Abstract
Background:
Natural products derived from plants play a significant function in healthcare in many cultures, both ancient and modern. curcumin (CUR) is a chemical constituent of turmeric with anticancer activity, but its poor water solubility limits its use clinically.Methods:
To improve the bioavailability and water solubility of curcumin, we synthesized five series of poly (caprolactone)-poly (ethylene glycol)- poly (caprolactone) (PCL-PEG-PCL) triblock copolymers. The structure of the copolymers was characterized by HNMR, FT-IR, DSC, and GPC techniques. The nanoparticles (NPs) were prepared using a solvent evaporation method. To achieve the best delivery system, we assigned the effect of the length of the copolymers’ hydrophilic and hydrophobic chains on the encapsulation of hydrophobic CUR, performed entrapment efficiency and drug loading assignments, as well as evaluated the particle distribution and in vitro release using the direct dispersion method.Results:
CUR was encapsulated with 71% and 83% efficiency in biodegradable nanoparticulate formulations, based on NP4 and NP5. Dynamic laser light scattering (DLS) indicated a particle diameter of 112 nm and 110 nm for NP4 and NP5, respectively. FT-IR and DSC analysis of the NPs showed that CUR was encapsulated into the NPs. The in vitro release experiments showed that NP5 controlled the release of CUR more effectively, with only 51% of CUR released after 120 hours.Conclusions:
The results indicate the successful formulation of curcumin-loaded PCL-PEG-PCL nanoparticles and improved water solubility of curcumin, which may have potential application in cancer treatment.Keywords
1. Background
Curcumin, a chemical constituent of turmeric, has been applied in the treatment of inflammatory disorders and cancer for many years (1-4). There is some data signifying that curcumin is a principle chemo sensitizer for chemotherapy and that it helps patients avoid the side effects of treatment (5, 6). Curcumin may reduce tumor growth via multiple mechanisms, including antitumor angiogenesis, suppression of proliferation, induction of apoptosis, and prevention of metastasis (7). However, the clinical applications of curcumin remain limited because of its short biological half-life, poor solubility resulting in poor absorption, and low bioavailability via the oral route (8, 9).
Nanotechnology has the potential to defeat the poor water solubility of lipophilic drugs (10-12). Encapsulation of hydrophobic drugs into NPs is an advance that clinicians hope will allow the drug to be formulated into one that is intravenously injectable. The amphiphilic NPs that include a hydrophobic core and a hydrophilic shell are excellent candidates for carrying hydrophobic drugs.
PCL-PEG-PCL copolymers that self-assemble into NPs with core-shell structure include a hydrophobic PCL core and a hydrophilic PEG shell. To achieve controlled drug release, biodegradable polymeric nanoparticles are often used in advanced anticancer drug delivery systems (13-15). Some drug delivery systems that employ a biodegradable polymer, such as nanoparticles delivering anticancer agents, are commercially available. PCL-PEG-PCL copolymers are biodegradable, easy to produce, have an amphiphilic character, and have strong potential application in drug delivery systems (16-18).
In order to improve the therapeutic efficiency of curcumin, various approaches for encapsulating curcumin have been recently introduced, including liposomal curcumin (19), PEG-curcumin conjugate, and PCL-PEG-PCL nanofibers encapsulating curcumin (20, 21). For this reason, it seems that encapsulating of curcumin in PCL-PEG-PCL nanoparticles is a novel delivery vehicle for curcumin in in vitro cancer therapy.
2. Methods
2.1. Materials
Curcumin (Merck, Darmstadt, Germany, Art No. 820354), stannous 2-ethyl-hexanoate (Sn (Oct) 2) (Aldrich, St. Louis, USA, CAS. 301100), PEG (Mn = 6000 Da) (Aldrich, St. Louis, USA, CAS.81323), ε-caprolactone (98% purity) (Aldrich, St. Louis, USA, CAS.502443), and other chemicals and solvent were purchased.
2.2. Synthesis of PCL-PEG-PCL Copolymer
The synthesis of PCL-PEG-PCL copolymers was prepared according to our previous report (22) using a ring opening polymerization of ε-caprolactone, with PEG as the initial molecule and Sn (Oct)2 as catalyst (22). The polyethylene glycole was kept under a high vacuum at 80°C for two hours to remove all moisture. ε-caprolactone (1, 2, 4, 8, and 10g), PEG (2g), and Sn (Oct) 2 (0.01 mmol) were heated to 120°C under a nitrogen atmosphere. The polymerization reaction was achieved by stirring. After 12 hours, the resulting product was cooled to room temperature (23°C), dissolved in dichloromethane, and precipitated in cold diethyl ether. The mixture was filtered, and the purification process was repeated twice more. The obtained triblock copolymer was dried under vacuum at room temperature for 24 hours.
