Abstract
Context:
Patients with mild cognitive impairment (MCI) and Alzheimer’s disease (AD) are more likely to suffer from cognitive dysfunction due to dementia. Transcranial direct current stimulation (tDCS) as a non-invasive treatment strategy has been used as an efficient strategy to improve cognitive function.Evidence Acquisition:
Several studies investigating tDCS over the dorsolateral prefrontal cortex (dlPFC) were reviewed.Results:
Most of these studies have targeted the left dlPFC, indicating that tDCS over the dlPFC can improve cognitive function, such as memory, visual recognition, attention, decision-making, and cognitive decline. However, there are still many unanswered questions about the effect of tDCS over the dlPFC on the connectivity of this brain region as a key node for cognitive function. Meanwhile, further research should be conducted to elucidate the effects of tDCS over the dlPFC on brain connectivity and AD biomarkers. In addition, more investigations should be conducted to identify the exact mechanisms affecting dlPFC stimulation on cognitive dysfunction in AD patients.Conclusions:
Based on the results, tDCS over the DlPFC could improve cognitive function in AD patients.Keywords
Transcranial Direct Current Stimulation Dorsolateral Prefrontal Cortex Cognition Alzheimer’s Disease
1. Context
Alzheimer’s disease (AD) is a progressive neurodegenerative disease, which leads to brain dysfunction, poor performance in memory, executive function, visuospatial abilities, language, planning, and abstract reasoning (1). According to estimates, 4% of people under 65 suffer from Alzheimer’s. Cognitive decline is the most critical problem in AD, and the dorsolateral prefrontal cortex (dlPFC) plays an essential role in cognitive function (2). Therefore, this area has been targeted in many studies to assess cognitive dysfunction in AD, which is discussed here. This review provided a literature overview on the effects of tDCS over the dlPFC on cognitive dysfunction induced by AD.
2. Evidence Acquisition
As mentioned, this paper reviews the effects of tDCS over the dlPFC on cognitive function in AD patients based on a non-systematic approach. The articles were searched in the Scopus-, Google Scholar-, and PubMed-based literature review that targeted AD using tDCS over the dlPFC and conducted before Sep 2021. The keywords included “Alzheimer’s disease” AND “Transcranial Direct Stimulation (tDCS),” “Cognitive Dysfunction” AND “tDCS,” “Dorsolateral Prefrontal Cortex (dlPFC),” AND “tDCS.” The data collection process focused primarily on brain stimulation methods, patient characteristics, presence and/or absence of cognitive symptoms, study design and experimental protocols, and quantification of stimulation parameters. Studies conducted using healthy subjects and animal models were excluded from this study.
2.1. Pathophysiology of AD
Previous studies have suggested several mechanisms underlying AD pathophysiology and AD-induced cognitive decline, including cholinergic deficits, formation/accumulation of neurotoxic substances, oxidative stress, neuroinflammation, and mitochondrial dysfunction (3). Amyloid beta (Aβ) accumulation, particularly soluble neurotoxic oligomers, affects tau hyperphosphorylation, oxidative stress, neuroinflammation, and mitochondrial dysfunction, progressing disease through their downstream molecular cascades. Neurofibrillary tangles (NFTs) form intracellular aggregations that slowly propagate in the hippocampal region, known as the golden hallmark of AD (4, 5). Even though these findings may explain the probable foundations of cognitive impairment in AD, the precise mechanisms behind the loss of synapses and neurons in AD remain to be elucidated.
2.2. Pharmacological and Non-pharmacological Treatments
Available pharmacological treatments for AD are currently ineffective, causing side effects such as nausea, diarrhea, vomiting, dizziness, and agitation (Table 1) (6). Considering such limitations of pharmacological treatments, most researchers may prefer recently developed non-pharmacological interventions such as brain stimulation.
Common and Rare Side Effects of Pharmacological Treatment of Alzheimer’s Disease
Drugs | Side Effects |
---|---|
Donepezil | Insomnia, diarrhea, dizziness, fatigue, dizziness, rhinitis, anxiety, sleep disturbances, rare seizures |
Galantamine | Convulsions, anorexia, dizziness, tremor, confusion, depression, rare seizures, and rare syncope |
Rivastigmine | Dizziness, fatigue, headache, rare severe vomiting with esophageal rupture, rare syncope |
Memantine | Dizziness, headache, constipation, rare seizures |
Tacrine | Dizziness, diarrhea, dyspepsia, myalgia, anorexia, seizures, dizziness, insomnia, hepatotoxicity |
2.3. Brain Stimulation
Brain stimulation modulates neuronal activity in specific brain regions or networks using an electronic or electromagnetic field. Invasive and noninvasive approaches are generally divided into two categories. There are two types of invasive brain stimulation: VNS and DBS. VNS can stimulate the vagus nerve using a programmable neurostimulator implanted subcutaneously in the chest or near the ear (7, 8). DBS involves implanted electrodes in the brain powered by a pulse generator placed subcutaneously in the chest (9). TMS and tDCS are two common forms of noninvasive brain stimulation. tDCS is a noninvasive approach that delivers weak electrical currents into the brain through saline-soaked sponge electrodes (10). TMS involves delivering magnetic currents via a coil of wire that generates an electric current in the targeted brain regions based on Faraday’s law (11).
