Abstract
Background:
Lactic acid bacteria (LAB) are among the most common probiotics and play a significant role in promoting health within the human intestinal microbiota. Their protective functions in the intestine include producing antimicrobial compounds, regulating the balance of the intestinal microbiome, and more.Objectives:
This study aims to investigate the antimicrobial effects of bacteriocin extracted from Lactobacillus plantarum (L. plantarum) on Escherichia coli (E. coli) strains that cause treatment-resistant urinary tract infections (UTIs) in humans and mastitis in livestock.Methods:
Twenty E. coli isolates were obtained from patients with UTIs and cows with mastitis. After culturing the samples on Eosin-methylene blue, McConkey agar, and blood agar, the strains were identified using biochemical tests. Bacteriocin produced by L. plantarum ATCC8014 was extracted, and its concentration was measured using the Bradford method. The antimicrobial effect of the bacteriocin was assessed using the broth microdilution method to determine the minimum inhibitory concentration (MIC) and minimum bactericidal concentration (MBC). Additionally, the bacteriocin's antimicrobial activity was evaluated through three repetitions of disk diffusion and agar well diffusion methods.Results:
The findings revealed that the MIC and MBC concentrations for all human and animal strains were 13.38 μg/mL and 26.76 μg/mL, respectively. Notably, two animal samples (No. 3 and No. 10) and one human sample (No. 6) showed lower MIC and MBC concentrations compared to the other samples and the standard E. coli sample. However, all strains were resistant to bacteriocin in the disk diffusion and agar well diffusion methods.Conclusions:
The data indicate a high risk of increasing treatment-resistant E. coli strains in UTIs and livestock, which could pose a significant threat to public and animal health. Therefore, it is essential to further investigate the effects of different bacteriocins on these resistant strains, in conjunction with antibiotic therapies.Keywords
1. Background
Lactic acid bacteria (LAB), or lactobacilli, are gram-positive, catalase-negative anaerobes, typically rod-shaped or non-sporulating cocci (1). These bacteria produce lactic acid, the primary byproduct of carbohydrate fermentation, along with several growth inhibitors such as bacteriocins, bacteriocin-like inhibitory substances (BLISs), hydrogen peroxide, diacetyl, and carbon dioxide. This group includes genera such as Streptococcus, Leuconostoc, Pediococcus, and Lactobacillus. Fermenting types generate lactic acid from environmental sugars (2), while non-fermenting types produce acetic acid, ethanol, carbon dioxide, and smaller amounts of other compounds in addition to lactic acid.
Lactobacillusplantarum (L. plantarum), one of the most well-known species of Lactobacillus, is found in various ecological niches, such as vegetables, fermented foods, and healthy human intestinal mucosa (3). Lactobacillus plantarum is widely used in the food and pharmaceutical industries as a starter culture or probiotic due to its significant health benefits. It has been shown to promote health in several ways, including managing fecal flora composition and preventing and treating irritable bowel syndrome (IBS), inflammatory bowel disease (IBD), cancer, coronary heart disease, and certain gastrointestinal symptoms. These attributes reinforce L. plantarum's potential to improve human health (4).
One of the key features of L. plantarum is its ability to produce bacteriocin, an antimicrobial compound that inhibits the growth of pathogenic microorganisms (1). Bacteriocins from L. plantarum disrupt bacterial membranes, causing leakage of cellular contents and interfering with protein synthesis and nucleic acid replication, making them effective against multidrug-resistant pathogens that resist conventional antibiotics (5). Additionally, their high specific activity against such pathogens makes bacteriocins advantageous for therapeutic applications. Thus, the use of bacteriocins, bacteriocin-producing probiotics, or both, presents a novel approach for treating several diseases, including intestinal infections (6).
The Enterobacteriaceae family consists of gram-negative bacilli and facultatively anaerobic organisms, typically measuring 0.5 - 2 micrometers in diameter and 2 - 4 micrometers in length. The family is divided into opportunistic and pathogenic intestinal bacteria (7). Intestinal pathogens include Salmonella, Shigella, and Yersinia, while opportunistic pathogens encompass other genera. Among these, Escherichiacoli (E. coli), a common commensal intestinal bacterium, can cause both gastrointestinal and extra-gastrointestinal infections. As a member of the Enterobacteriaceae family, E. coli is a gram-negative, non-sporing, rod-shaped, facultatively anaerobic, coliform bacterium (8, 9).