The obtained copolymer was characterized by Fourier Transform Infrared spectroscopy (FT-IR) (Bruker, Tensor 27) was used for characterization of the chemical structure of the PCL-PEG-PCL copolymer and Proton Nuclear Magnetic Resonance Spectroscopy (1 H NMR) in CDCl3 at 400 MHz (Bruker, Evans, 400). Thermal analysis of the triblock copolymers was determined by differential scanning calorimetry (DSC) (Mettler Toledo, model Star SW 9.30) and the data was recorded from 0 to 250°C. Molecular weight and distribution of the PCL-PEG-PCL copolymers were calculated by gel permeation chromatography (GPC) (Knaure, Berlin, Germany) composed of an ultrastyragel column and differential refractometric detector (4.6 × 30 mm) (Waters, Milford, USA, model HR 4E). Tetrahydrofuran (THF) as a mobile phase with a flow-rate of 1 mL/minute, and the injection volume was 100µl of stock solutions (0.1 - 0.5 w/v %). The standards of polystyrene mono, dispersed in the range of 4,500 - 29,500 DA (Varian Palo Alto, CA), obtained before measurements for use as the calibration curve for determination of average molecular weight of the PCL-PEG-PCL copolymers.
2.3. Preparation of Nanoparticles
The nano precipitation method was used to load the curcumin to the nanoparticles (22, 23). PCL-PEG-PCL copolymers (20 mg) and curcumin (1, 2, 5, 10, 15, and 20 mg) were dissolved in 2 mL of chloroform. The solution was then introduced into 20 mL of water under consistent mixing and was stirred magnetically at room temperature to achieve evaporation of the organic solvent. The chloroform was then thoroughly removed by rotary vacuum evaporation at 35°C. The resulting yellowish aqueous solution was filtered through a 0.45 µm filter membrane to remove the unloaded CUR. Nanoparticles were obtained by self-assembling, and the products were separated by centrifuging at 20,000 g for 20 minutes and freeze-dried under a pressure of 14 Pa at -78°C.
2.4. Characterization and Physicochemical Properties of the Nanoparticles
2.4.1. Particle Morphology
Atomic Force Microscopy (AFM) (JPK, Berlin, Germany, and model Nano Wizard 2) was used for morphological observation. One drop of NP solution was placed on freshly cleaved mica copper on substrate (1 cm2), followed by air-drying.
2.4.2. Particle Size and Zeta Potential of Curcumin-Loaded Nanoparticles
Dynamic light scattering (DLS) (Malvern Instruments, Worcestershire, UK, model Nano ZS) was used for determination of the particle size and zeta potential of the nanoparticles.
2.4.3. Stability of Nanoparticles
For evaluation of the physical stability of the nanoparticles, the particle size distribution of the nanoparticles was monitored by suspension of the nanoparticles in phosphate-buffer saline (PBS, pH = 7. 4) and kept at ambient temperature at times of 0, 15, and 30 days after preparation, using the method described in 2.4.
2.4.4. Drug Loading and Encapsulation Efficiency
The CUR content in the drug-loaded NPs was determined using HPLC at 420 nm. The drug-loading content and drug entrapment efficiency were calculated based on the following formula (22):
The drug loading ratio was evaluated by Equation 1:

Wdrug in nanoparticles shows weight of the encapsulated drug and Wnanoparticles shows the total weight of the corresponding drug-encapsulated nanoparticles. DL% is the drug-loading ratio (%).
For evaluation of the drug-loading ratio, 1mg of the final freeze-dried nano dispersion was dissolved in 1mL of dichloromethane. The drug entrapment was determined by high performance liquid chromatography (HPLC). The mobile phase consisted of methanol and 5% (w/v) acetic acid, in the volume ratio of 70:30, and was delivered at a flow rate of 1.0 mL/minute using a double-reciprocating pump. The analysis wavelength was at 420 nm (Knaure, Berlin, Germany). A C18 (ODS) analytical column (250 mm*4.6 mm, particle size 5 µm; Perfectsill, MZ-Analyse technik, Germany) equipped with a guard column was used. The calibration curve for curcumin was linear, with a concentration range of 0.0016 - 0.125 mg/mL. Encapsulation efficiency was obtained using the following Equation:

2.4.5. Extraction Recovery
Three samples with concentrations of 0.0016, 0.06, and 0.125 mg/mL (from high, middle, and low regions of the standard curve) were prepared in triplicate and analyzed by the developed HPLC method. Next, the ratio of the recorded peak heights to the peak heights resulting from the direct injection of the aqueous solutions of curcumin with the same concentrations were determined as a percentage.
2.4.6. FT-IR and DSC Analysis
DSC analysis was performed to provide further information on the drug-copolymer relationship using a differential scanning calorimeter. Approximately 10 mg of crude CUR powder or lyophilized NPs (with or without drug) was sealed in the standard aluminum pan, purged in the calorimeter with pure dried nitrogen gas set at a flow rate of 10mL/min, and heated at a constant rate of 10°C/minute from 0°C to 200°C. The FT-IR spectra of native CUR and lyophilized NPs (with or without drug) were obtained by using potassium bromide pellets on the FT-IR spectrometer, over the range of 4000 - 400 cm-1.
2.5. Drug release Study to in Vitro
The profile release of curcumin from the nanoparticles to in vitro was determined by the direct dispersion method, as described in the literature (22, 24). Two milliliters of phosphate-buffered saline (PBS, pH 7.4) was added to each vial containing 5mg of freeze-dried powder of nanoparticles, and the resulting dispersion was placed within a dialysis sac (Mw 12 kDa) and incubated at 37°C while immersed in 15 mL of PBS. At scheduled time intervals (0, 1, 2, 4, 6, 8, 10, 12, 24, 48, 72, 96, 120, 144, and 168 hours), 1 mL of samples were withdrawn and replaced by 1mL fresh PBS. All of the release studies were carried out in triplicate. The concentration of curcumin in the dialysate was determined by HPLC, and the analysis wavelength was at 420 nm.
