Abstract
Background:
Thymoquinone (TQ), a bioactive compound, has many pharmacological actions especially anticancer effects. Prostate cancer (PC) is one of the most common malignancies among males in the world.Objectives:
The current study aimed at evaluating the effects of TQ treatment on interleukin-6 (IL-6) expression, IL-6 secretion, cell viability, phosphorylated extracellular signal–regulated kinases (pERK1/2), phosphorylated AKT (pAKT), and phosphorylated signal transducer and activator of transcription 3 (pSTAT3) signaling pathways in human prostate cancer PC3 cells.Materials and Methods:
PC3 cells were cultured in different concentrations of TQ for 48 hours. Cell viability, expression, and secretion of IL-6 were assessed by MTT assay, real-time quantitative polymerase chain reaction (RT-q PCR) analysis, and the enzyme-linked immunosorbent assay (ELISA) technique, respectively. The changes of pERK1/2, pAKT, and pSTAT3 were determined using Western blot procedure.Results:
Treatment with TQ caused the reduction of viability (inhibitory concentration [IC50] = 45 µM) and survival in PC3 cells. RT-q PCR had a significant reduction (P < 0.05) in IL-6 gene expression compared with the control cells (50%, 59%, 68%, and 70% at 30, 40, 45, and 50 µM of TQ, respectively). TQ treatment resulted in a significant decline (P < 0.05) in the secretion of IL-6 compared with the control cells (56.89%, 71.12%, 84.32%, and 85.89% at 30, 40, 45, and 50 µM of TQ, respectively). Western blot showed a reduction in the phosphorylated STAT3, AKT, and ERK proteins.Conclusions:
The obtained results suggested that TQ can effectively reduce IL-6 signaling pathways (pSTAT3, pAKT, and pERK1/2). Also, TQ can decrease IL-6 gene expression, which leads to the reduction of cell proliferation and viability. Therefore, TQ may be beneficial to treat prostate cancer.Keywords
Nigella Sativa pERK1/2 pAKT pSTAT3 Antioxidant Thymoquinone Interleukin-6 PC3 cells
1. Background
Prostate cancer (PC), an androgen-sensitive cancer, is one of the most common malignancies among males in the United States and western world (1, 2). Recently, 233,000 new PC cases are reported by American Cancer Society statistics in 2014 and it is estimated that about 29,480 patients are expected to die from it (3). The geographical distribution of PC varies in the world to the extent that in Asian countries, including Iran, it is lower than the Western countries (4). There are a few studies on the epidemiology of PC in Iran (5), where it is the 3rd most common type of cancer in males and the 6th most prevalent cancer (6). Unfortunately, PC is highly resistant to chemotherapy in advanced stages of the disease and its progression depends on both genetic and epigenetic factors. Androgen ablation therapy is mainly used to treat hormone-sensitive PC. However, in most males usually after 2 to 3 years, metastatic PC leads to an androgen-independent state (7-9). It is shown that signaling pathways influence cell growth and its inhibition through cell cycle regulation (10). Several studies confirmed the role of interleukin-6 (IL-6, a glycoprotein including 212 amino) in the etiology and progression of PC (10-12). IL-6 plays an important role in the cellular growth of PC3 cells through its effects on the various signaling pathways such as Janus kinase (JAK), signal transducer and activator of transcription 3 (STAT3), and extracellular signal–regulated kinase 1/2 (ERK1/2) (11-13).
Nowadays, medicinal plants are used to treat a variety of diseases due to their flavonoids (14-16). Furthermore, there is a growing interest in naturally-occurring compounds with anticancer potentials. Thymoquinone (2-isopropyl-5-methyl-1,4-benzoquinone) (TQ), a bioactive compound isolated from Nigella sativa essential oil, has many anticancer effects including cell cycle arrest, antiproliferation, apoptosis, induction ROS (reactive oxygen species) generation, antimetastasis, and blockade of tumor angiogenesis in various cancers. In addition, some antitumor effects of TQ on colon, prostate, pancreatic, and lung cancers are reported (17, 18). TQ has relatively low toxicity as it is a well-tolerated agent in rodents (19). TQ exhibits its anticancer activity by modulating various molecular targets including activation of several caspases, induction of ROS generation, and cell signaling STAT3 (18, 20). Several studies explained the anticancer effects of TQ attributed to the induction of PPAR-γ (peroxisome proliferator-activated receptor gamma), PTEN (phosphatidylinositol 3, 4, 5-trisphosphate 3-phosphatase) expression, as well as p53-dependent and independent apoptosis. Also, TQ is an upregulator Bax/Bcl-2 ratio, caspase-9/3, smooth muscle actin, p53, and p21, while it is a downregulator of the pro-apoptotic genes including Bcl-2, Bcl-xL, and cell surviving (19, 21, 22).
