Abstract
Background:
Development of a new iron-chelating medication may eventually serve as treatment of iron overload diseases, such as thalassemia and other iron related disorders. Coriander sativum, a plant rich of phenol and flavonoids, has an iron-chelating effect in-vitro. The ethanolic extract of C. sativum was investigated for its in-vivo ameliorating effect on hepatic damage in rats with experimental iron overload.Methods:
Twenty-four adult male wistar rats were randomly divided to 4 groups: healthy control, iron overload, iron overload treated with deferoxamine, and 200 mg/kg of C. sativum extract, respectively. Assessments were performed for serum iron, ferritin, and serum markers of hepatic and cardiac damages, antioxidant enzyme, and lipid peroxidation levels.Results:
The results indicated serum iron and ferritin concentration were significantly increased in the iron overload group compared to healthy controls (P < 0.05). Treatment with the C. sativum extract significantly decreased iron and ferritin concentration (P < 0.05). Histopathological changes in the liver, kidney, and the cardiac system, and iron accumulation in the liver were observed in the iron overload group compared to healthy controls (P < 0.05). Treatment with C. sativum extract significantly decreased biochemical parameters, such as alanine aminotransferase (ALT), aspartate aminotransferase (AST), alkaline phosphatase (ALP), lactate dehydrogenase (LDH) and creatine phosphokinase (CPK), and improved tissue damage and decreased iron accumulation in the liver compared to the iron overload group (P < 0.05).Conclusions:
The study showed that the hydro-alcoholic extract of C. sativum has iron-chelating and liver protective effects similar to deferoxamine against experimental iron overload condition in rats.Keywords
Iron Overload Anti-Toxicity Hydro-Alcoholic Extra Coriander sativum
1. Background
Physiologically, iron serves several crucial roles in life. However, an excess of iron in most organs is toxic, mostly by increasing oxidative stress and creating reactive oxygen species, which in turn cause peroxidation of lipids, proteins, and nucleic acids. Liver is the target organ for iron toxicity because it is predominantly responsible for taking out and storing excessive amounts of iron (1, 2). When the amount of iron in circulation is more than the transferrin binding capacity, iron toxicity occurs. Excessive accumulation of free iron in the liver causes damage, including hepatocellular necrosis (3), inflammation (4), fibrosis (5, 6), and even carcinoma (7). Thus, in all iron overload diseases, treatment with an iron-chelating agent is a common method for reducing iron toxicity in all organisms.
Nowadays, synthetic iron-chelating compounds, including deferoxamine and deferiprone L1, are commonly used and quite effective in the treatment of iron overload diseases. However, they have several side effects and disadvantages (8-10). Moreover, deferoxamine is poorly absorbed orally, has a slow clearance and requires slow subcutaneous administration or intravenous infusion, resulting in low patient compliance (11, 12). Conversely, herbal medicines are frequently less toxic with fewer side effects. Therefore, search for a non-toxic and orally active iron-chelating agent, which is a more effective and safe treatment for thalassemia and other iron-overload associated disorders, is a new approach for research (13-15).
Coriandrum sativum L, a culinary and medical herb, is an annual small plant that belongs to the Apiaceae family and is commonly known as coriander. The leaves, roots and seeds of C. sativum are rich of aromatic savor and are generally used in soups in Thai and other Asian cooking. Traditionally, all parts of the plant are used to alleviate gastrointestinal complaints, bronchitis, muscular spasm, gout, and rheumatoid disorders (16). Several studies performed on this herb reported that the plant has various therapeutic properties, including, anti-microbial, anti-hyperlipidemia, hepato-protective, anti-peroxidative, insulin-releasing, and insulin-like activity (17-20). Various polyphenol and flavonoid compounds, including glycitin, caffeic acid, protocatechuic acid, apigenin, Aliphatic (2E)-alkenals, and alkanals were reported in the aerial parts of C. sativum (21-23) whereas, Quercetin 3-glucuronide, isoquercitrin, and rutin were found in the fruit (24). Recently, several novel phytochemical and pharmacological studies have been performed on C. sativum and results have been reported in review articles (20, 25). However, studies have always focused on the roots and aerial parts of C. sativum for their antioxidant properties (22-24). Research on the therapeutic properties of C. sativum is rare and regarding its iron-chelating effect, only limited in-vitro studies are available. Mirzaei and Khatami reported that coriander is a powerful iron chelator plant in in-vitro conditions, also, they found a positive correlation between phenolic and flavonoid content of the plant and iron chelating effects (15). The aim of this study was to investigate the iron-chelating and anti-toxicity effects of hydro-alcoholic extract of C. sativum in rats with experimental iron-overload condition.