3. Results
3.1. Synthesis and Characterization of PCL-PEG-PCL Copolymer
Triblock copolymers were successfully synthesized using the ring-opening polymerization of caprolactone in the presence of PEG, whose hydroxyl end group initiated the ring opening (22). Characterization of the PCL-PEG-PCL triblock copolymer structure was evaluated by HNMR spectroscopy in CDCl3 (Figure 1). The presence of ethylene (CH2) in PCL was observed around 1.25 ppm, 1.59 ppm, 2.31 ppm, and 4.04 ppm; the methylene (CH2) groups of PEG were around 3.67 ppm. Triblock copolymers of different molecular weights were obtained by controlling the ε-CL:PEG ratio. Characteristics of the synthesized copolymers are shown in Table 1. The sharp and intense bands at 1725 cm-1 and 1109 cm-1 were attributed to the presence of carboxylic ester (C=O) and ether (C–O) groups. The signals at 2995 and 2891 cm-1 were assigned to the absorption of the C-H stretching bonds of -CH2CH2, which are similar to those of ε-CL. The PCL-PEG-PCL copolymer formation was carried out successfully (Figure 2).
HNMR Spectrum of PCL-PEG-PCL Copolymer
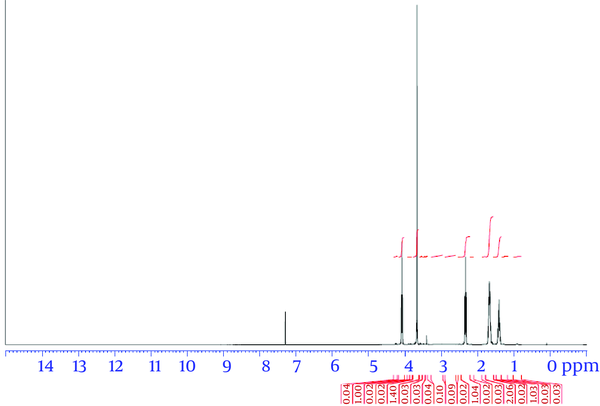
Molecular Characteristics of the Synthesized Copolymers
FT-IR Spectrum of PCL-PEG-PCL Copolymer
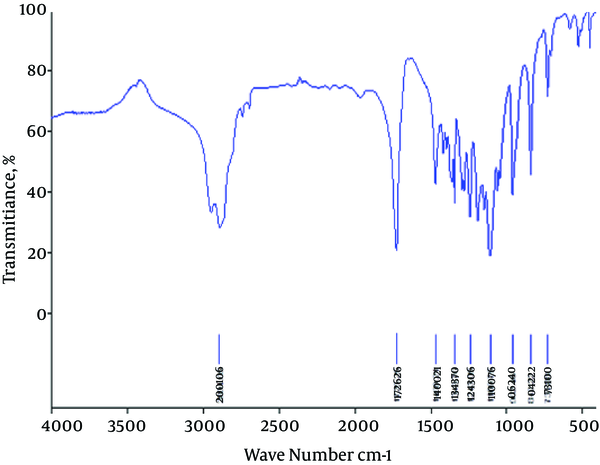
For GPC analysis, the single peak indicated the mono-distribution of macromolecular weight and absence of any homopolymer ε-CL or PEG and showed that no trans-esterification or backbiting reactions occurred during polymerization (25, 26). The results, designated in weight- and number-based average molecular weights of copolymers, are shown in Table 1.
3.2. Preparation and Characterization of Copolymeric Nanoparticles
Curcumin was encapsulated into PCL-PEG-PCL nanoparticles by a self-assembly method producing CUR/PCL-PEG-PCL nanoparticles (Figure 3) (22). AFM was used for confirmation of nanoparticles. As expected for the NPs, the PCL-PEG-PCL copolymeric nanoparticles showed a homogeneous spherical morphology. The size of copolymeric micelle, measured by AFM, was approximately 80 nm (Figure 4). The size of the NPs measured by AFM compared with DLS measurements was smaller, because the size measured by AFM is related to the collapsed nanoparticles after water evaporation, while micelle diameter measured by DLS represents their hydrodynamic diameter (27). The size of the NPs was measured by DLS technique (Figure 5). The Z-average and zeta potential of curcumin-loaded nanoparticles were determined at approximately 110nm and -5.58 mv, respectively, with their corresponding PDI being 0.181. The encapsulation efficiency and loading ratio of curcumin loaded to copolymeric NPs were determined by HPLC at 83 ± 1.29% and 17% ± 1.23%, respectively (Table 2: NPs 9 and Table 3: NPs 5). The HPLC chromatogram of curcumin-loaded nanoparticles is presented in Figure 6.