2. Objectives
The current study aimed at evaluating the effects of TQ treatment on IL-6 gene expression, cell viability, IL-6 secretion, pERK1/2, pAKT, and pSTAT3 signaling pathways in human PC3 cells.
3. Materials and Methods
The human PC cell line, PC3, was purchased from Pasteur Institute of Iran (Tehran, Iran). Thymoquinone was obtained from Santa Cruz Biotechnology Inc. (Santa Cruz, CA, USA). Biozol total RNA extraction reagent was purchased from BioFlux (BioFlux, Japan). Dimethyl sulfoxide (DMSO), trypan blue, Pen/Strep, and 3-(4, 5-dimethylthiaztol-2-yl)-2, 5-diphenyltetrazolium bromide (MTT) were purchased from Sigma (St. Louis, MO) and RPMI1640 medium and fetus bovine serum (FBS) from Gibco (Rockville, MD, USA). Antibodies were purchased from Abcam (San Francisco, CA, USA). All other chemicals were of analytical grade.
3.1. Cell Culture and MTT Assay
Prostate cancer PC3 cells were seeded at 5000 cells/cm2 onto 96-well plates, in RPMI-1640 supplemented with 10% FBS and 1% penicillin/streptomycin at 37°C in a humidified 5% CO2 incubator (23). The cells were allowed to attach for 24 hours before treatment with TQ. After 24 hours, culture media was removed from the plate and replaced with media containing different concentrations of TQ (0, 30, 40, 45, and 50 µM) solved in dimethyl sulfoxide (0.1% final concentration in media) for 48 hours. The experiments were repeated 3 times for each concentration. Then, the cytotoxicity effects of TQ on PC3 cells were assessed by MTT assay (24, 25). Briefly, after treating cells for 48 hours, the culture medium was removed and cells were exposed to 100 µL colorless RPMI and 10 µL MTT (5 mg/mL in phosphate buffered saline (PBS) added to each well and incubated for 4 hours. The medium was removed, and 150 µL DMSO was added to solubilize the formazan crystals. Absorbance of formazan solution was evaluated using a plate reader (Stat Fax 3200, Awareness Technology, USA) at 490 nm (3). The percentage viability was calculated as follows:
Percentage viability = A (sample)/ A (control) × 100.
3.2. Real-Time Quantitative Polymerase Chain Reaction for IL-6
Prostate cancer PC3 cells in the exponential growth phase were treated with different concentrations of TQ (0, 30, 40, 45, and 50 µM) in a 6-well plate for 48 hours. Then, the media were removed and the total RNA of the cells was extracted by Biozol reagent according to the manufacturer’s instructions. Total RNA were measured by 260/280 nm absorbance ratio (3) using the Nanodrop-2000 Spectrophotometer (Thermo-USA). The procedure of cDNA reverse transcription was carried out using PrimeScriptTM reagent Kit (Takara Bio Inc.) according to the manufacturer's instructions. Then, cDNA was amplified by RT-q PCR using SYBR® Green PCR Master Mix (Qiagen, Germany) in the presence of specific primers for IL-6 (forward: 5'-AAGCCAGAGCTGTGCAGATGAGTA-3'; reverse: 5' TGTCCTGCAGCCACTGGTTC -3') and GAPDH (forward: 5´ACACCCACTCCTCCACCTTTG-3'; reverse: 5'- CCACCACCCTGTTGCTGTAG-3'). The primers were designed using Oligo 6.0 software (Molecular Biology Insights Inc., Cascade, CO), confirmed by blast (NCBI) and purchased from Eurogentec (Seraing, Belgium). The IL-6 gene expression was determined using Rotor-Gene 3000 (Corbett, Australia) for each mentioned concentration. Amplification involved a first denaturation at 95°C for 10 minutes, RT-q PCR was down for 35 cycles in a 3-step program (including 15 seconds at 95°C, 20 seconds at 62°C, and 25 seconds at 72°C). A housekeeping gene, glyceraldehyde-3-phosphate dehydrogenase (GAPDH), was used as an endogenous control for values of mRNA expression and normalizing the data.
