expression among the studied groups, ΔΔCT (ΔCTSample-ΔCTControl) and 2-ΔΔCT were calculated. 2-ΔΔCT indicates the fold of change in gene expression compared to the control group (26).
3.4. Histological Analysis of the Liver
The liver tissue sections (5 μm) were stained with hematoxylin and eosin (H & E). They were then evaluated under a light microscope. Then, the steatotic degrees of each prepared liver sample were checked and scored by a pathologist, and the histological changes were recorded in accordance with the percentage of hepatocytes containing lipid droplets (27). All presented data were recorded as mean ± standard error of the mean. Comparison of the data was carried out using a one-way analysis of variance (ANOVA), followed by Dunnett’s test (SPSS version 11.5). Differences were considered significant at P < 0.05.
4. Results
Figure 1 shows a comparison of the serum levels of TG, TC, LDL-c, HDL-c, and VLDL-c between the studied groups. In the HCD group that received hypercholesterolemic diet, the serum levels of TG, TC, VLDL-c, and LDL-c increased significantly compared to the corresponding rates for the ND group (P < 0.05), whereas HDL-c levels reduced significantly (P < 0.05). Comparing the lipid profiles between the HCD + CZ2 and ND groups showed no significant differences. In the HCD + CZ8 group that received higher doses of CZ (8%), significant decreases in TG (36.6%), TC (42.45), LDL-c (77.7%), VLDL-c (36.6%), AST (12.2%) levels were observed when compared against the HCD group (Figure 1, P < 0.001). Also, an increase in HDL-c levels (11.8%) was found in the HCD + CZ8 group when compared with the HCD group. Furthermore, comparing HDL-c levels between the HCD + CZ8 and ND groups showed a significant reduction in the former group (P < 0.001). Changes in serum aminotransferases in the same groups are shown in Figure 2. As this figure shows for the HCD + CZ8 group, both AST and ALT levels reduced significantly in comparison to the corresponding rates for the HCD group, while for the HCD + CZ2 group, only AST levels reduced significantly (P < 0.01).
Comparison of the Lipid Profile in Treated Group (A - C)
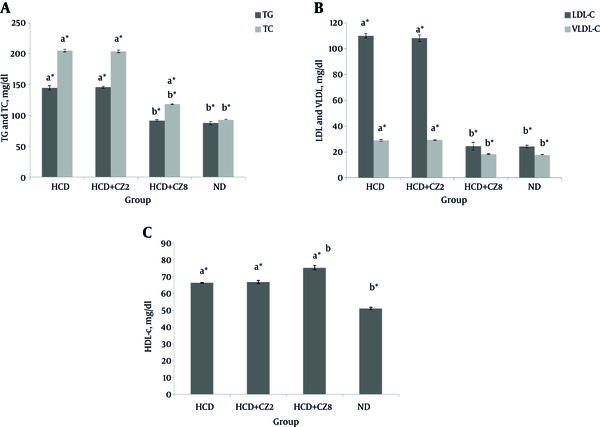
Comparison of AST and ALT Activity Among Different Groups
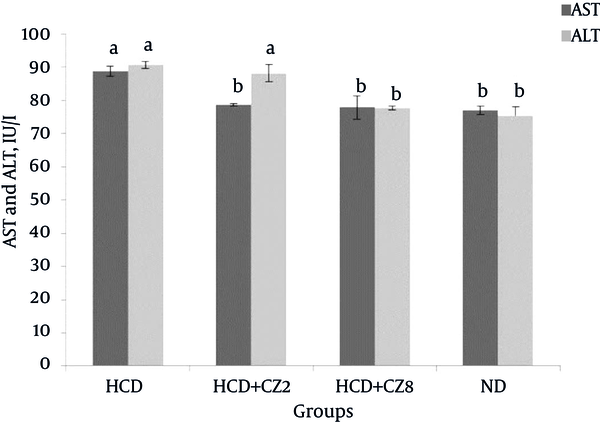
The results of the gel electrophoresis of the PCR products is shown in Figure 3, which confirms the proper obtained size.