2. Objectives
The current study aimed at evaluating the iron chelating, anti-toxicity, and liver protective potentials of hydro-alcoholic extract of C. sativum in rats with iron-overload condition.
3. Methods
3.1. Chemicals
Iron dextran was purchased from Sterop Co., Belgium. Deferoxamine was purchased from Novartis Co., Switzerland. For measurement of alanine aminotransferase (ALT), aspartate aminotransferase (AST), alkaline phosphatase (ALP) and lipid profile, commercial available kits were purchased from Pars Azmoon Co, Tehran, Iran.
3.2. Plant Collection, Authentication, and Preparation of Hydro alcoholic Extract
Coriander sativum was collected from the local area of Behbahan, south-west of Iran, during September to October, 2014. A voucher specimen of the plant was stored in the herbarium of medicinal plants and natural products research center, Ahvaz Jundishapur University of Medical Sciences, Ahvaz, Iran (Herbarium No.A150100400AP). The pharmacological parts of C. sativum are its leaves, seeds, and stem. In the current study, the whole plant was dried at room temperature in the shade, and then powdered. Afterwards, the powder was soaked in 1000-mL solution containing 70% ethanol and 30% water for 72 hours (the solution was gently shacked every 12 hours). Then, the solution was filtered using a Buchner funnel and liquid was separated by a rotary evaporator system (26). Finally, the extract was dried by a freezer dryer. For experimental use, the extract was dissolved in normal saline before gavage every day.
3.3. Induction of Iron Overload
Twenty-four adult male Wistar rats, weighing 220 to 250 g, were purchased from the animal house central of Ahvaz Jundishapur University of Medical Sciences, Ahvaz, Iran. Rats were kept under standard stainless cages during the study period. Dark-light period was set for 12/12 hours and the room temperature was about 22 to 25°C. Rats were fed rodent standard food with free access to water. All animal experiments were performed according to the animal care guidelines and were approved by the animal ethics committee of Ahvaz Jundishapur University of Medical Sciences, Ahvaz, Iran. For induction of iron overload, the rats were treated with iron-dextran (100mg/kg body weight) through intraperitoneal injection once each two days, during a two-week intervention. Healthy control rats were treated with an equal volume of normal saline at the same time. The experimental animals were divided to 4 groups (n=6), and were treated as follows:
Group 1, Healthy control treated with normal saline
Group 2, Iron overload treated with iron dextran (100 mg/kg once every 2 days during 2 weeks intraperitoneally (IP))
Group 3, Iron overload treated with deferoxamine (50 mg/kg once every 2 days during two weeks IP)
Group 4: Iron overload treated with C. sativum extract (200mg/kg extract for 14 days)
3.4. Biochemical Measurements
At the end of the experimental period, the fasted rats were anesthetized with an intraperitoneal injection of ketamine and xylazine, and fresh blood samples were directly collected via cardiac puncture using not heparinized syringes. Serum iron concentration, liver biomarkers, including alanine aminotransferase (ALT), aspartate aminotransferase (AST), alkaline phosphatase (ALP), lactate dehydrogenase (LDH), and creatine phosphokinase (CPK) were measured using commercial available kits (Pars Azmoon Co.Tehran, Iran) by Biotecnica BT-3000 plus Chemistry Analyzer, Italy. For measurement of iron concentration, first ferric carrier protein, transferrin, will release ferric ion into the solution at acid buffer. After reduction of ferric (Fe3+) to the ferrous form (Fe2+), iron reacts with Ferene S to generate a long-standing colored complex and provide absorbance at 600 nm. The assay system for measuring ALT and AST activity consisted of two amino acids and two oxo-acids. Continuous monitoring method was used to measure the enzyme activity by two couple enzyme reactions. The oxo-acids produced in each enzyme reaction was measured indirectly by enzymatic reduction to corresponding hydroxy acids, and the accompanying changes in NADH concentration was monitored spectrophotometrically at 340 nm. The ALP activity was measured based on production of 4-nitrofenoxide (a yellow quinonoid form) from its common substrate p-nitrophenyl phosphate at alkaline PH with a colorimetric method. Total LDH activity was measured using the kinetic spectrophotometry method to measure the conversion of reduced coenzyme NADH to oxidized NAD+ at 340 nm. Two coupled enzyme systems were used for determination of CPK activity. The enzyme catalyzes the conversion of creatine phosphate to creatine accompanied by phosphorylation of ADP to ATP. The produced ATP is consumed by coupled enzyme, glucose-6-phosphate dehydrogenase (G6PD), which finally converts NADP+ to NADPH, monitored with a spectrophotometer at 340 nm. Serum ferritin level was also measured by a mini vidas auto analyzer using the enzyme-linked fluorescent assay technique. The assay system mixes a one-step sandwich enzyme immunoassay with a final fluorescent detection assay. At the end of the assay, results were automatically reported by the instrument in relation to the standard curve.