Scheme of Formation of Nanoparticles
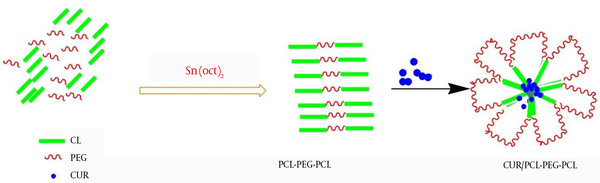
Three-Dimensional Graph of AFM Nanoparticles
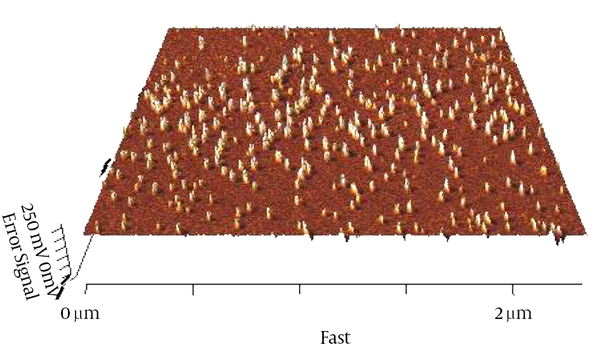
Particle Size Distribution and Zeta Potential of CUR/PCL-PEG-PCL Nanoparticles
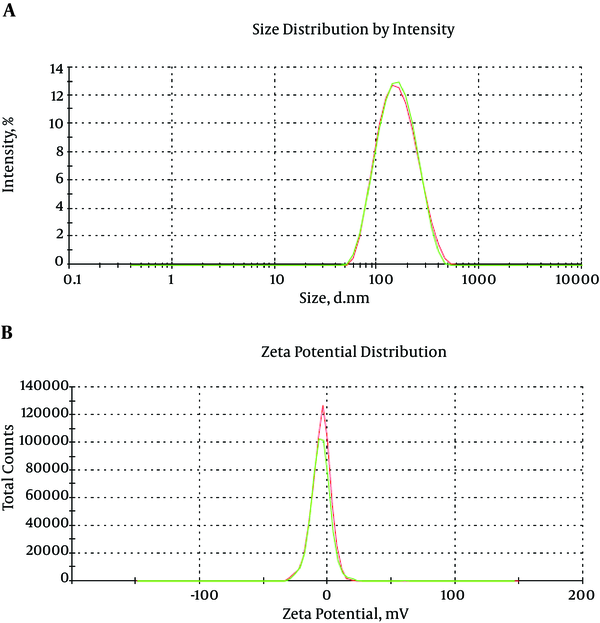
Properties of Curcumin-Loaded PCL-PEG-PCL Nanoparticles
Nanoparticles | CUR /Copolymer Mass Ratio | EE, % | DL, % | Size, nm | Zeta | PDI | Stability |
---|---|---|---|---|---|---|---|
6 | 0 | 0 | 0 | 101 | -4.01 | 0.112 | Yes |
7 | 0.05 | 43 | 11 | 104 | -3.2 | 0.103 | Yes |
8 | 0.1 | 56 | 12 | 103 | -1.7 | 0.131 | Yes |
9 | 0.25 | 83 | 17 | 110 | -5.58 | 0.181 | Yes |
10 | 0.5 | 65 | 18 | 234 | -1.1 | 0.312 | Yes |
11 | 0.75 | 56 | 18.1 | 345 | -3.1 | 0.423 | No |
12 | 1 | 48 | 17.4 | 376 | -4.5 | 0.435 | No |
Characteristics of Nanoparticles from the Synthesized Copolymers
Nanoparticles | CL / EG Feed | EE, % | DL, % | Size, nm | Zeta |
---|---|---|---|---|---|
1 | 0.5 | 47 | 9.8 | 96 | -4.2 |
2 | 1 | 53 | 11 | 101 | -3.1 |
3 | 2 | 60 | 13 | 98.09 | -4.12 |
4 | 4 | 71 | 17.1 | 112 | -5.09 |
5 | 5 | 83 | 17 | 110 | -5.58 |
HPLC Chromatogram of A, Curcumin-Loaded Micelles; B, Curcumin Standard, and C, Blank
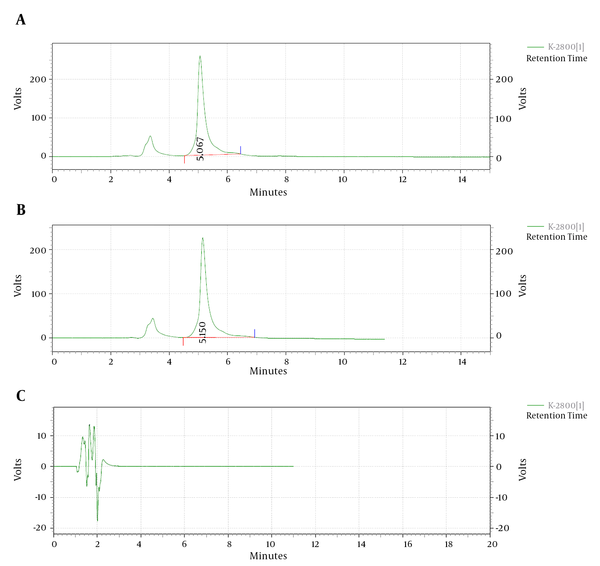
3.3. Effect of the Different Drug-to-Polymer Ratios on Drug Encapsulation Efficiency of NPs
To optimize the progression parameters and impending into the impact of the drug/copolymer ratio on loading and encapsulation efficiency, different CUR/MPEG-PCL mass ratios in the feed were evaluated in our study. Particle size and polydispersity index (PDI) also increased, but the stability of the CUR/MPEG-PCL nanoparticles decreased. When the CUR/MPEG-PCL mass ratio was 0.75 and 1, the obtained CUR/MPEG-PCL nanoparticles were not stable in water (micelles tended to aggregate, forming aggregates); thus, the CUR/MPEG-PCL mass ratio at 0.25 was chosen in our study (Table 2). The interpretation can be assigned in conditions of insolubility of CUR in the aqueous solution, which could allow more drugs at high concentration to be absorbed on the surface of NPs.