Amplification of LDL-R and GAPDH Gene PCR Products (With 200 and 137 bp Length) as Confirmed by Gel Electrophoresis
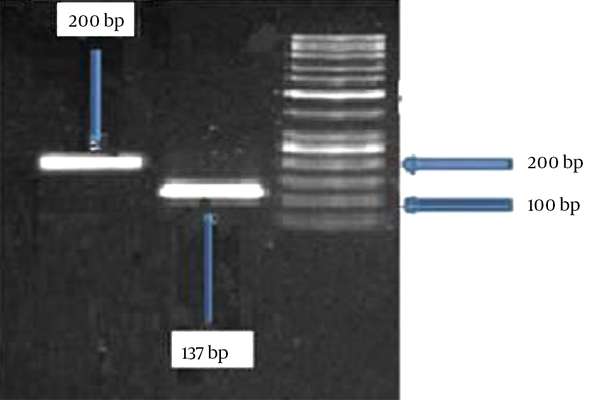
The results of the qRT-PCR are summarized in Table 2. A significant decrease in LDL-R gene expression (higher ΔCT) was observed in the HCD group as compared to the ND group, which shows the effects of four weeks on a hypercholesterolemic diet (Table 2). The calculation of 2-ΔΔCT also confirmed a 23.4-fold reduction in LDL-R gene expression in the HCD group as compared to the ND group. Additionally, there was an increase in LDL-R gene expression in the HCD + CZ2 group (19.18 fold) compared to the corresponding rates for the HCD group. Similar results also found for the HCD + CZ8 group, which showed a 20.79-fold increase in LDL-receptor gene expression as compared to the HCD group.
Following the staining of the liver tissues with H & E, the degree of hepatic steatosis was determined (Figure 4). In the liver slides of the hamsters fed with an ND, there was no noticeable steatosis, while remarkable liver steatosis was observed in the hamsters fed a HCD (Figure 4). These observations also indicated a significantly lower score of hepatic steatosis in the HCD + CZ8 group compared to that in the HCD group. Furthermore, no significant difference was observed in the scores of liver steatosis between the HCD + CZ8 and ND groups, which supports the effects of treatment with CZ8.
Pathologic Degrees of the Hamster Livers
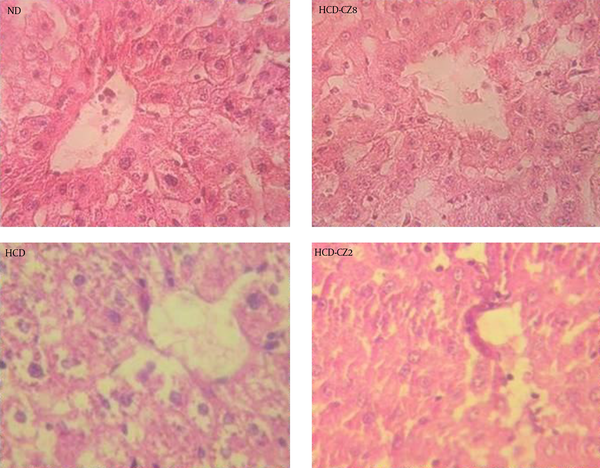
Further analysis revealed that in the HCD + CZ2 group, hepatic steatosis scores were lower than those of the HCD group; nevertheless, the scores in the HCD + CZ2 group were still higher than those of the ND group.
5. Discussion
In the present study, four weeks of administering a hypercholesterolemic diet to male hamsters resulted in the development of hypercholesterolemia, while supplementation with cinnamon improved the lipid profiles. Elevation of serum LDL-c in the group that received HCD might have resulted in reduction of liver LDL receptors, which was supported by increasing the ΔCT for the LDL-R gene; this could attributed to hypercholesterolemia itself. HCD also amplified the lipogenesis pathway, which increases TG, TC, free fatty acid, and phospholipids production (28).
Amin et al. revealed that the administration of an HCD to male albino rats led to hypercholesterolemia induction (23). After three and six weeks of this diet regimen, a significant increase was observed in serum TC, TG, and LDL-c concentrations (P < 0.05), while in both examined periods, HDL-c levels in the HCD group decreased in comparison to those of the ND group (23). Although the effects of HCD on TC, TG, and LDL-c serum concentrations in that study were similar to those of our investigation, the changes in HDL-c levels were in contrast to our findings (23).