Escherichia coli is found in various environments, including the digestive systems of humans and warm-blooded animals, where it is a part of the gut microbiota. It is a leading cause of both gastrointestinal and extraintestinal diseases in animals and humans (10). Urinary tract infections (UTIs) are the most common bacterial infections in humans, caused by various pathogens, including gram-negative bacteria like E. coli (11-13). Uropathogenic E. coli is responsible for about 75% of uncomplicated UTIs (13).
Moreover, E. coli is a major cause of bovine mastitis, leading to subclinical and clinical mastitis, which are characterized by systemic changes, abnormal milk appearance, and udder inflammation (14, 15). The susceptibility of cows to mastitis caused by E. coli varies based on factors such as the cow's age, stage of lactation, and delivery. The pathogenicity of E. coli in a cow's udder results from the interaction between its virulence factors and the host's defenses (14, 16).
2. Objectives
This study aimed to investigate the antimicrobial effects of microbial polypeptides (bacteriocins) extracted from L. plantarum on E. coli strains that cause treatment-resistant UTIs in humans and mastitis in livestock.
3. Methods
3.1. Collection and Separation of Samples
In this study, 20 E. coli samples were collected from both human and animal sources (10 from humans and 10 from animals) based on specific clinical criteria. Human samples were collected from patients diagnosed with bacterial infections, while animal samples were obtained from livestock showing signs of infection, ensuring the clinical relevance of the samples. A random selection process was employed to minimize selection bias. The collected samples were sent to the laboratory at Atieh Salamat Alborz. The bacterial samples were cultured on Eosin-methylene blue, MacConkey agar, and blood agar and incubated at 37°C for 24 hours. Isolated colonies were subjected to Gram staining. Biochemical tests, including indole production and motility in SIM (Merck KGaA, Darmstadt, Germany) medium, lactose fermentation, carbon dioxide gas production in TSI (Merck KGaA) medium, as well as the Methyl Red and Voges–Proskauer (Merck KGaA) tests, were performed to confirm the identification of E. coli.
3.2. Extraction of Microbial Polypeptide (Bacteriocin)
Lactobacillus plantarum ATCC8014, provided by Atiyeh Salamat Alborz Company, was inoculated in MRS broth (Quelab, Canada) and incubated at 37°C in a shaker incubator for 24 hours, until reaching a turbidity equivalent to 1 McFarland standard (approximately 3 × 108 CFU/mL at 600 nm wavelength). The bacterial suspension was centrifuged at 4°C at 7000 rpm for 20 minutes, and the supernatant was filtered using a 0.2-micron filter (Shimi Teb Kala, Iran). The filtered bacteriocin extract was transferred to a sterile Falcon tube and stored at 0 - 4°C.
To ensure bacteriocin stability, 50 µL of a 1 mmol solution of phenylmethylsulfonyl fluoride (PMSF) (Tamadkala, Iran) was added to 1 mL of bacteriocin extract to inhibit protease activity. The solution was then transferred to an Amicon Ultra Centrifugal Filter (3 kDa) (Tehran Zist Protein Pajooh) and centrifuged at 4°C at 4000 rpm for three consecutive intervals of 10, 30, and 60 minutes. The concentrated bacteriocin was stored in sterile microtubes at 0 - 4°C. Protein concentration was measured using the Bradford method, followed by antimicrobial testing using minimum inhibitory concentration (MIC), minimum bactericidal concentration (MBC), disk diffusion, and agar well diffusion methods.