These methods have some side effects which limit their application (see advantages and disadvantages in Figure 1). Among these methods, tDCS has the fewest side effects because it only induces mild effects like redness and itching of the skin (12). In addition, tDCS has several advantages over rTMS, such as being less invasive and expensive, easier to manage, more controllable, and portable, leading individuals to consider it as a suitable treatment at home in the future (13). Few studies have investigated the effects of tDCS on cognitive function in AD patients, given the efficiency of tDCS on various cognitive processes in healthy individuals as well as psychiatric and neurological patients, as mentioned above.
Classification of brain stimulation approaches. Blue represents the advantages, and pink presents the disadvantages of different types of brain stimulation.
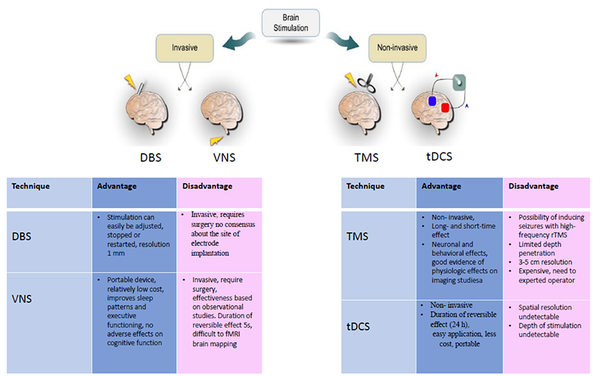
2.4. The dlPFC and Cognitive Function
The dlPFC is located in the middle frontal gyrus, typically defined as Brodmann areas 46 (BA 46) and 9 (BA 9) (14). This area is extensively connected to various cortical and subcortical regions involved in cognitive function, particularly in executive control and memory processing (15). More precisely, the dlPFC is involved in either working memory (16, 17), attentional bias (18), decision-making (19), language comprehension (20) and production (21), and cognitive control (22). Furthermore, some critical role is known for dlPFC, such as attention (23), problem-solving (24), working memory (25), and risky decision-making (26).
2.5. Effects of tDCS on Memory in AD Patients
Several studies have focused on the dlPFC due to its extensive connections to various cortical and subcortical regions involved in numerous cognitive functions (15). Numerous studies have evaluated the effects of tDCS over the left dlPFC on memory in AD patients. A single tDCS session has been shown to improve recognition memory (27), whereas multiple tDCS sessions in AD patients have improved global cognition (28). In contrast, Suemoto et al. demonstrated that repeated anodal tDCS applied over the dlPFC did not affect global cognitive performance (29).
The effect of tDCS over the dlPFC and recognition memory in AD improved visual recognition memory, but the Stroop digit span test showed no significant differences between the active and sham groups (27). Cotelli et al. (30) showed that anodal tDCS (AtDCS) plus computer-assisted training (CT) improved memory for naming faces in AD. This study showed that both (AtDCS + CT) and (PtDCS + CT) improved performance compared to the AtDCS + motor training group after two weeks of memory training (30). All patients received tDCS over the left dlPFC (five sessions of 25 min/day, at a constant current of 2 mA) for two weeks (30). A case study has examined the effect of 10 continuous daily sessions of tDCS over the left dlPFC and showed the stability of global cognition in mild AD subjects that lasted for three months (31). However, long-term treatment with tDCS over the dlPFC needs to be investigated. For example, a case study reported that daily tDCS stimulation over the temporal lobe for eight months improved memory and slowed cognitive decline in a patient with early-onset AD (32). Moreover, a recent study reported that applying tDCS at home could effectively improve cognitive decline accompanied by AD (33).
In the early stages of AD, patients suffer from dlPFC dysfunction (34), leading to WM impairment and especially its executive component (35). In addition, plasticity impairment in the dlPFC of AD patients should be considered an important index to improve WM deficits (36). Moreover, two trials have investigated noninvasive stimulation’s effect on improving WM in AD patients using tDCS in only one case (27, 31). Boggio et al. (27) applied tDCS over the left temporal and dlPFC to assess WM by a forward and backward digit span test and found no significant differences between sham and active stimulation (27), which may be due to the low sensitivity of the digit span test to the effects of tDCS (27). The electroencephalography (EEG) and event-related potentials used in another study have been found to improve the low sensitivity of previous tests using intelligent methods (37).