3.5. Liver Tissue Preparations
After collection of the blood sample, all animals were sacrificed and their livers were quickly removed and weighted, and then perfused with normal saline (0.9%) to exclude RBC contamination. Half of them were cut, weighed, and homogenized with a homogenizer (Heidolph Silentcrosher M, Germany) in potassium phosphate buffer (0.1 M with PH = 7.4). To remove additional components (cell debris), the homogenized compound was centrifuged (16000 g, 15 minutes) and the clear supernatant was used for the assessment of tissue content of Malondialdehyde (MDA) and catalase activity.
3.6. Estimation of Tissue Catalase Activity and Lipid Peroxidation Content
Catalase (CAT) activity of liver tissue was measured spectrophotometrically at 240 nm, according to Claiborne (27). Briefly, 1 mL of the final reaction mixture was prepared consisting of 50 mM potassium phosphate (pH 7.0), 19 mM H2O2, and 50 µL of the sample. After addition of H2O2, the reaction was started, and absorbance changes were measured at 240 nm for 30 seconds. The specific activity of CAT was expressed as unit mg-1 tissue.
Tissue lipid peroxides content was determined as Thiobarbituric Acid Reactive Substances (TBARS) formation, calculated as malondialdehyde (28). Briefly, 500 µL of supernatant was added to 1.5 mL of trichloroacetic acid and after centrifugation (4,000 × g for 10 minutes), 1.5 mL of the supernatant was mixed with 2 mL of TBA (0.67%) and warmed at 100°C for 30 minutes. After chilling, the samples were extracted with 2 mL n-butanol and then centrifuged (4000 × g for 15 minutes). Finally, the organic phase was collected. The absorbance was measured at 535 nm and the levels were expressed as nmol MDA mg-1 tissue.
3.7. Histopathological Examination
After removal of the liver from rats, a part of the liver was fixed with 10% formalin, embedded in paraffin, and stained with Hematoxylin and Eosin (H and E). In order to examine iron accumulation in the liver, the prepared sections of tissue were stained with Prussian blue (including potassium ferrocyanide 2% and hydrochloridric acid 2%, equally), then microscopic slides were evaluated using a light microscope (Olympus, Tokyo, Japan).
3.8. Statistical Analysis
All statistical analyses were done with the SPSS software (SPSS, Inc., Chicago IL, USA). Continuous variables are expressed as mean ± SD, and were compared by One-Way analysis of variance (ANOVA) followed by Turkey’s post hoc test. The level of significance was adjusted at P < 0.05.