3.3. Western Blotting
PC3 cells were cultured in RPMI 1640 medium with various concentrations of TQ (0, 30, 45, and 50 µM) for 48 hours. Then, the whole-cell proteins were extracted using RIPA buffer (50 mM Tris-HCl, pH 7.5, 150 mM NaCl, 1% Triton X-100, 0.5% sodium deoxycholate, 1 mM ethylenediaminetetraacetic acid (EDTA), 0.1% sodium azide, 50 mM NaF, and 0.1% sodium dodecyl sulfate (SDS), and 1 mM phenylmethanesulfonyl fluoride (PMSF) (26). Protein concentrations were determined in supernatant by Nanodrop-2000 Spectrophotometer. Protein lysates prepared with an equal volume of loading buffer (0.125 M Tris-HCl, 4% SDS, 20% glycine, and 10% 2-mercaptoethanol) and boiled for 5 minutes. Equal amounts of denatured proteins were subjected to 10% SDS–polyacrylamide gel electrophoresis (SDS-PAGE). Pre-stained blue protein markers (Bio-Rad) were used to determine molecular-weight. The gels were transferred and blotted onto polyvinylidene difluoride membranes (PVDF). Then, the membranes were placed into a blocking buffer containing 5% skim milk powder in TBS-Tween buffer (10 mM Tris, 100 mM NaCl, 0.1 mM Tween-20), and pH 7.4 for overnight at 4°C (26). The membranes were rinsed 3 times in TBS-Tween buffer for 10 minutes and subjected to primary antibodies against phosphorylated STAT3, phosphorylated p44/42 ERK1/2, and phosphorylated AKT at room temperature for 3 hours. The membranes were rinsed with TBS-Tween buffer 3 times for 10 minutes and incubated with an appropriate dilution of horseradish peroxidase-conjugated secondary antibody at room temperature for 2 hours. After rinsing the membranes 3 times for 10 minutes in TBS-Tween buffer, they were visualized using Western blotting BM Blue POD substrate; β-actin was assessed as an internal control.
3.4. Measurement of Il-6
PC3 cells were seeded in regular culture medium at a density of 2 × 105 cell/well in a 6-well plate at 37°C in a 5% CO2 incubator. Then, the cells were treated with different concentrations of TQ (0, 30, 45, and 50 µM) for 48 hours. The concentrations of secreted IL-6 protein in the supernatants were assessed using the enzyme-linked immunosorbent assay (ELISA) technique (AViBion Human IL-6 ELISA kit) according to the manufacturer’s instructions.
3.5. Statistical Analysis
Statistical analysis was carried out with SPSS version 20.0 (SPSS, Chicago, IL). Data were expressed as mean ± standard deviation (SD) for each replicated treatment. For expression analysis, the relative levels of quantitative gene expression were calculated with the 2−ΔΔCt method and data were expressed as fold change. P values < 0.05 were considered significant. Western blot experiments were repeated 3 times.
4. Results
4.1. Effects of TQ on PC-3 Cell Viability
TQ decreased the cell viability gradually with increased concentration of TQ (Figures 1 and 2) after 48 hours. In the current study, the IC50 value of TQ was calculated at 45 μM.
4.2. Effect of TQ on the Expression of IL-6 in PC3 Cells
Treated PC3 cells with/without TQ were analyzed for IL-6 gene expression using RT-q PCR. The expression of IL-6 in the treated PC3 cells after 48 hours with TQ significantly downregulated (P < 0.05) in a dose-dependent manner (Table 1) with respect to the control cells. There was a significant decline (P < 0.05) in IL-6 expression by almost 50%, 59%, 68%, and 70% at 30, 40, 45, and 50 µM of TQ concentrations, respectively in TQ-treated PC-3 cells compared with the control cells.