As discussed above, the effects of tDCS on memory have been explored in various studies, but the exact involved mechanisms are not yet clear. Alterations in multiple neurotransmitter systems may be one of the important mechanisms for the beneficial effects of tDCS (38). In addition, tDCS-induced modulation of neuronal excitability may, in part, account for some other beneficial effects of this technique in AD patients. For example, the temporal-parietal area in AD patients is hypoactive, and tDCS increases cortical activity (39). Cathodal tDCS may decrease hyperexcitability in the frontal region of AD patients (40). Despite these previous studies, the optimal stimulation protocol remains unknown, including current intensity, duration, session, and long-term period. Therefore, for future studies, the exact mechanisms involved in the effects of tDCS on memory, e.g., its probable effects on Ca2+ levels, brain-derived neurotrophic factor (BDNF), acetylcholine, dopamine, and other neurotransmitters, play important roles in neural plasticity.
2.6. The Effects of tDCS on Attention
Evidence shows that demanding executive processes of selective and divided attention may be impaired in the early stages of AD (35). AD is associated with deficits in span, focus, selective attention, and divided attention (41). As an important region in the attentional network, the PFC has been targeted in various tDCS studies. Boggio et al. (27) revealed that tDCS application over the left dlPFC in AD patients did not affect their attention in the Stroop task. In a literature survey, Siever (42) concluded that a large anodal electrode in FP1 or FP2 and a contralateral shoulder cathode might improve attention. Another protocol involves a large anodal electrode in FP1 or FP2 with a cathode in the neck. In stroke patients, experiments to strengthen attention have been performed using a similar protocol with an anodal electrode over the left dlPFC and a cathodal electrode in the contralateral supraorbital region (43). The effectiveness of tDCS over the dlPFC in AD patients has only been studied in a few studies, but data from other dementias suggest that stimulation can enhance attention. The main drawback of this approach includes using a bipolar montage (two electrodes were placed on the scalp) and the current distributions related to the size of the anodal and cathodal electrodes and the position of the reference electrode (10). However, the long-lasting effects of tDCS are poorly understood and need further investigation.
2.7. The tDCS and Decision Making
In AD patients, risky decision-making is associated with impairments in WM, mental flexibility, numerical ability, and inhibition, whereas impairments in learning ability, memory, and emotional processing occur in ambiguity (44). A literature survey revealed that a few studies have so far examined the effect of tDCS on the dlPFC on decision-making in AD patients (45, 46). Based on this study, the ability to decide in risky situations may be impaired, but ambiguity may not be damaged in AD patients (45). Thams et al. (46) evaluated the effects of the dlPFC on decision-making, combining three weeks of cognitive training (before and after brain stimulation) via tDCS (1 mA for 20 min). In this study, the immediate and long-term effects of tDCS (1 and 7 months after training) improved decision-making in AD patients (46). Overall, these studies have highlighted the need to assess other effects of tDCS over the dlPFC on decision-making in AD patients and determine the improvement of this noninvasive brain stimulation regarding the cognitive function component in AD patients. According to physiological theory, neurophysiological changes could be responsible for the altered decision-making in AD (47). Evidence reveals that alterations in various brain regions, including the frontal, parietal, and temporal cortices, and also changes in the neurotransmitters dopamine, serotonin, norepinephrine (48), and glutamate (49), could lead to altered decision-making in AD patients (47). More investigations are needed to examine the effects of tDCS on neurotransmitters and decision-making in AD patients.
3. Discussion
Alzheimer’s disease is a complex neurodegenerative disorder, representing one of modern society’s major problems. Research efforts to understand the underlying mechanisms of AD and develop treatment strategies have failed. Therefore, the efforts to elucidate the mechanism of the disease and find new methods continue with vigor.
This paper reviewed tDCS as a painless method to affect AD. This technique applies a weak electric current across the scalp whose distribution into cortical networks depends on various factors, including current density, stimulation duration, size and mounting of electrodes, and electric field orientation relative to the anatomical and geometric features. There are several possible explanations for these important issues. For instance, most studies above used a two-mA current intensity during a 10-session stimulation episode, but its duration varied from 20 to 30 min. Second, different electrode surfaces could be used in various cognitive functional networks, and a high-density tDCS (HD-tDCS) protocol may decrease this disturbance. Finally, duration and times of stimulations may be other determinant factors. Some previous studies have used daily stimulations, while others have applied 10 or 6 sessions during two weeks. Previous reports examined different protocols, and further efforts are required to ensure optimal protocols for tDCS application.