Badalzadeh et al. reported that long-term cinnamon supplementation and regular aerobic training have synergistic effects on cardiac activities in rats (29). The improvement in cardiac function through both cinnamon supplementation and regular training was associated with amelioration of serum lipid profiles (n = 6 in each group) (29); this lipid-lowering effect was similar to the findings in our study.
Mang et al. studying type 2 diabetic patients, indicated that cinnamon did not have a significant effect on plasma lipid profiles (30); this result is quite different from what we found in the present study. However, another study on an animal model of type 2 diabetes (high-fructose fed rats) showed lipid-lowering effects of cinnamon, which was consistent with our study (31).
Lee et al. showed that treatment with cinnamon in rats with high-fat regimens reduced the cholesterol concentration through inhibition of hepatic 5-hydroxy-3-methylglutaryl-coenzyme-A (HMG-CoA) reductase (32), whereas our study emphasized the hypocholesterolemic effects of cinnamon via upregulation of LDL-c by increasing LDL-R gene expression as another probable mechanism with the same results.
As has been reported in many studies, the liver transaminases (AST and ALT) are elevated significantly with a HCD compared with ND subjects, which may be the result of hepatocellular damage following hypercholesterolemia (33, 34). Since ALT activity in the liver is higher than that of other tissues, this enzyme is used as a more specific marker for hepatocellular damage in comparison to AST (35).
According to Amin et al. “Increased serum ALT and AST usually indicate hepatocellular damage and the most common presentation is fatty liver” (23). In their study on mice, AST and ALT increased significantly, and three weeks of cinnamon treatment significantly decreased their levels, suggesting that cinnamon may play an important role in improving liver function (23). Eidi et al.’s investigation indicated that CZ has protective effects in hepatocellular injuries induced by carbon tetrachloride in rats (36).
Gandhi et al. showed that the continuous intake of green tea (2 g) along with cinnamon extract (2.5 g) significantly improved some lipid parameters such as TC, TG, LDL-c, and HDL-c, and moderately reduced body weight and body fat distribution without any vigorous lifestyle changes even in overweight people without any adverse personal habits (37). Treatment of a HCD animals with 2 g CZ/100 g (HCD + CZ2 group) did not show any significant effects on lipid profiles and ALT in this study, but a significant reduction in AST levels was observed. In other words, the low dose of cinnamon could not provide protection against dyslipidemia and hepatic damage, even though LDL-R gene expression was elevated significantly compared with those of hamsters fed a HCD. It seems that low doses of CZ could not provoke the other mechanisms necessary for amelioration of induced hypercholesterolemia.
Rahman et al. administered a 35-day high-fat regimen to rats using a fatty mixture diet containing 15% powdered Cinnamomum cassia and compared the animals’ lipid profiles with those of a control group that did not receive Cinnamomum (8). They reported significantly lower serum levels of TC, LDL-C, and TG in the treated group, while HDL-c levels were not significantly changed (8). In the present study, the HDL-c level increased following administration of 8% powdered cinnamon for four weeks.
Javed et al. showed that CZ treatment decreased TC, LDL-c, and TG levels, and increased HDL-c levels in hyperlipidemic albino rabbits (38). Hassan et al. have also shown similar results in diabetic subjects (39). In contrast to these observations, Sambaiah et al. examined the effects of cinnamon on rats’ lipid profiles at a concentration proportional to what is used in human diets, and indicated that this treatment did not change the animals’ lipid profiles in a significant way (40).
In spite of these reports, the precise mechanisms involved in the CZ effects on serum lipid improvement have not been definitively characterized in the literature. Since CZ contains dietary fiber at a relatively high level, it can decrease the lipid absorption in the intestine. On the other hand, CZ contains antioxidant vitamins that can augment lipid catabolism. Due to increases in insulin levels, an anabolic hormone with regulatory action in lipid metabolism has been reported following CZ treatment; it may also contribute to the reduction of lipid levels.