Effect of Thymoquinone on the Secretion and Expression of IL-6 in PC3 Cells
Inhibitory Effects of TQ on PC3 Cell Viability
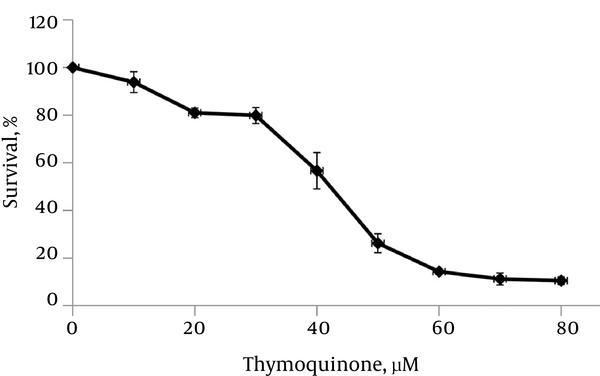
Effects of TQ on Morphological Changes and Proliferation of PC3 Cells
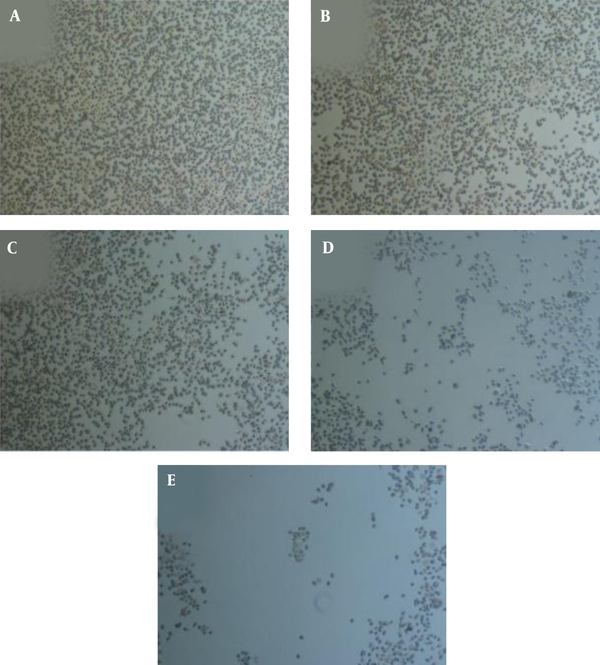
Effects of TQ on the Level of Cellular Signaling Pathways in PC3 Cells
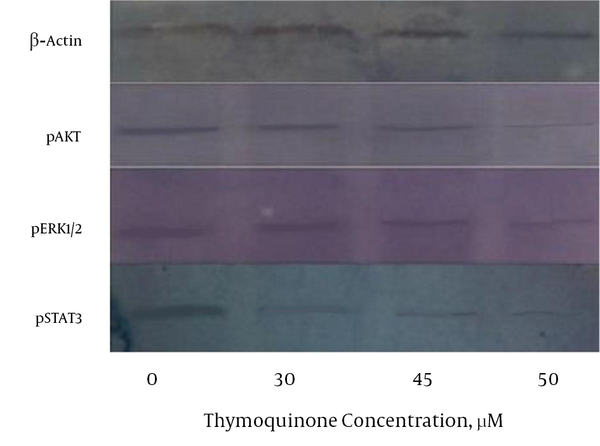
4.3. Effects of TQ on the Cellular Signaling Pathways in PC3 Cells
The Western blot data showed that PC3 treated cells with TQ resulted in a moderate to strong reduction of the cytoplasmic phosphorylated STAT3, ERK1/2, and AKT levels in a dose-dependent pattern after treatment with different doses of TQ for 48 hours (Figure 3). TQ resulted in the strong reduction of pSTAT3 and pERK1/2 at above 30 µM concentrations; however, TQ had moderate reduction of AKT at above 30 µM concentrations.
4.4. Effect of TQ on IL-6 Secretion
The IL-6 secretion by TQ-treated PC3 cells is shown in Table 1. TQ significantly resulted in a salient reduction (P < 0.05) of IL-6 in PC3 cells media in a dose-dependent manner compared with the control cells (56.89%, 71.12%, 84.32%, and 85.89% at 30, 40, 45, and 50 µM of TQ, respectively).