Most studies on the cognitive functions of tDCS in AD patients have primarily addressed multiple stimulations on the dlPFC, while a few have used single stimulations (50). tDCS may, in part, affect AD via inducing neural plasticity, especially LTP, in various brain regions. Most previous studies have followed up on the patients for six months. Since AD is a progressive disorder, further studies with longer durations of follow-up are recommended to assess the long-lasting effects of tDCS. The sufficient sample size in different cognitive domains, appropriate stimulation protocols (e.g., intensity, duration, and the number of repeated stimulations), cognitive tests with high sensitivity, and long-term follow-up would seem to be important issues in need of further investigations to evaluate the tDCS as a potential complementary treatment for AD.
AD is an irreversible, progressive neurodegenerative disease that begins decades before the onset of clinical symptoms (51). Previous studies have shown that changes in synaptic function, loss of synapses, and dystrophic axons are characteristic features in neurodegenerative diseases. Further studies have shown that Aβ and tau levels gradually increase. In contrast, symptomatic cognitive impairment occurs with a delay of 10 - 20 years between the onset of accumulation and a decrease in Aβ accumulation synchronous with the start of symptomatic disease (52). Knopman et al. (53) reported in a literature review that the Aβ and tau neurotoxicity leads to synaptic changes and synapse loss. In addition, studies have revealed a strong relationship between synaptic loss and cognitive decline (54). Moreover, evidence indicates that neuroinflammation occurs years before the onset of cognitive decline in AD (55). The anti-inflammatory effect of NA in the brain is well established (56), and the structure and functions of the NA system have also been shown to be disrupted at the early stages of AD. In animal models of AD, toxin-induced LC neurodegeneration could lead to increased accumulation of Aβ-plaques and neuroinflammation in the brain and alters the expression of NE receptors (57) as an early causal factor for AD.
Moreover, monoaminergic systems are involved in various physiological functions, including regulating movement, cognitive processes, mood, affective states, attention, and sleep. Monoaminergic systems also affect the degeneration of neurons within subcortical monoaminergic systems as an important early hallmark of AD before the deposition of extracellular β-amyloid plaques and intracellular NFT in the cortex (58). Evidence may also demonstrate that the initial degeneration of deep-seated monoaminergic nuclei may trigger the transneuronal spread of AD pathology toward the hippocampus and cortex, affecting learning and memory function (8, 59). The findings suggest exploring novel markers for therapeutic methods such as tDCS may be more effective before neuronal and synaptic dysfunction occurs.
4. Conclusions
Based on the results, tDCS can be an effective complementary therapy for AD-induced cognitive decline. The exact mechanisms involved in the beneficial effects of tDCS are not yet clear, but the probable mechanisms may include various neurophysiological signs and the underlying basis of AD, including reducing Aβ plaques and tau proteins, improving LTP and neural plasticity, and alterations of the abnormal neurotransmitter systems. Further studies should also consider important parameters, such as intensity, duration, and number of stimulations, in addition to the exact mechanisms. Furthermore, an important gap in previous findings is the lack of identifying biomarkers before neuronal and synaptic loss. Further studies are needed to develop a complete picture of the treatment strategy for AD and determine the above factors.
References
-
1.
Monsell S. Task switching. Trends Cogn Sci. 2003;7(3):134-40. [PubMed ID: 12639695]. https://doi.org/10.1016/s1364-6613(03)00028-7.
-
2.
Miller B, Cummings JL. The human frontal lobes: Functions and disorders. New York City, USA: Guilford Publications; 2017.
-
3.
Guo T, Zhang D, Zeng Y, Huang TY, Xu H, Zhao Y. Molecular and cellular mechanisms underlying the pathogenesis of Alzheimer's disease. Mol Neurodegener. 2020;15(1):40. [PubMed ID: 32677986]. [PubMed Central ID: PMC7364557]. https://doi.org/10.1186/s13024-020-00391-7.
-
4.
Vickers JC, Dickson TC, Adlard PA, Saunders HL, King CE, McCormack G. The cause of neuronal degeneration in Alzheimer's disease. Prog Neurobiol. 2000;60(2):139-65. [PubMed ID: 10639052]. https://doi.org/10.1016/s0301-0082(99)00023-4.
-
5.