4. Results
4.1. Biochemical Findings
It was shown that iron dextran injection led to iron overload in the rats at the end of experimental period, because of the level of iron and ferritin in serum were increased (390 ± 46.43 and 3.88 ± 0.17, respectively). The treatment with deferoxamine (50 mg/kg, IP) resulted in significant reduction of serum iron and ferritin compared to the iron dextran group (P < 0.05). In addition, oral administration of hydro-alcoholic extract of C. sativum (200 mg/kg) significantly reduced serum iron and ferritin compared to iron overloaded rats (P < 0.05), (Table 1). The mean levels of liver biomarkers including alanine aminotransferase (ALT), aspartate aminotransferase (AST), and alkaline phosphatase (ALP) in different studied groups after 14 days of treatment are presented in Table 2. The results showed that induction of iron overload led to increase in serum levels of ALT, AST and ALP in iron overloaded rats compared to healthy controls (P < 0.05). The injection of deferoxamine significantly decreased the serum level of AST and ALT (P < 0.05), yet changes in the serum levels of ALP was not statistically significant. Also, treatment with the extract decreased serum level of liver biomarkers toward a normal range, so, these changes were significant for AST and ALP compared to iron overloaded rats and those treated with deferoxamine (P < 0.05). Moreover, the results indicated that the mean values of malondialdehyde (MDA) and catalase (CAT) activity in the liver homogenate were increased in the disease control group compared to the healthy controls. Also, a significant decrease in the mean values of malondialdehyde (MDA) and catalase (CAT) activity was observed in the iron overload and treated with deferoxamine rats compared to the iron overload rats. In addition, oral administration of hydro-alcoholic extract of C. sativum (200 mg/kg) significantly decreased both of them compared to healthy controls and iron overloaded rats (Table 3). In order to determine the effect of hydro-alcoholic extract of C. sativum on cardiac function, serum activity of lactate dehydrogenase (LDH) and creatine phosphokinase (CPK) were measured and results are presented in Table 4. In iron overload, after treatment with iron dextran, increased serum levels of these cardiac biomarkers was observed. Although, after treatment with deferoxamine, LDH was significantly decreased, yet, reduction of CPK was not statistically significant (Table 4). Moreover, in iron overload treated with C. sativum extract, significantly decreased serum level of LDH and CPK was observed compared to iron overload and healthy controls, respectively (P < 0.05).
The Mean Values of Serum Iron and Ferritin in the Studied Groupsa
The Mean Values of Liver Biomarkers in the Studied Groupsa
The Mean Values of Liver Malondialdehyde Content and Catalase Activity in the Studied Groupsa
The Mean Values of Serum Creatine Phosphokinase and Lactate Dehydrogenase Activity in the Studied Groupsa
4.2. Histophatological Findings
Histopathological results in iron overload group indicated dramatic changes in the liver, including, loss of radial arrangement, sinusoid dilation, cell vacuolation, and high accumulation of iron in the hepatocytes when compared to the healthy control group. However, in the group treated with deferoxamine and the group treated with hydroalcoholic extract of C. sativum these changes were less than the iron overload group (Figures 1 and 2).
The Protective Effects of Hydroalcholic Extract of C. Sativum on the Iron Overloaded Induced Liver Complications
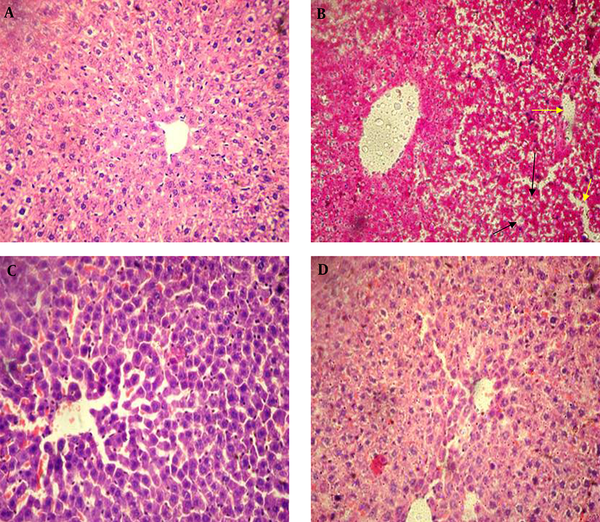
The Protective Effects of Hydroalcholic Extract of C. Sativum on the Liver Iron Accumulation
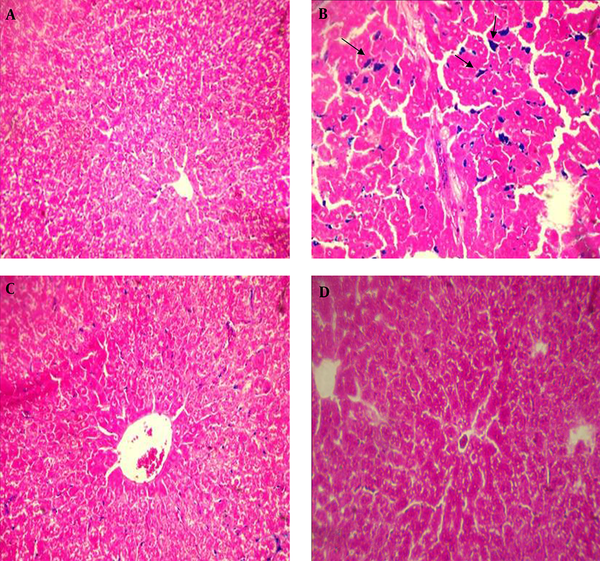
5. Discussion
Clinically, acute iron overload toxicity is one of the most common metal toxicities. The role of iron in the development of hepatic injuries in several clinical and experimental conditions has commonly been studied by induction of an iron overload condition (29-33).