3.3. Quantification of Protein Concentration by the Bradford Method
The Bradford reagent was prepared by dissolving 10 mg of Coomassie Brilliant Blue (Tamadkala Company) in 5 mL of 95% ethanol. Then, 10 mL of 85% orthophosphoric acid (Tamadkala Company) was added, and the volume of the solution was brought up to 100 mL with distilled water. The resulting solution was filtered using Whatman filter paper No. 1 (Talaye Teb Azma, Iran) to remove any suspended particles and stored in a dark container in the refrigerator. The Bradford method was employed to generate a standard protein curve. Bovine serum albumin (Tamadkala, Iran) was used to prepare protein standards at different concentrations (at least three standards ranging from 20 to 200 μg/mL). Glass or polystyrene cuvettes were utilized, and the optical absorbance of each tube was measured against a blank using a spectrophotometer (Rayleigh, China) at a wavelength of 595 nm. The standard curve was then plotted using Excel and GraphPad software (17).
3.4. Determining the Minimum Inhibitory Concentration and the Minimum Bactericidal Concentration
To evaluate the effect of the bacteriocin extracted from Lactobacillus plantarum on human and animal E. coli isolates using the broth microdilution method, sterile 96-well microplates (Shimi Teb Kala, Iran) were used. First, fresh streak cultures (18 - 24 hours) of E. coli isolates were grown on Mueller-Hinton Agar (Merck KGaA). A bacterial suspension was prepared by transferring a portion of the fresh bacterial colony with a sterile loop into sterile normal saline, adjusting the turbidity to match 0.5 McFarland (1 x 108 CFU/mL). This suspension was then diluted 1:20 to reach a concentration of 5 × 10⁶ CFU/mL.
Next, 100 µL of BHI broth (Merck KGaA) was dispensed into each well of the microplate. Then, 100 µL of the prepared bacteriocin was added to the first well, with initial experiments indicating that bacteriocin at 200 µg/mL demonstrated significant inhibitory activity in this well. Serial dilutions were performed across the wells to determine the MICs and MBCs. A 100 µL aliquot of the prepared E. coli bacterial suspension, adjusted to a final concentration of 106 CFU/mL, was added to all wells except the negative and positive control wells. The negative control well contained 100 µL of BHI broth, while the positive control well contained 100 µL of BHI broth and 100 µL of the bacterial suspension. Non-bacteriocin-negative controls were included to confirm that spontaneous bacterial inhibition did not occur, and gentamicin-positive controls ensured favorable assay conditions.
To eliminate contamination concerns, a blank test using sterile distilled water was also performed. To reduce errors, each test was repeated three times. The reference E. coli strain ATCC35218 was used for further validation. The microplates were incubated for 24 hours at 37°C. After incubation, bacterial growth was assessed by comparing the wells with control wells using an ELISA reader (Parsian Tebzaman, PYTHON) at a wavelength of 600 nm. Growth was indicated by an increase in optical density (OD), whereas inhibition was indicated by minimal or no change in OD. The MIC was determined as the lowest concentration of bacteriocin that prevented an increase in OD.
To determine the MBC, 100 µL was taken from one well before and one after the MIC well and transferred onto BHI agar plates, which were then incubated to check for bacterial growth. The methods were adapted from those described by Haghighatafshar et al. in their study on the effect of bacteriocin isolated from Lactobacillus rhamnosus on Pseudomonasaeruginosa lipopolysaccharides (18).
3.5. Disc Diffusion Method
To prepare the discs containing the extracted bacteriocin, 10 microliters of the polypeptide were added to blank discs. The discs were then stored in a sterile and dry environment in sterile vials to maintain their antimicrobial properties. For the bacterial suspension preparation, a portion of the fresh bacterial colony (18 - 24 hours) was dissolved in sterile physiological serum using a sterile loop, achieving a turbidity equivalent to 0.5 McFarland (1 × 108 CFU/mL). The suspension prepared from all 20 E. coli isolates was then cultured on Mueller-Hinton agar medium.
Using sterile laboratory forceps, the bacteriocin-containing discs were placed on the agar medium. The plates were subsequently incubated for 24 hours at 37°C. To minimize error, the test was repeated three times. After incubation, the diameter of the inhibition zone around each disc (non-growth halo) was measured. For comparison, a commercial gentamicin disc (Padtan Teb©, Iran) was also placed on the plate, and the standard strain E. coli ATCC35218 was tested under identical conditions.