Mikaeili Galeh T, Ghazvini H, Sarvi S, Mohammadi M, Asgarian-Omran H, Hajizadeh F, et al. Controversial Effects of Diverse Types of Toxoplasma gondii on the Anxiety-like Behavior and Cognitive Impairments in the Animal Model of Alzheimer's Disease. Iran J Psychiatry Behav Sci. 2022;16(3). https://doi.org/10.5812/ijpbs-122961.
-
6.
Shafqat S. Alzheimer disease therapeutics: perspectives from the developing world. J Alzheimers Dis. 2008;15(2):285-7. [PubMed ID: 18953114]. https://doi.org/10.3233/jad-2008-15211.
-
7.
Aalbers MW, Rijkers K, Klinkenberg S, Majoie M, Cornips EM. Vagus nerve stimulation lead removal or replacement: surgical technique, institutional experience, and literature overview. Acta Neurochir (Wien). 2015;157(11):1917-24. [PubMed ID: 26335753]. [PubMed Central ID: PMC4604497]. https://doi.org/10.1007/s00701-015-2547-9.
-
8.
Ghazvini H, Zarghami M, Rafaiee R, Beirami E, Seyedhosseini Tamijani SM. Noninvasive Vagus Nerve Stimulation in the Treatment of Methamphetamine Use Disorder: A Review Article. Iran J Psychiatry Behav Sci. 2022;16(4). https://doi.org/10.5812/ijpbs-123423.
-
9.
Lozano AM, Lipsman N. Probing and regulating dysfunctional circuits using deep brain stimulation. Neuron. 2013;77(3):406-24. [PubMed ID: 23395370]. https://doi.org/10.1016/j.neuron.2013.01.020.
-
10.
Woods AJ, Antal A, Bikson M, Boggio PS, Brunoni AR, Celnik P, et al. A technical guide to tDCS, and related non-invasive brain stimulation tools. Clin Neurophysiol. 2016;127(2):1031-48. [PubMed ID: 26652115]. [PubMed Central ID: PMC4747791]. https://doi.org/10.1016/j.clinph.2015.11.012.
-
11.
Hallett M. Transcranial magnetic stimulation: a primer. Neuron. 2007;55(2):187-99. [PubMed ID: 17640522]. https://doi.org/10.1016/j.neuron.2007.06.026.
-
12.
Russo C, Souza Carneiro MI, Bolognini N, Fregni F. Safety Review of Transcranial Direct Current Stimulation in Stroke. Neuromodulation. 2017;20(3):215-22. [PubMed ID: 28220641]. [PubMed Central ID: PMC5389927]. https://doi.org/10.1111/ner.12574.
-
13.
Nitsche MA, Cohen LG, Wassermann EM, Priori A, Lang N, Antal A, et al. Transcranial direct current stimulation: State of the art 2008. Brain Stimul. 2008;1(3):206-23. [PubMed ID: 20633386]. https://doi.org/10.1016/j.brs.2008.06.004.
-
14.
Hunsaker MR, Tran GT, Kesner RP. A behavioral analysis of the role of CA3 and CA1 subcortical efferents during classical fear conditioning. Behav Neurosci. 2009;123(3):624-30. [PubMed ID: 19485569]. https://doi.org/10.1037/a0015455.
-
15.
Frith C, Dolan R. The role of the prefrontal cortex in higher cognitive functions. Brain Res Cogn Brain Res. 1996;5(1-2):175-81. [PubMed ID: 9049084]. https://doi.org/10.1016/s0926-6410(96)00054-7.
-
16.
McEchron MD, Bouwmeester H, Tseng W, Weiss C, Disterhoft JF. Hippocampectomy disrupts auditory trace fear conditioning and contextual fear conditioning in the rat. Hippocampus. 1998;8(6):638-46. [PubMed ID: 9882021]. https://doi.org/10.1002/(SICI)1098-1063(1998)8:6<638::AID-HIPO6>3.0.CO;2-Q.
-
17.
Soto D, Humphreys GW, Heinke D. Working memory can guide pop-out search. Vision Res. 2006;46(6-7):1010-8. [PubMed ID: 16257030]. https://doi.org/10.1016/j.visres.2005.09.008.
-
18.
Knight HC, Smith DT, Ellison A. The role of the left dorsolateral prefrontal cortex in attentional bias. Neuropsychologia. 2020;148:107631. [PubMed ID: 32976854]. https://doi.org/10.1016/j.neuropsychologia.2020.107631.
-
19.
Nitsche MA, Paulus W. Transcranial direct current stimulation--update 2011. Restor Neurol Neurosci. 2011;29(6):463-92. [PubMed ID: 22085959]. https://doi.org/10.3233/RNN-2011-0618.
-
20.