The current results indicated that in rats with iron overload, serum iron and ferritin concentration dramatically increased, while the oral administration of hydro-alcoholic extract of C. sativum significantly decreased the iron and ferritin concentration. Treatment of experimental animals with iron-dextran, similar to hemochromatosis, resulted in, iron loaded anemia (34). In order to exclude the probability of iron chelation before adsorption or direct hindrance of absorption by extract in the intestine, it was preferred to use IP injection of iron dextran for iron-overload induction. Since, the liver is the main organ for iron storage, liver damage was significant in the iron overload condition (35). Studies have also shown that iron overload causes liver damage, such as fibrosis and even cirrhosis. In addition, increased lipid peroxidation during liver damage was reported (29, 30, 36). It has been demonstrated that imbalance between oxidant and antioxidant factors, occurred in the iron overload condition, which resulted in reduction of enzyme activity such as catalase (31, 34). In the current study, iron overload resulted in various adverse effects, such as increase in ALT, AST, and ALP activity and MDA level, as well as decreased CAT activity, which indicates liver damage. These changes may be the result of the production of ROS and oxidative damage by hepatic iron accumulation, which, finally, may result in chronic diseases (30) and leakage of cellular enzymes into the circulation due to increased liver membrane permeability (31, 34). However, after treatment with hydro-alcoholic extract of C. sativum, reduction of theses liver biomarkers was observed. For example, liver histological injuries were significantly alleviated after treatment with the extract, and liver iron content was also decreased. Clinically, advanced lipid peroxidation usually produces several cytotoxic products, such as MDA, which can produce covalent adducts with proteins, phospholipids, and DNA. The formation of MDA-protein adducts have been found in the liver of rats with iron overload, and greater formation of these MDA-macromolecules adducts may be a potential mechanism in iron-induced hepatic damage (31). Thus, inhibition of lipid peroxidation can be one of the major strategies for the treatment of hepatic injuries under iron overload conditions. Nevertheless, according to the current results, catalase activity and malondialdehyde level were not significantly changed after treatment with extract. In order to find such changes in iron overload condition, more intervals for intervention may be required.
Previous studies have shown that toxic level of iron causes several damages on the kidney and heart through increasing the activity of oxidative stress pathway (2, 32). Results reported by Gavin et al. and Shengjiang Guan et al. indicated dysfunction of heart and kidney in iron overload (2, 33). In the current study, after injection of iron dextran, kidney histological damage was observed, yet treatment with the extract of C. sativum improved kidney damage. It has been shown that flavonoids could be interfering with iron absorption and act as an iron chelator compound (37, 38). Previous studies confirmed that the iron-chelating properties of a plant directly related to its flavonoids content (39). Najafzadeh et al. demonstrated that silymarin as a flavonoid could decrease iron overload-induced liver toxicity and its effects were similar to deferoxamine (40). In another study showed that baicalin (a commercial flavonoid) has iron chelating and liver protective effects in iron overload mice (36). Ebrahimzadeh et al. reported that there was an association between iron chelating activity and phenol and flavonoid content of herbs. Thus, plants, such as leonurus cardiana and grammosciadium platycarpum, with the highest phenol and flavonoid contents have more iron chelating effects than other plants (41).
Moreover, the intake of polyphenol-containing beverages has been proposed as a valuable plan to decrease non-haem iron absorption in patients with iron overload disorders (42). Nowadays, all humans use polyphenol compounds in their dietary regime, because most of the beverages and vegetables are rich of these compounds (43, 44). Mirzaei et al. in their study conducted on rats reported that coriander is a potential iron chelator plant. In addition, they found that the plant has antioxidant property and confirmed that these effects may result from high phenols and flavonoids content (15). In addition, Sreelatha et al., reported that injection of 100 and 200 mg/kg of coriander extract is non-toxic and has protective effects on the liver of rats with oxidative stress condition (16).