5. Discussion
Many studies showed that IL-6 protein has a central role in the development of PC by affecting cellular regulatory signaling pathways (11, 12). Previous studies showed that TQ exhibited many biological effects including antioxidant, anti-inflammatory, antitumor, and particularly antiproliferative activities on PC cells (17, 18, 27). In the current study, TQ resulted in the decrease of PC3 cells viability and proliferation (Figures 1 and 2). Thus, TQ had strong potential antiproliferative effects on PC3 cells as reported by other investigators (28). On the other hand, TQ treatment of PC3 cells led to a reduction of IL-6 protein secretion by PC3 cells (Table 1). Also in the current study, results of RT-q PCR confirmed the reduction of IL-6 expression due to TQ treatment (Table 1), which was in accordance with the reduction of IL-6 protein secretion by PC3 cells. Previous investigations demonstrated that IL-6 protein plays a major role in the promotion, growth, and proliferation of PC3 cells (10, 12). Therefore, in the current study the reduction of viability and proliferation of PC3 cells may be due to the effects of TQ on decreasing IL-6 cytokine production. Many published data showed that TQ led to the reduction of the number of cytokines including IL-4, IL-5, IL-13, attenuated lipopolysaccharides (LPS)-induced IL-1b, tumor necrosis factor alpha (TNF-a), matrix metalloproteinase (MMP)-13, cyclooxygenase-2, and prostaglandin E2 (10, 29). It is reported that IL-6, due to its receptor, uses many cellular mediators and transducers such as Janus kinase-signal transducers and activators of transcription (JAK-STAT), phosphorylated ERK1/2 (T202/Y204), and phospho-AKT (S473) for its signaling pathways (11, 13). In the current study, treatment of PC3 cells with TQ led to the reduction of pAKT, pSTAT3, and ERK1/2 protein signaling pathways (Figure 3), which were in line with those reported in other studies (11, 13). According to the reported studies, STAT3 regulates the transcription of genes involved in cell differentiation, inflammation, proliferation, apoptosis, angiogenesis, metastasis, tumor cell transformation, immune responses, and chemoresistance of tumor cells (11, 13, 30), which can be a reason for the reduction of cell proliferation and viability in the current study. Also, overexpression of antiapoptotic genes such as Bcl-2 and Bcl-XL is associated with constitutive STAT3 activity. Then, STAT3 inhibition induces apoptosis (31). Therefore, in the current study decreased IL-6 and pSTAT3 may be, at least in part, a strong potential to reduce viability and proliferation of PC3 cells, which is in agreement with the results of Li et al., who found that TQ downregulated the expression of STAT3 (32). Also, previous studies demonstrated that TQ suppresses IL-6-inducible STAT3 phosphorylation in U266 multiple myeloma cells (32). On the other hand, AKT is implicated in the progression of many human cancers, because it controls several factors involved in the apoptosis and cell cycle progression (33), which is in agreement with the findings of the current study. Also, ERK is a key regulatory kinase to control endothelial cell cycle, proliferation, growth, migration, and apoptosis in various types of cancers (34, 35). Therefore in the current study, another possible mechanism for antiproliferative effects of TQ on PC3 cells, at least in part, can be applied by reducing pAKT signaling pathway. Also, the ERK1/2 MAPK pathway (depending on the individual ligand, cell surface receptor, and cell type) is involved in the invasive, proliferation, differentiation, survival, metastatic, apoptosis, and development of several cancers through the phosphorylation of both cytoplasmic and nuclear targets including phosphatases, transcriptional factors, and cytoskeletal proteins (36, 37). TQ can prevent proliferation and angiogenesis by suppressing ERK and AKT phosphorylation in human umbilical vein endothelial cells (HUVECs). The current study findings demonstrated that pERK1/2 signaling pathways were diminished by TQ (Figure 3), which was in line with previously published papers (34, 35, 38). Thus, additional mechanism for antiproliferative effects of TQ on PC3 cells may be applied by inhibiting pERK1/2 signaling pathway.