3.4. Impact of Copolymer Composition on Drug Entrapment Efficiency
To develop an ideal formulation of higher DL or EE, five series of nanoparticulate CUR (based on PCL-PEG-PCL) were synthesized, and the effects of the ratio of PCL and PEG on DL and EE were studied. The DL and EE of NP1-5 are listed in Table 3. In NPs with fixed PEG length, the DL and EE increased with the rise of feed weight ratio of PEG:ε-CL (from 1:0.5 to 1:5). The maximum EE reached 71% and 83% for NP4 and NP5, respectively. Amphiphilic PCL-PEG-PCL copolymer formed NPs with a core-shell structure in water, where the core and shell were a hydrophobic PCL chain and a hydrophilic PEG chain, respectively. The hydrophobic drug could then be encapsulated into a hydrophobic core by means of the hydrophobic interaction of PCL and CUR and, with the interaction between water and the hydrophilic shell of PEG, the drug was dissolved in water. The increase of the DL and EE of CUR in PCL-PEG-PCL may be caused by the strong hydrophobic interaction between the longer hydrophobic PCL block chain and hydrophobic drug. After DL, the size of the NPs increased. This result was expected, because incorporation of CUR into the hydrophobic cores increased the volume of the NPs.
3.5. Relative Recovery (Matrix Effect)
The extraction recovery determined for curcumin was shown to be consistent, precise, and reproducible. Data is shown in Table 4. This data indicates that there is no significant matrix effect on the outputs of the assay method (Figure 6): HPLC chromatogram of A, curcumin-loaded micelles, B, curcumin standard, and C, blank. The total run time of the HPLC chromatogram was 20 minutes.
Relative Recovery of Curcumin by the HPLC Method, N = 3
Nominal Added Concentration, mg/mL | Amount Found Concentration, mg/mL | Sample Number | Recovery Percent, % | Mean (SD) | CV% |
---|---|---|---|---|---|
0.0016 | 0.00157 | 1 | 98.15 | 95.78 (2.47) | 2.58 |
0.00149 | 2 | 93.21 | |||
0.00153 | 3 | 95.98 | |||
0.06 | 0.0560 | 1 | 93.42 | 94.67 (1.58) | 1.67 |
0.0578 | 2 | 96.45 | |||
0.0564 | 3 | 94.15 | |||
0.125 | 0.114 | 1 | 91.60 | 93.47 (1.80) | 1.93 |
0.119 | 2 | 95.21 | |||
0.117 | 3 | 93.61 |
3.6. FT-IR Analysis
Interaction of copolymer with drug by FT-IR spectrum approved to the pervious our works (22). For the FT-IR spectrum of curcumin, characteristic bands appeared at 3515.77 cm-1 (O-H, stretching) for the phenolic hydroxyl group, 1630.97 cm-1 (C=C, stretching), 1512.94 cm-1 (C=C band of benzene), and 856.38 cm-1(C-H, stretching of aromatic ring). FT-IR spectra of nanoparticles was the blue shift of the C=O vibration, from 1725.25 to 1730.56 cm-1 for drug-loaded PCL-PEG-PCL nanoparticles, compared to the copolymer spectrum (Figure 2). The shift in the nanoparticles spectrum indicates that there exist some form(s) of association between curcumin and the C=O functional group of copolymer (28-30). Comparing this data with the drug-loaded nanoparticles spectrum exhibits the presence of curcumin characteristic peaks in the spectrum of nanoparticles, which could demonstrate the successful loading of curcumin in the nanoparticles.
3.7. DSC Analysis
Figure 7 shows the DSC thermograms corresponding to the PCL-PEG-PCL copolymer, curcumin, and nanoparticles loaded by curcumin (22). The thermogram of the PCL-PEG-PCL copolymer displayed an endothermic peak at 58.68°C, which is indicative of the melting of the crystalline PCL segment of the copolymer. The thermogram of curcumin displayed an endothermic peak at 176.71°C, and the nanoparticles displayed two endothermic peaks at 49.09°C and 175.55°C, which indicates the melting of the copolymer and curcumin association in the form of nanoparticles. As the melting point of PCL nanoparticles was lower than the melting point of both copolymer (58.68°C) and pure curcumin (176.71°C), the endothermic peak of the nanoparticles presumably confirms a physical interaction between the copolymer and curcumin upon loading of the drug into the nanoparticles.