The hypolipidemic effects of some plant extracts may be due to decreases in hepatic-3-hydroxy-3-methylglutaryl-CoA reductase, cholesterol 7α-hydroxylase, pentose-phosphate pathways, or antioxidant activities (3, 21, 24, 41, 42). Other mechanisms have also been reported. LDL-R gene expression could be one of the mechanisms involved in the scavenging of LDL-c; however, few researchers have studied this mechanism thus far. Although Venkatesan et al. emphasized the involvement of several mechanisms in LDL uptake, only one of the mechanisms was LDL-R gene expression (43).
The present study demonstrated that cinnamon increased LDL-R gene expression in the livers of hamsters; hence, this could likely result in amelioration of dyslipidemia. We demonstrated that low doses of CZ could not provide protection against dyslipidemia and hepatic damage, although such doses could exert a significant 19.18-fold increase in LDL-R gene expression in HCD + CZ2 group. LDL-R gene expression was increased 20.79 fold in the HCD + CZ8 group, and this can justify the aforementioned amelioration in serum LDL-c and cholesterol levels.
Some reports indicated that the statins that inhibit HMG-CoA reductase gene expression can lower serum cholesterol levels, but taking these drugs for long periods of time and at high doses can lead to hepatocellular damage, rhabdomyolysis, and resistance as well as intolerance (9-11, 42). The findings in this study showed no changes in AST and ALT levels in the serum following higher doses of CZ consumption, indicating no harmful effects on the liver. However, da Cunha V et al.’s study indicated a reduction in HDL-c levels lower than the baseline (normal group); according to our experiments, HDL-c levels in hypercholesterolemic animals was higher than those of the normal group, and hence the results of our study were different (44).
We found that HCD decreased LDL-R gene expression in the animal model of hypercholesterolemia, while treatment with cinnamon up-regulated its expression. In the present study, histological analysis indicated that hepatic steatosis scores in the group treated with cinnamon significantly diminished in comparison to those of the control (hamsters fed HCD); the change was obvious in the group that received higher CZ doses. These changes confirm the effectiveness of CZ on the amelioration of lipid profiles and the reversal of liver steatosis and fatty liver in a dose-related manner.
In summary, our results indicated that the supplementation of cinnamon results in protection against dyslipidemia and hepatic damage following the administration of a high cholesterol diet. Increases in the expression of the LDL-R gene following cinnamon consumption might be another mechanism that can explain the lipid-lowering activity. Also, the results of our biochemical analysis and histopathologic experiments confirmed no side effects for this herbal medicine; therefore, it can be suggested for use as an anti-hypercholesterolemic agent; however, more clinical studies on human subjects are required to reveal further details and confirm this generalization.
Acknowledgements
References
-
1.
Nelson RH. Hyperlipidemia as a risk factor for cardiovascular disease. Prim Care. 2013;40(1):195-211. [PubMed ID: 23402469]. https://doi.org/10.1016/j.pop.2012.11.003.
-
2.
Mathur M, Kusum Devi V. Potential of novel drug delivery strategies for the treatment of hyperlipidemia. J Drug Target. 2016:1-11. [PubMed ID: 27029893]. https://doi.org/10.3109/1061186X.2016.1172586.
-
3.
Mohammadi A, Bazrafshani MR, Oshaghi EA. Effect of garlic extract on some serum biochemical parameters and expression of npc1l1, abca1, abcg5 and abcg8 genes in the intestine of hypercholesterolemic mice. Indian J Biochem Biophys. 2013;50(6):500-4. [PubMed ID: 24772974].
-
4.
Tavoosi Z, Moradi-Sardareh H, Saidijam M, Yadegarazari R, Borzuei S, Soltanian A, et al. Cholesterol Transporters ABCA1 and ABCG1 Gene Expression in Peripheral Blood Mononuclear Cells in Patients with Metabolic Syndrome. Cholesterol. 2015;2015:682904. [PubMed ID: 26788366]. https://doi.org/10.1155/2015/682904.
-
5.
Sham TT, Chan CO, Wang YH, Yang JM, Mok DK, Chan SW. A review on the traditional Chinese medicinal herbs and formulae with hypolipidemic effect. Biomed Res Int. 2014;2014:925302. [PubMed ID: 25110708]. https://doi.org/10.1155/2014/925302.