3.6. Agar Well Diffusion Method
A bacterial suspension with a concentration equivalent to 0.5 McFarland was prepared from each of the 20 tested E. coli isolates and the standard strain E. coli ATCC35218. The suspension was applied to the surface of Lawn culture plates. Under sterile conditions, wells with a diameter of 6 mm were created on the Mueller-Hinton agar medium. Then, 10 microliters of extracted bacteriocin were carefully added to each well. After allowing the polypeptide to be completely absorbed (approximately one hour), the plates were transferred to an incubator. After 24 hours of incubation at 37°C, the diameter of the non-growth halos (zones of inhibition) was measured and recorded.
The disc diffusion method was employed to qualitatively assess the inhibition zones produced by the bacteriocin, demonstrating its antimicrobial activity. In contrast, the agar well diffusion method allowed for a more quantitative assessment by determining the concentration of bacteriocin required to inhibit bacterial growth. The combination of these methods provided a comprehensive evaluation of the bacteriocin’s efficacy, with disc diffusion yielding general efficacy results and agar well diffusion offering insights into the specific concentrations needed for bacterial inhibition.
4. Results
In this study, 20 E. coli isolates (10 from individuals with urinary infections and 10 from cows with mastitis) were obtained from the bacterial collection of the Atiyeh Alborz Company laboratory. To ensure accuracy, the strains were verified using the biochemical and confirmation tests described in this research.
4.1. Results of the Bradford Test and Standard Curve
Lactobacillus plantarum bacteriocin was extracted using a Falcon tube containing an Amicon 3KD filter. Its concentration was determined using the Bradford method, yielding a concentration of 26.76 μg/mL. The culture results are displayed in Figure 1. Additionally, the standard curve of this protein was plotted (Figure 2).
Results of tests conducted. A, culture in EMB medium; B, Gram culture; C, TSI; D, simon citrate; E, indole; and F, methyl red.
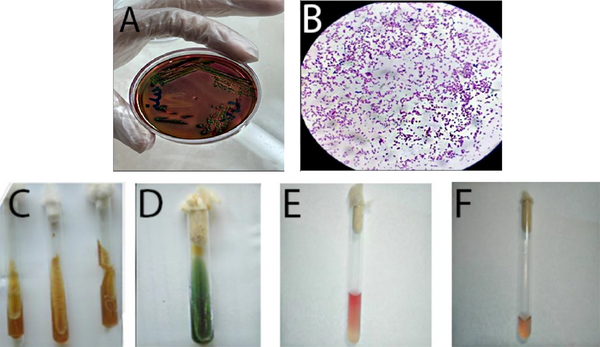
The standard curve of L. plantarum bacteriocin.
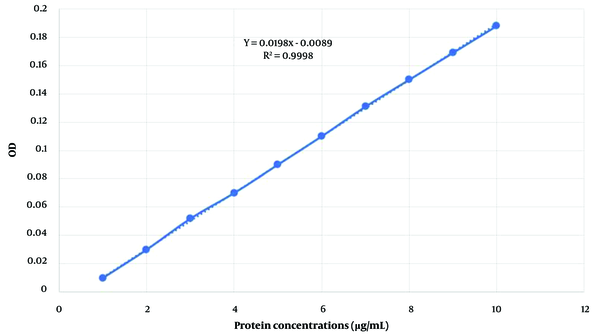
4.2. The Results of Minimum Inhibitory Concentration and Minimum Bactericidal Concentration
The results of the MIC and MBC tests on human and animal E. coli strains, as well as the standard E. coli strain ATCC35218, after exposure to bacteriocin, were determined both visually and using an ELISA device. The data revealed that the growth of these isolates was inhibited at a bacteriocin concentration of 13.38 μg/mL. The MBC for these strains was found to be 26.76 μg/mL. Interestingly, only one human strain (sample 6) and two animal strains (samples 3 and 10) exhibited different MIC (6.69 μg/mL) and MBC (13.38 μg/mL) values compared to other E. coli strains and the standard strain. The ELISA plot provides a clear depiction of the MIC and MBC values for the animal E. coli isolates after exposure to bacteriocin extracted from L. plantarum (Table 1).