5.1. Conclusion
To the best of our knowledge, this is the first study to provide information about the iron-chelating effect of C. sativum extract in rats with experimental iron-overload. The results indicated that hydro-alcoholic extract of C. sativum has an iron-chelating and liver protective potential similar to deferoxamine in the experimental iron overload condition. The efficacy of C. sativum as a medicinal plant may be introduced in clinical trial studies. Moreover, to achieve extraction, isolation and characterization of bioactive compounds in the C. sativum extract, further studies are needed.
Acknowledgements
References
-
1.
Kohgo Y, Ikuta K, Ohtake T, Torimoto Y, Kato J. Body iron metabolism and pathophysiology of iron overload. Int J Hematol. 2008;88(1):7-15. [PubMed ID: 18594779]. https://doi.org/10.1007/s12185-008-0120-5.
-
2.
Sengsuk C, Tangvarasittichai O, Chantanaskulwong P, Pimanprom A, Wantaneeyawong S, Choowet A, et al. Association of Iron Overload with Oxidative Stress, Hepatic Damage and Dyslipidemia in Transfusion-Dependent beta-Thalassemia/HbE Patients. Indian J Clin Biochem. 2014;29(3):298-305. [PubMed ID: 24966477]. https://doi.org/10.1007/s12291-013-0376-2.
-
3.
Milic S, Mikolasevic I, Orlic L, Devcic E, Starcevic-Cizmarevic N, Stimac D, et al. The Role of Iron and Iron Overload in Chronic Liver Disease. Med Sci Monit. 2016;22:2144-51. [PubMed ID: 27332079].
-
4.
Wessling-Resnick M. Iron homeostasis and the inflammatory response. Annu Rev Nutr. 2010;30:105-22. [PubMed ID: 20420524]. https://doi.org/10.1146/annurev.nutr.012809.104804.
-
5.
Liu D, He H, Yin D, Que A, Tang L, Liao Z, et al. Mechanism of chronic dietary iron overload-induced liver damage in mice. Mol Med Rep. 2013;7(4):1173-9. [PubMed ID: 23404080]. https://doi.org/10.3892/mmr.2013.1316.
-
6.
Philippe MA, Ruddell RG, Ramm GA. Role of iron in hepatic fibrosis: one piece in the puzzle. World J Gastroenterol. 2007;13(35):4746-54. [PubMed ID: 17729396]. https://doi.org/10.3748/wjg.v13.i35.4746.
-
7.
Kew MC. Hepatic iron overload and hepatocellular carcinoma. Liver Cancer. 2014;3(1):31-40. [PubMed ID: 24804175]. https://doi.org/10.1159/000343856.
-
8.
Alavi S, Sadeghi E, Ashenagar A. Efficacy and safety of combined oral iron chelation therapy with deferasirox and deferiprone in a patient with beta-thalassemia major and persistent iron overload. Blood Res. 2014;49(1):72-3. [PubMed ID: 24724073]. https://doi.org/10.5045/br.2014.49.1.72.
-
9.
Poggiali E, Cassinerio E, Zanaboni L, Cappellini MD. An update on iron chelation therapy. Blood Transfus. 2012;10(4):411-22. [PubMed ID: 22790257]. https://doi.org/10.2450/2012.0008-12.
-
10.
Hatcher HC, Singh RN, Torti FM, Torti SV. Synthetic and natural iron chelators: therapeutic potential and clinical use. Future Med Chem. 2009;1(9):1643-70. [PubMed ID: 21425984]. https://doi.org/10.4155/fmc.09.121.
-
11.
Cappellini MD. Overcoming the challenge of patient compliance with iron chelation therapy. Semin Hematol. 2005;42(2 Suppl 1):S19-21. [PubMed ID: 15846581]. https://doi.org/10.1053/j.seminhematol.2005.01.001.
-
12.
Varzi HN, Esmailzadeh S, Morovvati H, Avizeh R, Shahriari A, Givi ME. Effect of silymarin and vitamin E on gentamicin-induced nephrotoxicity in dogs. J Vet Pharmacol Ther. 2007;30(5):477-81. [PubMed ID: 17803742]. https://doi.org/10.1111/j.1365-2885.2007.00901.x.
-
13.
Taher AT, Porter JB, Viprakasit V, Kattamis A, Chuncharunee S, Sutcharitchan P, et al. Approaching low liver iron burden in chelated patients with non-transfusion-dependent thalassemia: the safety profile of deferasirox. Eur J Haematol. 2014;92(6):521-6. [PubMed ID: 24460655]. https://doi.org/10.1111/ejh.12270.