Hsu NS, Jaeggi SM, Novick JM. A common neural hub resolves syntactic and non-syntactic conflict through cooperation with task-specific networks. Brain Lang. 2017;166:63-77. [PubMed ID: 28110105]. [PubMed Central ID: PMC5293615]. https://doi.org/10.1016/j.bandl.2016.12.006.
-
21.
Humphreys GF, Gennari SP. Competitive mechanisms in sentence processing: common and distinct production and reading comprehension networks linked to the prefrontal cortex. Neuroimage. 2014;84:354-66. [PubMed ID: 24012545]. https://doi.org/10.1016/j.neuroimage.2013.08.059.
-
22.
Finn ES, Huber L, Jangraw DC, Molfese PJ, Bandettini PA. Layer-dependent activity in human prefrontal cortex during working memory. Nat Neurosci. 2019;22(10):1687-95. [PubMed ID: 31551596]. [PubMed Central ID: PMC6764601]. https://doi.org/10.1038/s41593-019-0487-z.
-
23.
Johnson JA, Zatorre RJ. Neural substrates for dividing and focusing attention between simultaneous auditory and visual events. Neuroimage. 2006;31(4):1673-81. [PubMed ID: 16616520]. https://doi.org/10.1016/j.neuroimage.2006.02.026.
-
24.
Juliyanto E, Marwoto P, Iswari RS, Nugroho SE, Mindyarto BN; Wiyanto. Brain activity of problem solving proccess: a systematic literarure review. J Phys: Conf Ser. 2021;1918(5). https://doi.org/10.1088/1742-6596/1918/5/052068.
-
25.
Owen AM. The functional organization of working memory processes within human lateral frontal cortex: the contribution of functional neuroimaging. Eur J Neurosci. 1997;9(7):1329-39. [PubMed ID: 9240390]. https://doi.org/10.1111/j.1460-9568.1997.tb01487.x.
-
26.
Minati L, Campanha C, Critchley HD, Boggio PS. Effects of transcranial direct-current stimulation (tDCS) of the dorsolateral prefrontal cortex (DLPFC) during a mixed-gambling risky decision-making task. Cogn Neurosci. 2012;3(2):80-8. [PubMed ID: 24168688]. https://doi.org/10.1080/17588928.2011.628382.
-
27.
Boggio PS, Khoury LP, Martins DC, Martins OE, de Macedo EC, Fregni F. Temporal cortex direct current stimulation enhances performance on a visual recognition memory task in Alzheimer disease. J Neurol Neurosurg Psychiatry. 2009;80(4):444-7. [PubMed ID: 18977813]. https://doi.org/10.1136/jnnp.2007.141853.
-
28.
Khedr EM, Gamal NF, El-Fetoh NA, Khalifa H, Ahmed EM, Ali AM, et al. A double-blind randomized clinical trial on the efficacy of cortical direct current stimulation for the treatment of Alzheimer's disease. Front Aging Neurosci. 2014;6:275. [PubMed ID: 25346688]. [PubMed Central ID: PMC4191219]. https://doi.org/10.3389/fnagi.2014.00275.
-
29.
Suemoto CK, Apolinario D, Nakamura-Palacios EM, Lopes L, Leite RE, Sales MC, et al. Effects of a non-focal plasticity protocol on apathy in moderate Alzheimer's disease: a randomized, double-blind, sham-controlled trial. Brain Stimul. 2014;7(2):308-13. [PubMed ID: 24262299]. https://doi.org/10.1016/j.brs.2013.10.003.
-
30.
Cotelli M, Manenti R, Brambilla M, Petesi M, Rosini S, Ferrari C, et al. Anodal tDCS during face-name associations memory training in Alzheimer's patients. Front Aging Neurosci. 2014;6:38. [PubMed ID: 24678298]. [PubMed Central ID: PMC3958642]. https://doi.org/10.3389/fnagi.2014.00038.
-
31.
Penolazzi B, Bergamaschi S, Pastore M, Villani D, Sartori G, Mondini S. Transcranial direct current stimulation and cognitive training in the rehabilitation of Alzheimer disease: A case study. Neuropsychol Rehabil. 2015;25(6):799-817. [PubMed ID: 25379604]. https://doi.org/10.1080/09602011.2014.977301.
-
32.
Bystad M, Rasmussen ID, Gronli O, Aslaksen PM. Can 8 months of daily tDCS application slow the cognitive decline in Alzheimer's disease? A case study. Neurocase. 2017;23(2):146-8. [PubMed ID: 28485663]. https://doi.org/10.1080/13554794.2017.1325911.
-
33.