-
6.
Belguith-Hadriche O, Bouaziz M, Jamoussi K, Simmonds MS, El Feki A, Makni-Ayedi F. Comparative study on hypocholesterolemic and antioxidant activities of various extracts of fenugreek seeds. Food Chem. 2013;138(2-3):1448-53. [PubMed ID: 23411266]. https://doi.org/10.1016/j.foodchem.2012.11.003.
-
7.
Mohammadi A, Oshaghi EA. Effect of garlic on lipid profile and expression of LXR alpha in intestine and liver of hypercholesterolemic mice. J Diabetes Metab Disord. 2014;13(1):20. [PubMed ID: 24476027]. https://doi.org/10.1186/2251-6581-13-20.
-
8.
Rahman S, Begum H, Rahman Z, Ara F, Iqbal M, Mohammad YA. Effect of cinnamon (cinnamomum cassia) as a lipid lowering agent on hypercholesterolemic rats. J Enam Med College. 2013;3(2):94-8.
-
9.
Floyd JS, Brody JA, Tiniakou E, Psaty BM, Mammen A. Absence of anti-HMG-CoA reductase autoantibodies in severe self-limited statin-related myopathy. Muscle Nerve. 2016;54(1):142-4. [PubMed ID: 27038110]. https://doi.org/10.1002/mus.25127.
-
10.
Tada H, Kobayashi J, Kawashiri MA, Miyashita K, Nohara A, Inazu A, et al. Changes in lipoprotein lipase and endothelial lipase mass in familial hypercholesterolemia during three-drug lipid-lowering combination therapy. Lipids Health Dis. 2016;15:66. [PubMed ID: 27039080]. https://doi.org/10.1186/s12944-016-0238-z.
-
11.
Sahebkar A, Watts GF. New therapies targeting apoB metabolism for high-risk patients with inherited dyslipidaemias: what can the clinician expect? Cardiovasc Drugs Ther. 2013;27(6):559-67. [PubMed ID: 23913122]. https://doi.org/10.1007/s10557-013-6479-4.
-
12.
Vijayakumar MV, Pandey V, Mishra GC, Bhat MK. Hypolipidemic effect of fenugreek seeds is mediated through inhibition of fat accumulation and upregulation of LDL receptor. Obesity (Silver Spring). 2010;18(4):667-74. [PubMed ID: 19851306]. https://doi.org/10.1038/oby.2009.337.
-
13.
Dugoua JJ, Seely D, Perri D, Cooley K, Forelli T, Mills E, et al. From type 2 diabetes to antioxidant activity: a systematic review of the safety and efficacy of common and cassia cinnamon bark. Can J Physiol Pharmacol. 2007;85(9):837-47. [PubMed ID: 18066129]. https://doi.org/10.1139/Y07-080.
-
14.
Ranasinghe P, Pigera S, Premakumara GA, Galappaththy P, Constantine GR, Katulanda P. Medicinal properties of 'true' cinnamon (Cinnamomum zeylanicum): a systematic review. BMC Complement Altern Med. 2013;13:275. [PubMed ID: 24148965]. https://doi.org/10.1186/1472-6882-13-275.
-
15.
Goodarzi MT, Tootoonchi AS, Karimi J, Abbasi OE. Anti-diabetic effects of aqueous extracts of three Iranian medicinal plants in type 2 diabetic rats induced by high fructose diet. Avi J Med Biochem. 2013;1(1):7-13.
-
16.
Joshi K, Awte S, Bhatnagar P, Walunj S, Gupta R, Joshi S, et al. Cinnamomum zeylanicum extract inhibits proinflammatory cytokine tnf: In vitro and in vivo studies. Res Pharmaceutical Biotechnol. 2010;2(2):14-21.
-
17.
Vetal S, Bodhankar SL, Mohan V, Thakurdesai PA. Anti-inflammatory and anti-arthritic activity of type-a procyanidine polyphenols from bark of cinnamomum zeylanicum in rats. Food Sci Human Wellness. 2013;2(2):59-67.