ELISA Plot Showing the Minimum Inhibitory Concentration and Minimum Bactericidal Concentration Values of Animal Escherichia coli Isolates After Exposure to Bacteriocin Extracted from Lactobacillus plantarum
Row | Sample Code | Source | MIC (µg/mL) | MBC (µg/mL) |
---|---|---|---|---|
1 | 1402/p-19-11 | Animal | 13.38 | 26.76 |
2 | 1402/p-19-12 | Animal | 13.38 | 26.76 |
3 | 1402/p-19-13 | Animal | 6.69 | 13.38 |
4 | 1402/p-19-14 | Animal | 13.38 | 26.76 |
5 | 1402/p-19-15 | Animal | 13.38 | 26.76 |
6 | 1402/p-19-16 | Animal | 13.38 | 26.76 |
7 | 1402/p-19-17 | Animal | 13.38 | 26.76 |
8 | 1402/p-19-18 | Animal | 13.38 | 26.76 |
9 | 1402/p-19-19 | Animal | 13.38 | 26.76 |
10 | 1402/p-19-20 | Animal | 6.69 | 13.38 |
- | E. coli ATCC35218 | Standard strain | 13.38 | 26.76 |
4.3. Bacteriocin Evaluation Resulsuppts by Disc Diffusion Method
The results demonstrated that all human and animal strains, including the standard E. coli strain ATCC35218, were resistant to the bacteriocin extracted from L. plantarum ATCC8014. The extracted polypeptide was unable to inhibit or destroy the target strains. Additionally, no growth inhibition halo was observed around the gentamicin disc, further indicating the high resistance of the strains to both bacteriocin and gentamicin treatments. The disc diffusion results of bacteriocin and gentamicin on E. coli ATCC35218 are illustrated in Figure 3.
The disc diffusion results of bacteriocin and gentamicin on Escherichia coli ATCC35218
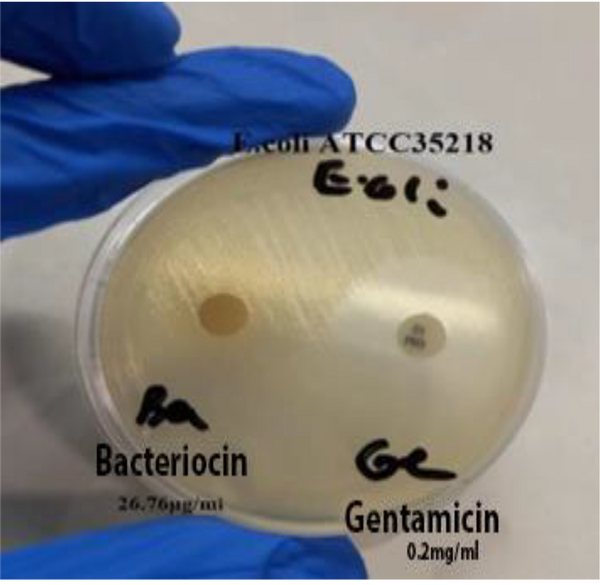
4.4. Bacteriocin Evaluation Results by Agar Well Diffusion Method
The results of this study revealed that all human and animal E. coli strains, including the standard strain E. coli ATCC35218, were resistant to bacteriocin at a concentration of 26.76 μg/mL. The findings suggest that the bacteriocin extracted in this research did not exhibit strong antimicrobial activity against the studied strains, with all strains demonstrating resistance. Both the disc diffusion and agar well diffusion methods indicated no antimicrobial activity, likely due to E. coli’s protective lipopolysaccharide layer and potential resistance mechanisms, which could hinder the bacteriocin's ability to penetrate and exert its effects.