-
14.
Pandey A, Bigoniya P, Raj V, Patel KK. Pharmacological screening of Coriandrum sativum Linn. for hepatoprotective activity. J Pharm Bioallied Sci. 2011;3(3):435-41. [PubMed ID: 21966166]. https://doi.org/10.4103/0975-7406.84462.
-
15.
Mirzaei A, Khatami R. Antioxidant and iron chelating activity of Coriander sativum and Petroselinum crispum. Bull Environ Pharmacol Life Sci. 2013;2:27-31.
-
16.
Sreelatha S, Padma PR, Umadevi M. Protective effects of Coriandrum sativum extracts on carbon tetrachloride-induced hepatotoxicity in rats. Food Chem Toxicol. 2009;47(4):702-8. [PubMed ID: 19146910]. https://doi.org/10.1016/j.fct.2008.12.022.
-
17.
Matasyoh JC, Maiyo ZC, Ngure RM, Chepkorir R. Chemical composition and antimicrobial activity of the essential oil of Coriandrum sativum. Food Chem. 2009;113(2):526-9. https://doi.org/10.1016/j.foodchem.2008.07.097.
-
18.
Lal AA, Kumar T, Murthy PB, Pillai KS. Hypolipidemic effect of Coriandrum sativum L. in triton-induced hyperlipidemic rats. Indian J Exp Biol. 2004;42(9):909-12. [PubMed ID: 15462185].
-
19.
Naquvi KJ, Ali M, Ahamad J. Antidiabetic activity of aqueous extract of coriandrum sativum L. fruits in streptozotocin induced rats. Int J Pharm Pharm Sci. 2011;4(239-40).
-
20.
Asgarpanah J, Kazemivash N. Phytochemistry, pharmacology and medicinal properties of Coriandrum sativum L. Afr J Pharm Pharmacol. 2012;6:2340-5.
-
21.
Wong P, Kitts D. Studies on the dual antioxidant and antibacterial properties of parsley (Petroselinum crispum) and cilantro (Coriandrum sativum) extracts. Food Chem. 2006;97(3):505-15. https://doi.org/10.1016/j.foodchem.2005.05.031.
-
22.
Tang EL, Rajarajeswaran J, Fung SY, Kanthimathi MS. Antioxidant activity of Coriandrum sativum and protection against DNA damage and cancer cell migration. BMC Complement Altern Med. 2013;13:347. [PubMed ID: 24517259]. https://doi.org/10.1186/1472-6882-13-347.
-
23.
Hashim M, Lincy S, Remya V, Teena M, Anila L. Effect of polyphenolic compounds from on HO-induced oxidative stress in human lymphocytes. Food Chem. 2005;92(4):653-60. https://doi.org/10.1016/j.foodchem.2004.08.027.
-
24.
Momin AH, Acharya SS, Gajjar AV. Coriandrum sativum-review of advances in phytopharmacology. Int J Pharm Sci Res. 2012;3:1233-9.
-
25.
Al-Snafi AE. A review on chemical constituents and pharmacological activities of Coriandrum sativum. IOSR J Pharm. 2016;6(7):17-42. https://doi.org/10.9790/3013-067031742.
-
26.
Kath RK, Gupta RK. Antioxidant activity of hydroalcoholic leaf extract of ocimum sanctum in animal models of peptic ulcer. Indian J Physiol Pharmacol. 2006;50(4):391-6. [PubMed ID: 17402269].
-
27.
Claiborne A. Catalase Activity. In: Greenwald RA, editor. Handbook of methods for oxygen radical research. Boca Raton, USA: CRC Press; 1986.
-
28.
Valko M, Jomova K, Rhodes CJ, Kuca K, Musilek K. Redox- and non-redox-metal-induced formation of free radicals and their role in human disease. Arch Toxicol. 2016;90(1):1-37. [PubMed ID: 26343967]. https://doi.org/10.1007/s00204-015-1579-5.
-
29.
Abd Elkader M, Farouk Aly H. Protective effect of melatonin against iron overload-induced toxicity in rats. Int J Pharm Pharm Sci. 2015;7:116-21.
-
30.