DSC Spectra of A, PCL-PEG-PCL; B, Curcumin, and C, CUR/PCL-PEG-PCL Nanoparticles
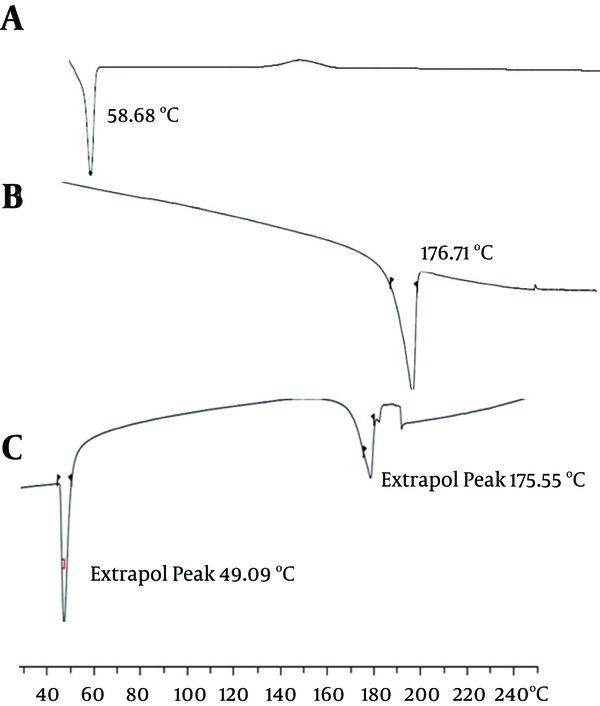
3.7.1. Curcumin Release from Nanoparticles Impacted by Different Copolymer Composition
The in vitro release behaviors of CUR solution and CUR-NPs in buffered solution (pH = 7.4) are representatively shown in Figure 8. In a releasing medium of pH = 7.4, rapid release was observed in the controlled solution, with 78% of CUR released in 12 hours. The typical two-phase release profile was observed for CUR release from NP3, NP4, and NP5; that is, a relatively rapid release in the first stage, followed by a sustained and slow release rate over a prolonged time of up to 168 hours. Overall, they showed a slower release than that of the control solution. At 120 hours, only 65.0%, 57.3%, and 51% of the CUR was released from NP3, NP4, and NP5, respectively. It seems that the release rate of CUR from drug-loaded polymeric NPs decreased as the molecular weight of PCL-PEG-PCL in pH 7.4 buffers increased. As the length of the hydrophobic ester chain in NP5 was longer than that in NP4 and NP3, its hydrolysis was slower, which led to the slower release of CUR.
The Release Profiles of Curcumin from Different CUR/PCL-PEG-PCL Nanoparticles in Media with PH = 7.4
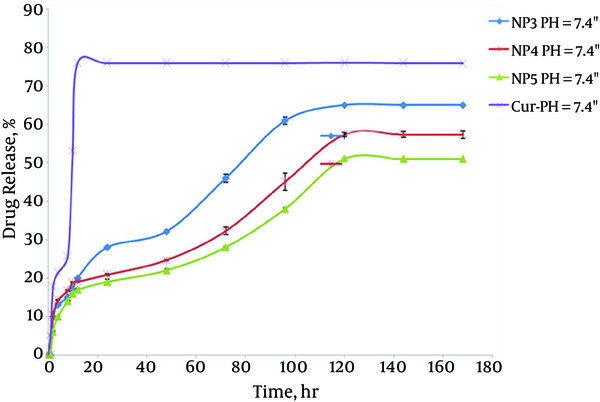
4. Discussion
Curcumin is a promising agent for anticancer therapy. However, CUR is a lipophilic drug and is insoluble in water, which limits its clinical application. Encapsulation by nanoparticles appears to be a brilliant advance to conquer the hydrophobicity of drugs. There are many encapsulation methods, such as spray-drying and solvent removal, to encapsulate CUR into micelles or liposomes (31, 32). In this study, we used PCL-PEG-PCL micelles to encapsulate CUR. In the training procedure, a CUR and PCL-PEG-PCL triblock copolymer mixture was first dissolved in chloroform solution, followed by evaporating the organic solvent. The CUR/PCL-PEG-PCL nanoparticles showed high encapsulation efficiency, making this an aqueous formulation of curcumin. In addition, the CUR/PCL-PEG-PCL nanoparticles had a negative surface charge of -5.58 mV, which increased the circulation time of the drug. Surface charge is important in determining whether the nanoparticles will cluster in blood flow or will adhere to or interact with an oppositely charged cell membrane.
This study explored the effect of the length of hydrophilic and hydrophobic chains on the encapsulation of hydrophilic CUR to obtain an appropriate sustained-release property for CUR in vitro. The results suggest that CUR-loaded NP5 is The Cur /PCL-PEG-PCL nanoparticles were able to slowly release curcumin (Figure 8). The sustained release of curcumin from PCL-PEG-PCL nanoparticles might be due to the diffusion of curcumin from the nanoparticles and the degradation or hydrolysis of the nanoparticles. These PCL-PEG-PCL nanoparticles are biodegrad¬able, biocompatible, amphiphilic, stable in blood, nontoxic, non-immunogenic, non-inflammatory, and small in size. These characteristics make PCL-PEG-PCL nanoparticles an excellent candidate for drug-delivery systems.
4.1. Conclusion
This study shows that PCL-PEG-PCL copolymeric nanoparticles are suitable for delivery of curcumin. The curcumin-loaded nanoparticles had spherical structure, with excellent size and zeta potential. The encapsulation efficiency and loading efficiency of curcumin to the nanoparticles was very noticeable. In vitro release of curcumin from curcumin-entrapped nanoparticles was clearly sustained in all the media tested for this purpose, with the apparent release plateau reached late, at about 120 hours. The CUR/PCL-PEG-PCL nanoparticles improved the water solubility of curcumin and may have potential application in cancer treatment. In summary, curcumin was effectively entrapped by the prepared nanoparticles, which could improve the solubility of curcumin and prolong its retention in the systemic circulation.