-
18.
Manosi D, Suvra M, Budhimanta M, Jayram H. Ethnobotany, phytochemical and pharmacological aspects of Cinnamomum zeylanicum blume. Inter Res J Pharm. 2013;4:58-63.
-
19.
Simic A, Sokovic MD, Ristic M, Grujic-Jovanovic S, Vukojevic J, Marin PD. The chemical composition of some Lauraceae essential oils and their antifungal activities. Phytother Res. 2004;18(9):713-7. [PubMed ID: 15478207]. https://doi.org/10.1002/ptr.1516.
-
20.
Taher M, Deny S, Mohamad Roji S, Hasnah M, Fadzilah A, Farediah A. Antioxdant activity of cinnamtannin B1 from cinnamomum zeylanicum blume. Phytomedicines. 2006;16:601-8.
-
21.
Jayaprakasha GK, Rao LJ. Chemistry, biogenesis, and biological activities of Cinnamomum zeylanicum. Crit Rev Food Sci Nutr. 2011;51(6):547-62. [PubMed ID: 21929331]. https://doi.org/10.1080/10408391003699550.
-
22.
Hosseini ZN, Moghadam S, Ebrahimi F, Abdollahi M, Zahedi M, Nazari M. In Vitro screening of selected Iranian medicinal plants against helicobacter pylori. Int J of Green Pharm. 2011;5:282-5.
-
23.
Amin KA, Abd El-Twab TM. Oxidative markers, nitric oxide and homocysteine alteration in hypercholesterolimic rats: role of atorvastatine and cinnamon. Int J Clin Exp Med. 2009;2(3):254-65. [PubMed ID: 19918318].
-
24.
Pandey S, Pandey R, Singh P. Phytochemical screening of selected medicinal plant cinnamon zeylanicum bark extract, area of research; uttarakhand, India. Inter J Sci Res Public. 2014;4:1-6.
-
25.
Abbasi O. E, Noori S. A, Rezaei A. Effects of walnut on lipid profile as well as the expression of sterol-regulatory element binding protein-1c(SREBP-1c) and peroxisome proliferator activated receptors α(PPARα) in Diabetic Rat. Food Nut Sci. 2012:255-9.
-
26.
Abbasi Oshaghi E, Khodadadi I, Saidijam M, Yadegarazari R, Shabab N, Tavilani H, et al. Lipid Lowering Effects of Hydroalcoholic Extract of Anethum graveolens L. and Dill Tablet in High Cholesterol Fed Hamsters. Cholesterol. 2015;2015:958560. [PubMed ID: 26823981]. https://doi.org/10.1155/2015/958560.
-
27.
Uno M, Kurita S, Misu H, Ando H, Ota T, Matsuzawa-Nagata N, et al. Tranilast, an antifibrogenic agent, ameliorates a dietary rat model of nonalcoholic steatohepatitis. Hepatology. 2008;48(1):109-18. [PubMed ID: 18571789]. https://doi.org/10.1002/hep.22338.
-
28.
Lai YS, Lee WC, Lin YE, Ho CT, Lu KH, Lin SH, et al. Ginger essential oil ameliorates hepatic injury and lipid accumulation in high fat diet-induced nonalcoholic fatty liver disease. J Agric Food Chem. 2016;64(10):2062-71. [PubMed ID: 26900108]. https://doi.org/10.1021/acs.jafc.5b06159.
-
29.
Badalzadeh R, Shaghaghi M, Mohammadi M, Dehghan G, Mohammadi Z. The effect of Cinnamon extract and long-term aerobic training on heart function, biochemical alterations and lipid profile following exhaustive exercise in male Rats. Adv Pharm Bull. 2014;4:515-20.
-
30.
Mang B, Wolters M, Schmitt B, Kelb K, Lichtinghagen R, Stichtenoth DO, et al. Effects of a cinnamon extract on plasma glucose, HbA, and serum lipids in diabetes mellitus type 2. Eur J Clin Invest. 2006;36(5):340-4. [PubMed ID: 16634838]. https://doi.org/10.1111/j.1365-2362.2006.01629.x.
-
31.