Zaribaf F, Entezari MH, Hassanzadeh A, Mirzaian S. Association between dietary iron, iron stores, and serum lipid profile in reproductive age women. J Educ Health Promot. 2014;3:15. [PubMed ID: 24741655]. https://doi.org/10.4103/2277-9531.127586.
-
31.
Badria FA, Ibrahim AS, Badria AF, Elmarakby AA. Curcumin Attenuates Iron Accumulation and Oxidative Stress in the Liver and Spleen of Chronic Iron-Overloaded Rats. PLoS One. 2015;10(7). e0134156. [PubMed ID: 26230491]. https://doi.org/10.1371/journal.pone.0134156.
-
32.
Sarkar R, Hazra B, Mandal N. Hepatoprotective Potential of Caesalpinia crista against Iron-Overload-Induced Liver Toxicity in Mice. Evid Based Complement Alternat Med. 2012;2012:896341. [PubMed ID: 22919421]. https://doi.org/10.1155/2012/896341.
-
33.
Aziza SA, Azab Mel S, El-Shall SK. Ameliorating role of rutin on oxidative stress induced by iron overload in hepatic tissue of rats. Pak J Biol Sci. 2014;17(8):964-77. [PubMed ID: 26031015]. https://doi.org/10.3923/pjbs.2014.964.977.
-
34.
Galleano M, Simontacchi M, Puntarulo S. Nitric oxide and iron: effect of iron overload on nitric oxide production in endotoxemia. Mol Aspects Med. 2004;25(1-2):141-54. [PubMed ID: 15051323]. https://doi.org/10.1016/j.mam.2004.02.015.
-
35.
Zurlo MG, De Stefano P, Borgna-Pignatti C, Di Palma A, Piga A, Melevendi C, et al. Survival and causes of death in thalassaemia major. Lancet. 1989;2(8653):27-30. [PubMed ID: 2567801]. https://doi.org/10.1016/S0140-6736(89)90264-X.
-
36.
Powell LW, Bassett ML, Halliday JW. Hemochromatosis: 1980 update. Gastroenterology. 1980;78(2):374-81. [PubMed ID: 6985597].
-
37.
Jaccob AA, Hussain SA. Effects of long-term use of flavonoids on the absorption and tissue distribution of orally administered doses of trace elements in rats. Pharmacol Pharm. 2012;3(4):474-80. https://doi.org/10.4236/pp.2012.34065.
-
38.
Kumar S, Pandey AK. Chemistry and biological activities of flavonoids: an overview. ScientificWorldJournal. 2013;2013:162750. [PubMed ID: 24470791]. https://doi.org/10.1155/2013/162750.
-
39.
Ebrahimzadeh MA, Pourmorad F, Bekhradnia AR. Iron chelating activity, phenol and flavonoid content of some medicinal plants from Iran. Afr J Biotechnol. 2008;7(18):3188-92.
-
40.
Najafzadeh H, Jalali MR, Morovvati H, Taravati F. Comparison of the prophylactic effect of silymarin and deferoxamine on iron overload-induced hepatotoxicity in rat. J Med Toxicol. 2010;6(1):22-6. [PubMed ID: 20182837]. https://doi.org/10.1007/s13181-010-0030-9.
-
41.
Ebrahimzadeh MA, Nabavi SM, Nabavi SF. Correlation between the in vitro iron chelating activity and poly phenol and flavonoid contents of some medicinal plants. Pak J Biol Sci. 2009;12(12):934-8. [PubMed ID: 19777789]. https://doi.org/10.3923/pjbs.2009.934.938.
-
42.
Kaltwasser JP, Werner E, Schalk K, Hansen C, Gottschalk R, Seidl C. Clinical trial on the effect of regular tea drinking on iron accumulation in genetic haemochromatosis. Gut. 1998;43(5):699-704. [PubMed ID: 9824354]. https://doi.org/10.1136/gut.43.5.699.
-
43.
Lima GPP, Vianello F, Corrêa CR, Campos RS, Borguini MG. Polyphenols in fruits and vegetables and its effect on human health. Food Nutr Sci. 2014;5(11):1065-82. https://doi.org/10.4236/fns.2014.511117.
-
44.
Aguilera Y, Martin-Cabrejas MA, González de Mejia E. Phenolic compounds in fruits and beverages consumed as part of the mediterranean diet: their role in prevention of chronic diseases. Phytochem Rev. 2015;15(3):405-23. https://doi.org/10.1007/s11101-015-9443-z.