Knotkova H, Clayton A, Stevens M, Riggs A, Charvet LE, Bikson M. Home-Based Patient-Delivered Remotely Supervised Transcranial Direct Current Stimulation. In: Knotkova H, Nitsche MA, Bikson M, Woods AJ, editors. Practical Guide to Transcranial Direct Current Stimulation. New York City, USA: Springer; 2019. p. 379-405. https://doi.org/10.1007/978-3-319-95948-1_13.
-
34.
Kaufman LD, Pratt J, Levine B, Black SE. Executive deficits detected in mild Alzheimer's disease using the antisaccade task. Brain Behav. 2012;2(1):15-21. [PubMed ID: 22574270]. [PubMed Central ID: PMC3343295]. https://doi.org/10.1002/brb3.28.
-
35.
Huntley JD, Howard RJ. Working memory in early Alzheimer's disease: a neuropsychological review. Int J Geriatr Psychiatry. 2010;25(2):121-32. [PubMed ID: 19672843]. https://doi.org/10.1002/gps.2314.
-
36.
Kumar S, Zomorrodi R, Ghazala Z, Goodman MS, Blumberger DM, Cheam A, et al. Extent of Dorsolateral Prefrontal Cortex Plasticity and Its Association With Working Memory in Patients With Alzheimer Disease. JAMA Psychiatry. 2017;74(12):1266-74. [PubMed ID: 29071355]. [PubMed Central ID: PMC6583382]. https://doi.org/10.1001/jamapsychiatry.2017.3292.
-
37.
Cespon J, Rodella C, Miniussi C, Pellicciari MC. Behavioural and electrophysiological modulations induced by transcranial direct current stimulation in healthy elderly and Alzheimer's disease patients: A pilot study. Clin Neurophysiol. 2019;130(11):2038-52. [PubMed ID: 31541981]. https://doi.org/10.1016/j.clinph.2019.08.016.
-
38.
Dayan E, Censor N, Buch ER, Sandrini M, Cohen LG. Noninvasive brain stimulation: from physiology to network dynamics and back. Nat Neurosci. 2013;16(7):838-44. [PubMed ID: 23799477]. [PubMed Central ID: PMC4876726]. https://doi.org/10.1038/nn.3422.
-
39.
Fernandez A, Maestu F, Amo C, Gil P, Fehr T, Wienbruch C, et al. Focal temporoparietal slow activity in Alzheimer's disease revealed by magnetoencephalography. Biol Psychiatry. 2002;52(7):764-70. [PubMed ID: 12372668]. https://doi.org/10.1016/s0006-3223(02)01366-5.
-
40.
Hansen N. Action mechanisms of transcranial direct current stimulation in Alzheimer's disease and memory loss. Front Psychiatry. 2012;3:48. [PubMed ID: 22615703]. [PubMed Central ID: PMC3351674]. https://doi.org/10.3389/fpsyt.2012.00048.
-
41.
Festa EK, Heindel WC, Ott BR. Dual-task conditions modulate the efficiency of selective attention mechanisms in Alzheimer's disease. Neuropsychologia. 2010;48(11):3252-61. [PubMed ID: 20621109]. [PubMed Central ID: PMC2928570]. https://doi.org/10.1016/j.neuropsychologia.2010.07.003.
-
42.
Siever D. Neuro Connections. 2013. Transcranial dc stimulation. Available from: https://www.elixa.com/wp-content/uploads/2018/02/tDCS-Neuroconnections-article.pdf.
-
43.
Kang EK, Baek MJ, Kim S, Paik NJ. Non-invasive cortical stimulation improves post-stroke attention decline. Restor Neurol Neurosci. 2009;27(6):645-50. [PubMed ID: 20042788]. https://doi.org/10.3233/RNN-2009-0514.
-
44.
Gaubert F, Chainay H. Decision-Making Competence in Patients with Alzheimer's Disease: A Review of the Literature. Neuropsychol Rev. 2021;31(2):267-87. [PubMed ID: 33576942]. https://doi.org/10.1007/s11065-020-09472-2.
-
45.
Sun T, Xie T, Wang J, Zhang L, Tian Y, Wang K, et al. Decision-Making Under Ambiguity or Risk in Individuals With Alzheimer's Disease and Mild Cognitive Impairment. Front Psychiatry. 2020;11:218. [PubMed ID: 32256419]. [PubMed Central ID: PMC7093589]. https://doi.org/10.3389/fpsyt.2020.00218.
-
46.
Thams F, Kuzmina A, Backhaus M, Li SC, Grittner U, Antonenko D, et al. Cognitive training and brain stimulation in prodromal Alzheimer's disease (AD-Stim)-study protocol for a double-blind randomized controlled phase IIb (monocenter) trial. Alzheimers Res Ther. 2020;12(1):142. [PubMed ID: 33160420]. [PubMed Central ID: PMC7648990]. https://doi.org/10.1186/s13195-020-00692-5.