5. Discussion
Recently, bacteriocin, a natural antimicrobial peptide, has been widely studied as a potential alternative to chemical preservatives. Bacteriocin-producing strains can also be used as probiotics (19, 20). Bacteriocins can serve as food additives and be applied in the treatment of pathogen-related diseases and cancer. Infectious diseases caused by pathogenic strains of gram-positive bacteria such as Staphylococcus, Micrococcus, and Streptococcus can be prevented with bacteriocins. Additionally, many gram-negative bacteria, including E. coli, Salmonella, Shigella, Listeria, and Vibrio, have been tested to explore the antagonistic activity of newly isolated antimicrobial peptides (1). Notably, bacteriocins can inhibit or kill phylogenetically related or unrelated microorganisms but do not have an antimicrobial effect on the bacteriocin-producing strains themselves.
It has been suggested that the resistance of E. coli strains to gentamicin results from the acquisition of specific resistance genes that encode aminoglycoside-modifying enzymes. In research conducted by Keikha and Rava in 2016, the antibiotic resistance of E. coli strains isolated from patients with urinary infections was investigated. Among the 78 E. coli samples isolated, 13.7% were resistant to gentamicin (21), which differs from the findings of the present study regarding the resistance percentage. In the current research, the disc and well diffusion methods showed that no growth halo was formed around the gentamicin disc, and all strains (100%) were resistant to gentamicin, indicating a high level of resistance in the studied strains.
In 2023, Wang et al. extracted a new bacteriocin from L. plantarum and investigated its biological properties. Their analysis of the metabolite, named plantaricin w3-2, showed that it possesses broad antimicrobial potential and good pH stability (22). Similarly, in a study conducted by Li et al. in 2023, a new bacteriocin produced by L. plantarum FB-2 was purified, and its antimicrobial mechanism against Staphylococcusaureus was explored. Their results demonstrated that this bacteriocin had strong antimicrobial activity, functioning by disrupting the bacterial cell membrane structure, increasing cell permeability, promoting cytoplasmic content leakage, and raising intracellular reactive oxygen species levels (23). However, the current research revealed that the bacteriocin extracted from L. plantarum ATCC8014 did not display broad antimicrobial activity against the tested E. coli strains, which highlights a contradiction between the findings of this study and previous research.
Hassan et al.'s study in 2020 aimed to identify bacteriocins extracted (using the organic solvent extraction method) from twelve Lactobacillus species found in yogurt and evaluate their bactericidal effects on foodborne bacteria. Bacteriocins produced by Lactobacillus helveticus and L. plantarum demonstrated the most antimicrobial activity, particularly against S. aureus and Acinetobacter baumannii. Both bacteriocins were active in acidic pH, with L. plantarum bacteriocin showing heat stability, unlike L. helveticus bacteriocin. Proteolytic enzyme treatments confirmed the protein nature of both bacteriocins. The findings suggest that L.helveticus bacteriocin may be more effective against food pathogens than L. plantarum bacteriocin (1). In contrast to Hassan et al.'s findings, the results of our study showed that the microbial polypeptide extracted from L. plantarum ATCC8014 did not exhibit broad antimicrobial properties against the tested E. coli strains (1).
Similarly, Zangeneh et al.'s 2020 study aimed to isolate and partially characterize bacteriocin produced by L.plantarum species from traditional sourdough. After partial purification, the bacteriocin was tested under harsh conditions, and its antibacterial activity against Listeriamonocytogenes was evaluated as an indicator. Their results demonstrated that the bacteriocin exhibited primarily bacteriostatic activity, significantly inhibiting the growth of pathogens such as S.aureus, E. coli, and Listeria monocytogenes (24).
An analysis of past and current research reveals contradictions in findings, which may stem from various factors, including climatic and geographical differences, as well as the presence of different serotypes causing infections in varying populations. The differences in sample separation and identification methods may also play a role. However, the pathogenic factors in E. coli strains responsible for urinary infections, as well as the excessive and long-term use of antibiotics, particularly in livestock farms, likely contribute to the severity and spread of infections, making treatment and disease control more challenging.