Acknowledgements
References
-
1.
Sun YM, Zhang HY, Chen DZ, Liu CB. Theoretical elucidation on the antioxidant mechanism of curcumin: a DFT study. Org Lett. 2002;4(17):2909-11. [PubMed ID: 12182586].
-
2.
Kohli K, Ali J, Ansari MJ, Raheman Z. Curcumin: a natural antiinflammatory agent. Ind J Pharmacol. 2005;37:141-77.
-
3.
Panchatcharam M, Miriyala S, Gayathri VS, Suguna L. Curcumin improves wound healing by modulating collagen and decreasing reactive oxygen species. Mol Cell Biochem. 2006;290(1-2):87-96. [PubMed ID: 16770527]. https://doi.org/10.1007/s11010-006-9170-2.
-
4.
Sun A, Shoji M, Lu YJ, Liotta DC, Snyder JP. Synthesis of EF24-tripeptide chloromethyl ketone: a novel curcumin-related anticancer drug delivery system. J Med Chem. 2006;49(11):3153-8. [PubMed ID: 16722634]. https://doi.org/10.1021/jm051141k.
-
5.
Somasundaram S, Edmund NA, Moore DT, Small GW, Shi YY, Orlowski RZ. Dietary curcumin inhibits chemotherapy-induced apoptosis in models of human breast cancer. Cancer Res. 2002;62(13):3868-75. [PubMed ID: 12097302].
-
6.
Aggarwal BB, Shishodia S, Takada Y, Banerjee S, Newman RA, Bueso-Ramos CE, et al. Curcumin suppresses the paclitaxel-induced nuclear factor-kappaB pathway in breast cancer cells and inhibits lung metastasis of human breast cancer in nude mice. Clin Cancer Res. 2005;11(20):7490-8. [PubMed ID: 16243823]. https://doi.org/10.1158/1078-0432.CCR-05-1192.
-
7.
Aggarwal BB, Kumar A, Bharti AC. Anticancer potential of curcumin: preclinical and clinical studies. Anticancer Res. 2003;23(1A):363-98. [PubMed ID: 12680238].
-
8.
Letchford K, Liggins R, Burt H. Solubilization of hydrophobic drugs by methoxy poly(ethylene glycol)-block-polycaprolactone diblock copolymer micelles: theoretical and experimental data and correlations. J Pharm Sci. 2008;97(3):1179-90. [PubMed ID: 17683080]. https://doi.org/10.1002/jps.21037.
-
9.
Anand P, Kunnumakkara AB, Newman RA, Aggarwal BB. Bioavailability of curcumin: problems and promises. Mol Pharm. 2007;4(6):807-18. [PubMed ID: 17999464]. https://doi.org/10.1021/mp700113r.
-
10.
Lin CC, Lin HY, Chen HC, Yu MW, Lee MH. Stability and characterisation of phospholipid-based curcumin-encapsulated microemulsions. Food Chemistry. 2009;116(4):923-8.
-
11.
Anitha A, Maya S, Deepaa N, Chennazhi KP, Nair SV, Tamura H. Efficient water soluble O-carboxymethyl chitosan nanocarrier for the delivery of curcumin to cancer cells. Carbohydr Polym. 2011;83:452-61.
-
12.
Anitha A, Deepagan VG, Rani VVD, Menon D, Nair SV, Jayakumar R. Preparation, characterization, in vitro drug release and biological studies of curcumin loaded dextran sulphate–chitosan nanoparticles. Carbohydrate Polymers. 2011;84(3):1158-64.
-
13.
Avgoustakis K, Beletsi A, Panagi Z, Klepetsanis P, Livaniou E, Evangelatos G, et al. Effect of copolymer composition on the physicochemical characteristics, in vitro stability, and biodistribution of PLGA-mPEG nanoparticles. Int J Pharm. 2003;259(1-2):115-27. [PubMed ID: 12787641].
-
14.
Shieh YA, Yang SJ, Wei MF, Shieh MJ. Aptamer-based tumor-targeted drug delivery for photodynamic therapy. ACS Nano. 2010;4(3):1433-42. [PubMed ID: 20166743]. https://doi.org/10.1021/nn901374b.
-
15.
Danafar H, Rostamizadeh K, Davaran S, Hamidi M. Drug-conjugated PLA-PEG-PLA copolymers: a novel approach for controlled delivery of hydrophilic drugs by micelle formation. Pharm Dev Technol. 2016:1-11. [PubMed ID: 26740008]. https://doi.org/10.3109/10837450.2015.1125920.
-
16.
Wei X, Gong C, Gou M, Fu S, Guo Q, Shi S, et al. Biodegradable poly(epsilon-caprolactone)-poly(ethylene glycol) copolymers as drug delivery system. Int J Pharm. 2009;381(1):1-18. [PubMed ID: 19664700]. https://doi.org/10.1016/j.ijpharm.2009.07.033.
-
17.
Gou M, Wei X, Men K, Wang B, Luo F, Zhao X, et al. PCL/PEG copolymeric nanoparticles: potential nanoplatforms for anticancer agent delivery. Curr Drug Targets. 2011;12(8):1131-50. [PubMed ID: 21443476].