-
47.
Sun W, Matsuoka T, Narumoto J. Decision-Making Support for People With Alzheimer's Disease: A Narrative Review. Front Psychol. 2021;12:750803. [PubMed ID: 34867639]. [PubMed Central ID: PMC8633444]. https://doi.org/10.3389/fpsyg.2021.750803.
-
48.
Mohr PN, Li SC, Heekeren HR. Neuroeconomics and aging: neuromodulation of economic decision making in old age. Neurosci Biobehav Rev. 2010;34(5):678-88. [PubMed ID: 19501615]. https://doi.org/10.1016/j.neubiorev.2009.05.010.
-
49.
Samanez-Larkin GR, Knutson B. Decision making in the ageing brain: changes in affective and motivational circuits. Nat Rev Neurosci. 2015;16(5):278-89. [PubMed ID: 25873038]. [PubMed Central ID: PMC5645075]. https://doi.org/10.1038/nrn3917.
-
50.
Nardone R, Bergmann J, Christova M, Caleri F, Tezzon F, Ladurner G, et al. Effect of transcranial brain stimulation for the treatment of Alzheimer disease: a review. Int J Alzheimers Dis. 2012;2012:687909. [PubMed ID: 22114748]. [PubMed Central ID: PMC3202129]. https://doi.org/10.1155/2012/687909.
-
51.
Dubois B, Hampel H, Feldman HH, Scheltens P, Aisen P, Andrieu S, et al. Preclinical Alzheimer's disease: Definition, natural history, and diagnostic criteria. Alzheimers Dement. 2016;12(3):292-323. [PubMed ID: 27012484]. [PubMed Central ID: PMC6417794]. https://doi.org/10.1016/j.jalz.2016.02.002.
-
52.
Jagust WJ, Landau SM, Alzheimer's Disease Neuroimaging I. Temporal Dynamics of beta-Amyloid Accumulation in Aging and Alzheimer Disease. Neurology. 2021;96(9):e1347-57. [PubMed ID: 33408147]. [PubMed Central ID: PMC8055327]. https://doi.org/10.1212/WNL.0000000000011524.
-
53.
Knopman DS, Amieva H, Petersen RC, Chetelat G, Holtzman DM, Hyman BT, et al. Alzheimer disease. Nat Rev Dis Primers. 2021;7(1):33. [PubMed ID: 33986301]. [PubMed Central ID: PMC8574196]. https://doi.org/10.1038/s41572-021-00269-y.
-
54.
DeKosky ST, Scheff SW. Synapse loss in frontal cortex biopsies in Alzheimer's disease: correlation with cognitive severity. Ann Neurol. 1990;27(5):457-64. [PubMed ID: 2360787]. https://doi.org/10.1002/ana.410270502.
-
55.
Kuo HK, Yen CJ, Chang CH, Kuo CK, Chen JH, Sorond F. Relation of C-reactive protein to stroke, cognitive disorders, and depression in the general population: systematic review and meta-analysis. Lancet Neurol. 2005;4(6):371-80. [PubMed ID: 15907742]. https://doi.org/10.1016/S1474-4422(05)70099-5.
-
56.
Giorgi FS, Saccaro LF, Galgani A, Busceti CL, Biagioni F, Frati A, et al. The role of Locus Coeruleus in neuroinflammation occurring in Alzheimer's disease. Brain Res Bull. 2019;153:47-58. [PubMed ID: 31419539]. https://doi.org/10.1016/j.brainresbull.2019.08.007.
-
57.
Jardanhazi-Kurutz D, Kummer MP, Terwel D, Vogel K, Thiele A, Heneka MT. Distinct adrenergic system changes and neuroinflammation in response to induced locus ceruleus degeneration in APP/PS1 transgenic mice. Neuroscience. 2011;176:396-407. [PubMed ID: 21129451]. https://doi.org/10.1016/j.neuroscience.2010.11.052.
-
58.
Satoh A, Iijima KM. Roles of tau pathology in the locus coeruleus (LC) in age-associated pathophysiology and Alzheimer's disease pathogenesis: Potential strategies to protect the LC against aging. Brain Res. 2019;1702:17-28. [PubMed ID: 29274876]. https://doi.org/10.1016/j.brainres.2017.12.027.
-
59.
Gallo A, Pillet LE, Verpillot R. New frontiers in Alzheimer's disease diagnostic: Monoamines and their derivatives in biological fluids. Exp Gerontol. 2021;152:111452. [PubMed ID: 34182050]. https://doi.org/10.1016/j.exger.2021.111452.