The current study did not evaluate the effect of TQ on other protein signaling pathways such as the nuclear translocation level of p53, caspase3, and regulatory effectors such as Bcl2 level, Bax level, etc. These factors can influence cell apoptosis and survival. Therefore, it was suggested that future studies focus on the effects of TQ on other cell signaling and effector proteins.
In conclusion, the current study results demonstrated that inhibition of IL-6 signaling by TQ treatment may be a beneficial way to reduce pSTAT3, pAKT, and pERK1/2 signaling pathways in PC3 cells, which leads to the reduction of cell proliferation and viability.
Acknowledgements
References
-
1.
Rasul A, Di J, Millimouno FM, Malhi M, Tsuji I, Ali M, et al. Reactive oxygen species mediate isoalantolactone-induced apoptosis in human prostate cancer cells. Molecules. 2013;18(8):9382-96. [PubMed ID: 23921797]. https://doi.org/10.3390/molecules18089382.
-
2.
Siegel R, Naishadham D, Jemal A. Cancer statistics for Hispanics/Latinos, 2012. CA Cancer J Clin. 2012;62(5):283-98. [PubMed ID: 22987332]. https://doi.org/10.3322/caac.21153.
-
3.
American Cancer Society. Cancer Facts & Figures 2014. 2014. Available from: http://www.cancer.org/acs/groups/content/@research/documents/webcontent/acspc-042151.pdf.
-
4.
Ferlay J, Soerjomataram I, Dikshit R, Eser S, Mathers C, Rebelo M, et al. Cancer incidence and mortality worldwide: sources, methods and major patterns in GLOBOCAN 2012. Int J Cancer. 2015;136(5):E359-86. [PubMed ID: 25220842]. https://doi.org/10.1002/ijc.29210.
-
5.
Kolahdoozan S, Sadjadi A, Radmard AR, Khademi H. Five common cancers in Iran. Arch Iran Med. 2010;13(2):143-6. [PubMed ID: 20187669].
-
6.
Pakzad R, Rafiemanesh H, Ghoncheh M, Sarmad A, Salehiniya H, Hosseini S, et al. Prostate Cancer in Iran: Trends in Incidence and Morphological and Epidemiological Characteristics. Asian Pac J Cancer Prev. 2016;17(2):839-43. [PubMed ID: 26925689].
-
7.
Vlachostergios PJ, Papandreou CN. Targeting neuroendocrine prostate cancer: molecular and clinical perspectives. Front Oncol. 2015;5:6. [PubMed ID: 25699233]. https://doi.org/10.3389/fonc.2015.00006.
-
8.
Saraon P, Musrap N, Cretu D, Karagiannis GS, Batruch I, Smith C, et al. Proteomic profiling of androgen-independent prostate cancer cell lines reveals a role for protein S during the development of high grade and castration-resistant prostate cancer. J Biol Chem. 2012;287(41):34019-31. [PubMed ID: 22908226]. https://doi.org/10.1074/jbc.M112.384438.
-
9.
de Leeuw R, Berman-Booty LD, Schiewer MJ, Ciment SJ, Den RB, Dicker AP, et al. Novel actions of next-generation taxanes benefit advanced stages of prostate cancer. Clin Cancer Res. 2015;21(4):795-807. [PubMed ID: 25691773]. https://doi.org/10.1158/1078-0432.CCR-14-1358.
-
10.
Nguyen DP, Li J, Tewari AK. Inflammation and prostate cancer: the role of interleukin 6 (IL-6). BJU Int. 2014;113(6):986-92. [PubMed ID: 24053309]. https://doi.org/10.1111/bju.12452.
-
11.
Eskandari E, Heidarian E, Amini SA, Saffari-Chaleshtori J. Evaluating the effects of ellagic acid on pSTAT3, pAKT, and pERK1/2 signaling pathways in prostate cancer PC3 cells. J Cancer Res Therapeut. 2016;12(4):1266.
-
12.
Culig Z. Proinflammatory cytokine interleukin-6 in prostate carcinogenesis. Am J Clin Exp Urol. 2014;2(3):231-8. [PubMed ID: 25374925].
-
13.