-
18.
Danafar H, Davaran S, Rostamizadeh K, Valizadeh H, Hamidi M. Biodegradable m-PEG/PCL Core-Shell Micelles: Preparation and Characterization as a Sustained Release Formulation for Curcumin. Adv Pharm Bull. 2014;4(Suppl 2):501-10. [PubMed ID: 25671181]. https://doi.org/10.5681/apb.2014.074.
-
19.
Shi HS, Gao X, Li D, Zhang QW, Wang YS, Zheng Y, et al. A systemic administration of liposomal curcumin inhibits radiation pneumonitis and sensitizes lung carcinoma to radiation. Int J Nanomedicine. 2012;7:2601-11. [PubMed ID: 22679371]. https://doi.org/10.2147/IJN.S31439.
-
20.
Danafar H, Sharafi A, Kheiri Manjili H, Andalib S. Sulforaphane delivery using mPEG-PCL co-polymer nanoparticles to breast cancer cells. Pharm Dev Technol. 2016:1-10. [PubMed ID: 26916923]. https://doi.org/10.3109/10837450.2016.1146296.
-
21.
Men K, Gou ML, Guo QF, Wang XH, Shi S, Kan B, et al. A novel drug and gene co-delivery system based on Poly(epsilon-caprolactone)-Poly(ethylene glycol)-Poly(epsilon-caprolactone) grafted polyethyleneimine micelle. J Nanosci Nanotechnol. 2010;10(12):7958-64. [PubMed ID: 21121283].
-
22.
Manjili HK, Sharafi A, Danafar H, Hosseini M, Ramazani A, Ghasemi MH. Poly (caprolactone)–poly (ethylene glycol)–poly (caprolactone)(PCL–PEG–PCL) nanoparticles: a valuable and efficient system for in vitro and in vivo delivery of curcumin. RSC Advances. 2016;6(17):14403-15.
-
23.
Danafar H, Rostamizadeh K, Davaran S, Hamidi M. PLA-PEG-PLA copolymer-based polymersomes as nanocarriers for delivery of hydrophilic and hydrophobic drugs: preparation and evaluation with atorvastatin and lisinopril. Drug Dev Ind Pharm. 2014;40(10):1411-20. [PubMed ID: 23944838]. https://doi.org/10.3109/03639045.2013.828223.
-
24.
Piao L, Dai Z, Deng M, Chen X, Jing X. Synthesis and characterization of PCL/PEG/PCL triblock copolymers by using calcium catalyst. Polymer. 2003;44(7):2025-31.
-
25.
Bisht S, Feldmann G, Soni S, Ravi R, Karikar C, Maitra A, et al. Polymeric nanoparticle-encapsulated curcumin ("nanocurcumin"): a novel strategy for human cancer therapy. J Nanobiotechnology. 2007;5:3. [PubMed ID: 17439648]. https://doi.org/10.1186/1477-3155-5-3.
-
26.
Choi HS, Liu W, Misra P, Tanaka E, Zimmer JP, Itty Ipe B, et al. Renal clearance of quantum dots. Nat Biotechnol. 2007;25(10):1165-70. [PubMed ID: 17891134]. https://doi.org/10.1038/nbt1340.
-
27.
Song Z, Feng R, Sun M, Guo C, Gao Y, Li L, et al. Curcumin-loaded PLGA-PEG-PLGA triblock copolymeric micelles: Preparation, pharmacokinetics and distribution in vivo. J Colloid Interface Sci. 2011;354(1):116-23. [PubMed ID: 21044788]. https://doi.org/10.1016/j.jcis.2010.10.024.
-
28.
Danafar H, Hamidi M. Simple and Sensitive High-Performance Liquid Chromatography (HPLC) Method with UV Detection for Mycophenolic Acid Assay in Human Plasma. Application to a Bioequivalence Study. Adv Pharm Bull. 2015;5(4):563-8. [PubMed ID: 26819930]. https://doi.org/10.15171/apb.2015.076.
-
29.
Mohanty C, Sahoo SK. The in vitro stability and in vivo pharmacokinetics of curcumin prepared as an aqueous nanoparticulate formulation. Biomaterials. 2010;31(25):6597-611. [PubMed ID: 20553984]. https://doi.org/10.1016/j.biomaterials.2010.04.062.
-
30.
Abouzid KA, Khalil NA, Ahmed EM, Zaitone SA. Synthesis and biological evaluation of new heteroaryl carboxylic acid derivatives as anti-inflammatory-analgesic agents. Chem Pharm Bull (Tokyo). 2013;61(2):222-8. [PubMed ID: 23370197].
-
31.
Sen GS, Mohanty S, Hossain DM, Bhattacharyya S, Banerjee S, Chakraborty J, et al. Curcumin enhances the efficacy of chemotherapy by tailoring p65NFkappaB-p300 cross-talk in favor of p53-p300 in breast cancer. J Biol Chem. 2011;286(49):42232-47. [PubMed ID: 22013068]. https://doi.org/10.1074/jbc.M111.262295.
-
32.
Kumari A, Yadav SK, Yadav SC. Biodegradable polymeric nanoparticles based drug delivery systems. Colloids Surf B Biointerfaces. 2010;75(1):1-18. [PubMed ID: 19782542]. https://doi.org/10.1016/j.colsurfb.2009.09.001.