5.1. Conclusions
Despite testing several E. coli strains with bacteriocin produced by L. plantarum ATCC8014, no antimicrobial effects were observed. The growing concern over antibiotic resistance, along with resistance to bacteriocin and gentamicin in these strains, underscores the urgent need for alternative treatment approaches. Combating drug-resistant E. coli may require other antimicrobial agents or combinations of treatments. Further detailed research is needed to explore their potential effectiveness.
References
-
1.
Hassan MU, Nayab H, Rehman TU, Williamson MP, Haq KU, Shafi N, et al. Characterisation of Bacteriocins Produced by Lactobacillus spp. Isolated from the Traditional Pakistani Yoghurt and Their Antimicrobial Activity against Common Foodborne Pathogens. Biomed Res Int. 2020;2020:8281623. [PubMed ID: 33005689]. [PubMed Central ID: PMC7503110]. https://doi.org/10.1155/2020/8281623.
-
2.
Bintsis T. Lactic acid bacteria as starter cultures: An update in their metabolism and genetics. AIMS Microbiol. 2018;4(4):665-84. [PubMed ID: 31294241]. [PubMed Central ID: PMC6613329]. https://doi.org/10.3934/microbiol.2018.4.665.
-
3.
Aritonang S, Elly R, Afriani S. Short Communication: Application of bacteriocin from Lactobacillus plantarum SRCM 1 004 34 strain isolated from okara as a natural preservative in beef sausage. Biodiversitas J Biological Diversity. 2020;21(5). https://doi.org/10.13057/biodiv/d210553.
-
4.
Le B, Yang SH. Efficacy of Lactobacillus plantarum in prevention of inflammatory bowel disease. Toxicol Rep. 2018;5:314-7. [PubMed ID: 29854599]. [PubMed Central ID: PMC5977373]. https://doi.org/10.1016/j.toxrep.2018.02.007.
-
5.
Tiwari SK; Anjana. Bacteriocin-Producing Probiotic Lactic Acid Bacteria in Controlling Dysbiosis of the Gut Microbiota. Front Cell Infect Microbiol. 2022;12:851140. [PubMed ID: 35651753]. [PubMed Central ID: PMC9149203]. https://doi.org/10.3389/fcimb.2022.851140.
-
6.
Fong W, Li Q, Yu J. Gut microbiota modulation: a novel strategy for prevention and treatment of colorectal cancer. Oncogene. 2020;39(26):4925-43. [PubMed ID: 32514151]. [PubMed Central ID: PMC7314664]. https://doi.org/10.1038/s41388-020-1341-1.
-
7.
Morales-Lopez S, Yepes JA, Prada-Herrera JC, Torres-Jimenez A. Enterobacteria in the 21st century: a review focused on taxonomic changes. J Infect Dev Ctries. 2019;13(4):265-73. [PubMed ID: 32045369]. https://doi.org/10.3855/jidc.11216.
-
8.
Jenkins C, Rentenaar RJ, Landraud L, Brisse S. Enterobacteriaceae. Infect Dis. 2017;2. https://doi.org/10.1016/B978-0-7020-6285-8.00180-5.
-
9.
Janda J, Abbott Sharon L. The Changing Face of the Family Enterobacteriaceae (Order: “Enterobacterales”): New Members, Taxonomic Issues, Geographic Expansion, and New Diseases and Disease Syndromes. Clinical Microbiology Reviews. 2021;34(2):10.1128/cmr.00174-20. https://doi.org/10.1128/cmr.00174-20.
-
10.
M. Basavaraju, B. S. Gunashree. Escherichia coli: An Overview of Main Characteristics. In: Marjanca Starčič E, editor. Escherichia coli. Rijeka: IntechOpen; 2022. Ch. 1 p. https://doi.org/10.5772/intechopen.105508.
-
11.
Flores-Mireles AL, Walker JN, Caparon M, Hultgren SJ. Urinary tract infections: epidemiology, mechanisms of infection and treatment options. Nat Rev Microbiol. 2015;13(5):269-84. [PubMed ID: 25853778]. [PubMed Central ID: PMC4457377]. https://doi.org/10.1038/nrmicro3432.
-
12.