Santoni M, Massari F, Del Re M, Ciccarese C, Piva F, Principato G, et al. Investigational therapies targeting signal transducer and activator of transcription 3 for the treatment of cancer. Expert Opin Investig Drugs. 2015;24(6):809-24. [PubMed ID: 25746129]. https://doi.org/10.1517/13543784.2015.1020370.
-
14.
Lin PH, Aronson W, Freedland SJ. Nutrition, dietary interventions and prostate cancer: the latest evidence. BMC Med. 2015;13:3. [PubMed ID: 25573005]. https://doi.org/10.1186/s12916-014-0234-y.
-
15.
Heidarian E, Saffari J, Jafari-Dehkordi E. Hepatoprotective action of Echinophora platyloba DC leaves against acute toxicity of acetaminophen in rats. J Diet Suppl. 2014;11(1):53-63. [PubMed ID: 24409975]. https://doi.org/10.3109/19390211.2013.859217.
-
16.
Heidarian E, Rafieian-Kopaei M. Protective effect of artichoke (Cynara scolymus) leaf extract against lead toxicity in rat. Pharm Biol. 2013;51(9):1104-9. [PubMed ID: 23745593]. https://doi.org/10.3109/13880209.2013.777931.
-
17.
Salem AA, El Haty IA, Abdou IM, Mu Y. Interaction of human telomeric G-quadruplex DNA with thymoquinone: a possible mechanism for thymoquinone anticancer effect. Biochim Biophys Acta. 2015;1850(2):329-42. [PubMed ID: 25450185]. https://doi.org/10.1016/j.bbagen.2014.10.018.
-
18.
Woo CC, Kumar AP, Sethi G, Tan KH. Thymoquinone: potential cure for inflammatory disorders and cancer. Biochem Pharmacol. 2012;83(4):443-51. [PubMed ID: 22005518]. https://doi.org/10.1016/j.bcp.2011.09.029.
-
19.
Islam MT, Sultana N, Riaz TA, Ferdous J, Guha B, Mohagon S, et al. Thymoquinone is knocking at the door of clinical trial. Int Arch Med. 2016;9.
-
20.
Darakhshan S, Pour AB, Colagar AH, Sisakhtnezhad S. Thymoquinone and its therapeutic potentials. Pharmacol Res. 2015;95:138-58.
-
21.
Parbin S, Shilpi A, Kar S, Pradhan N, Sengupta D, Deb M, et al. Insights into the molecular interactions of thymoquinone with histone deacetylase: evaluation of the therapeutic intervention potential against breast cancer. Mol Biosyst. 2016;12(1):48-58. [PubMed ID: 26540192]. https://doi.org/10.1039/c5mb00412h.
-
22.
Woo CC, Loo SY, Gee V, Yap CW, Sethi G, Kumar AP, et al. Anticancer activity of thymoquinone in breast cancer cells: possible involvement of PPAR-gamma pathway. Biochem Pharmacol. 2011;82(5):464-75. [PubMed ID: 21679698]. https://doi.org/10.1016/j.bcp.2011.05.030.
-
23.
Pandurangan AK. Potential targets for prevention of colorectal cancer: a focus on PI3K/Akt/mTOR and Wnt pathways. Asian Pac J Cancer Prev. 2013;14(4):2201-5. [PubMed ID: 23725112].
-
24.
Woo CC, Hsu A, Kumar AP, Sethi G, Tan KH. Thymoquinone inhibits tumor growth and induces apoptosis in a breast cancer xenograft mouse model: the role of p38 MAPK and ROS. PLoS One. 2013;8(10). e75356. [PubMed ID: 24098377]. https://doi.org/10.1371/journal.pone.0075356.
-
25.
Heidarian E, Keloushadi M, Ghatreh-Samani K, Valipour P. The reduction of IL-6 gene expression, pAKT, pERK1/2, pSTAT3 signaling pathways and invasion activity by gallic acid in prostate cancer PC3 cells. Biomed Pharmacother. 2016;84:264-9. [PubMed ID: 27665471]. https://doi.org/10.1016/j.biopha.2016.09.046.
-
26.
Hwang SL, Yen GC. Effect of hesperetin against oxidative stress via ER- and TrkA-mediated actions in PC12 cells. J Agric Food Chem. 2011;59(10):5779-85. [PubMed ID: 21486081]. https://doi.org/10.1021/jf104632a.