Asadi S, Sohrabi M, Zarei S, Ghyasvand T, Rezaei Farimani A, Goodarzi MT. Effects of Curcuma longa and Cinnamon aqueous extracts on Serum Carbohydrates and Lipids metabolism and oxidative status in high Fructose fed Rats. Int Res J Biological Sci. 2014;3(3):78-83.
-
32.
Lee JS, Jeon SM, Park EM, Huh TL, Kwon OS, Lee MK, et al. Cinnamate supplementation enhances hepatic lipid metabolism and antioxidant defense systems in high cholesterol-fed rats. J Med Food. 2003;6(3):183-91. [PubMed ID: 14585184]. https://doi.org/10.1089/10966200360716599.
-
33.
Prasanna M. Hypolipidemic Effect of Fenugreek: A clinical study. Ind J Pharmacol. 2000;32:34-6.
-
34.
Mohammadi A, Oshaghi EA, Noori Sorkhani A, Oubari F, Hosseini KR, Rezaei A. Effect of opium on lipid profile and expression of liver X receptor alpha (LXRα) in normolipidemic mouse. Food Nut Sci. 2012;3:249-54.
-
35.
Abbasi Oshaghi E, Tavilani H, Khodadadi I, Goodarzi MT. Dill tablet: a potential antioxidant and anti-diabetic medicine. Asian Pac J Trop Biomed. 2015;59:720-7.
-
36.
Eidi A, Eidi M, Sookhteh M. Effect of alcoholic extracts Fenugreek seeds (Trigonella foenum graecum L.) in the activity of hepatic enzymes in normal and diabetic rats. J Med Plant. 2006;5:36-41.
-
37.
Gandhi K, Sasikumar S, Eagappan K. Coalesced impact of green tea and cinnamon on metabolic parameters of overweight subjects. J Nutr Disord Ther. 2015;2015.
-
38.
Javed I, Faisal I, Rahman Z, Khan MZ, Muhammad F, Aslam B, et al. Lipid lowering effect of Cinnamomum zeylanicum in hyperlipidaemic albino rabbits. Pak J Pharm Sci. 2012;25(1):141-7. [PubMed ID: 22186322].
-
39.
Hassan SA, Barthwal R, Nair MS, Haque SS. Aqueous bark extract of Cinnamomum Zeylanicum: a potential therapeutic agent for streptozotocin-induced type 1 diabetes mellitus (T1DM) rats. Trop J Pharm Res. 2012;11(3):429-35.
-
40.
Sambaiah K, Srinivasan K. Effect of cumin, cinnamon, ginger, mustard and tamarind in induced hypercholesterolemic rats. Nahrung. 1991;35(1):47-51. [PubMed ID: 1865890].
-
41.
Abbasi Oshaghi E, Tavilani H, Khodadadi I, Goodarzi MT. Effect of dill tablet (Anethum graveolens L) on antioxidant status and biochemical factors on carbon tetrachloride-induced liver damage on rat. Int J Appl Basic Med Res. 2016;6:111-4.
-
42.
Kumano-Kuramochi M, Fujimura T, Komba S, Maeda-Yamamoto M, Machida S. Screening, expression, and characterization of an anti-human oxidized low-density lipoprotein single-chain variable fragment. J Biosci Bioeng. 2016;122(3):287-93. [PubMed ID: 27038672]. https://doi.org/10.1016/j.jbiosc.2016.02.014.
-
43.
Venkatesan N, Devaraj SN, Devaraj H. Increased binding of LDL and VLDL to apo B,E receptors of hepatic plasma membrane of rats treated with Fibernat. Eur J Nutr. 2003;42(5):262-71. [PubMed ID: 14569407]. https://doi.org/10.1007/s00394-003-0420-8.
-
44.
da Cunha V, Martin-McNulty B, Vincelette J, Zhang L, Rutledge JC, Wilson DW, et al. Interaction between mild hypercholesterolemia, HDL-cholesterol levels, and angiotensin II in intimal hyperplasia in mice. J Lipid Res. 2006;47(3):476-83. [PubMed ID: 16371646]. https://doi.org/10.1194/jlr.M500341-JLR200.