O'Brien VP, Hannan TJ, Nielsen HV, Hultgren SJ. Drug and Vaccine Development for the Treatment and Prevention of Urinary Tract Infections. Microbiol Spectr. 2016;4(1). [PubMed ID: 26999391]. [PubMed Central ID: PMC4887100]. https://doi.org/10.1128/microbiolspec.UTI-0013-2012.
-
13.
Zhou Y, Zhou Z, Zheng L, Gong Z, Li Y, Jin Y, et al. Urinary Tract Infections Caused by Uropathogenic Escherichia coli: Mechanisms of Infection and Treatment Options. Int J Mol Sci. 2023;24(13). [PubMed ID: 37445714]. [PubMed Central ID: PMC10341809]. https://doi.org/10.3390/ijms241310537.
-
14.
Zaatout N. An overview on mastitis-associated Escherichia coli: Pathogenicity, host immunity and the use of alternative therapies. Microbiol Res. 2022;256:126960. [PubMed ID: 35021119]. https://doi.org/10.1016/j.micres.2021.126960.
-
15.
Ruegg PL. A 100-Year Review: Mastitis detection, management, and prevention. J Dairy Sci. 2017;100(12):10381-97. [PubMed ID: 29153171]. https://doi.org/10.3168/jds.2017-13023.
-
16.
Vangroenweghe F, Duchateau L, Burvenich C. Short communication: J-5 Escherichia coli vaccination does not influence severity of an Escherichia coli intramammary challenge in primiparous cows. J Dairy Sci. 2020;103(7):6692-7. [PubMed ID: 32331898]. https://doi.org/10.3168/jds.2019-17799.
-
17.
Ernst O, Zor T. Linearization of the bradford protein assay. J Vis Exp. 2010;(38). [PubMed ID: 20386536]. [PubMed Central ID: PMC3164080]. https://doi.org/10.3791/1918.
-
18.
Haghighatafshar H, Talebi R, Tukmechi A. The Effect of Bacteriocin Isolated From Lactobacillus rhamnosus on Pseudomonas aeruginosa Lipopolysaccharides. Avicenna J Clin Microbiol Infect. 2021;8(2):45-50. https://doi.org/10.34172/ajcmi.2021.09.
-
19.
Luong HX, Thanh TT, Tran TH. Antimicrobial peptides - Advances in development of therapeutic applications. Life Sci. 2020;260:118407. [PubMed ID: 32931796]. [PubMed Central ID: PMC7486823]. https://doi.org/10.1016/j.lfs.2020.118407.
-
20.
Simonson AW, Aronson MR, Medina SH. Supramolecular Peptide Assemblies as Antimicrobial Scaffolds. Mol. 2020;25(12). [PubMed ID: 32545885]. [PubMed Central ID: PMC7355828]. https://doi.org/10.3390/molecules25122751.
-
21.
Keikha M, Rava M. [Trend of antibiotic resistance of Escherichia coli strains isolated from urinary tract infections in outpatient patients from Zahedan]. J Paramedical Sci Rehabil. 2017;6(4):73-8. FA. https://doi.org/10.22038/jpsr.2017.21755.1556.
-
22.
Wang Z, Zhang Y, Chen C, Fan S, Deng F, Zhao L. A novel bacteriocin isolated from Lactobacillus plantarum W3-2 and its biological characteristics. Front Nutr. 2022;9:1111880. [PubMed ID: 36704783]. [PubMed Central ID: PMC9872010]. https://doi.org/10.3389/fnut.2022.1111880.
-
23.
Li Y, Yu S, Weng P, Wu Z, Liu Y. Purification and antimicrobial mechanism of a novel bacteriocin produced by Lactiplantibacillus plantarum FB-2. LWT. 2023;185:115123. https://doi.org/10.1016/j.lwt.2023.115123.
-
24.
Zangeneh M, Khorrami S, Khaleghi M. Bacteriostatic activity and partial characterization of the bacteriocin produced by L. plantarum sp. isolated from traditional sourdough. Food Sci Nutr. 2020;8(11):6023-30. [PubMed ID: 33282254]. [PubMed Central ID: PMC7684595]. https://doi.org/10.1002/fsn3.1890.