-
27.
Harzallah HJ, Kouidhi B, Flamini G, Bakhrouf A, Mahjoub T. Chemical composition, antimicrobial potential against cariogenic bacteria and cytotoxic activity of Tunisian Nigella sativa essential oil and thymoquinone. Food Chem. 2011;129(4):1469-74.
-
28.
Koka PS, Mondal D, Schultz M, Abdel-Mageed AB, Agrawal KC. Studies on molecular mechanisms of growth inhibitory effects of thymoquinone against prostate cancer cells: role of reactive oxygen species. Exp Biol Med (Maywood). 2010;235(6):751-60. [PubMed ID: 20511679]. https://doi.org/10.1258/ebm.2010.009369.
-
29.
Vaillancourt F, Silva P, Shi Q, Fahmi H, Fernandes JC, Benderdour M. Elucidation of molecular mechanisms underlying the protective effects of thymoquinone against rheumatoid arthritis. J Cell Biochem. 2011;112(1):107-17. [PubMed ID: 20872780]. https://doi.org/10.1002/jcb.22884.
-
30.
Johnston PA, Grandis JR. STAT3 signaling: anticancer strategies and challenges. Mol Interv. 2011;11(1):18-26. [PubMed ID: 21441118]. https://doi.org/10.1124/mi.11.1.4.
-
31.
Siddiqui WA, Ahad A, Ahsan H. The mystery of BCL2 family: Bcl-2 proteins and apoptosis: an update. Arch Toxicol. 2015;89(3):289-317. [PubMed ID: 25618543]. https://doi.org/10.1007/s00204-014-1448-7.
-
32.
Li F, Rajendran P, Sethi G. Thymoquinone inhibits proliferation, induces apoptosis and chemosensitizes human multiple myeloma cells through suppression of signal transducer and activator of transcription 3 activation pathway. Br J Pharmacol. 2010;161(3):541-54. [PubMed ID: 20880395]. https://doi.org/10.1111/j.1476-5381.2010.00874.x.
-
33.
Shukla S, Bhaskaran N, Babcook MA, Fu P, Maclennan GT, Gupta S. Apigenin inhibits prostate cancer progression in TRAMP mice via targeting PI3K/Akt/FoxO pathway. Carcinogenesis. 2014;35(2):452-60. [PubMed ID: 24067903]. https://doi.org/10.1093/carcin/bgt316.
-
34.
Surmacz E, Otvos L. Molecular targeting of obesity pathways in cancer. Horm Mol Biol Clin Investig. 2015;22(2):53-62. [PubMed ID: 25879324]. https://doi.org/10.1515/hmbci-2015-0007.
-
35.
da Silva MR, Moreira GA, Goncalves da Silva RA, de Almeida Alves Barbosa E, Pais Siqueira R, Teixera RR, et al. Splicing Regulators and Their Roles in Cancer Biology and Therapy. Biomed Res Int. 2015;2015:150514. [PubMed ID: 26273588]. https://doi.org/10.1155/2015/150514.
-
36.
Munkley J, Lafferty NP, Kalna G, Robson CN, Leung HY, Rajan P, et al. Androgen-regulation of the protein tyrosine phosphatase PTPRR activates ERK1/2 signalling in prostate cancer cells. BMC Cancer. 2015;15:9. [PubMed ID: 25592066]. https://doi.org/10.1186/s12885-015-1012-8.
-
37.
Robertson BW, Bonsal L, Chellaiah MA. Regulation of Erk1/2 activation by osteopontin in PC3 human prostate cancer cells. Mol Cancer. 2010;9:260. [PubMed ID: 20868520]. https://doi.org/10.1186/1476-4598-9-260.
-
38.
Yi T, Cho SG, Yi Z, Pang X, Rodriguez M, Wang Y, et al. Thymoquinone inhibits tumor angiogenesis and tumor growth through suppressing AKT and extracellular signal-regulated kinase signaling pathways. Mol Cancer Ther. 2008;7(7):1789-96. [PubMed ID: 18644991]. https://doi.org/10.1158/1535-7163.MCT-08-0124.