Abstract
Background:
An essential factor in the validity of motor evoked potential (MEP)s recorded by transcranial magnetic stimulation (TMS) over multiple times is their test-retest reliability which to a large extent depends on the accuracy and competence of the assessor (intra-rater reliability). However, intra-rater reliability is infrequently reported in TMS studies suggesting that this is rarely done.Objectives:
This study was conducted to determine the intra-rater within and between-session reliability of a newly trained TMS assessor prior to a main TMS study and report on the methodology used to encourage similar practice.Methods:
Fourteen (10 males, 4 females; mean age: 32 ± 5.8 years) participants took part in the study. Motor evoked potentials were elicited from a relaxed, right first dorsal interosseous (FDI) muscle three times (T1, T2 and T3) across two testing sessions at least 48 hours apart. During the first session, MEPs were recorded twice (T1 and T2) within an interval of 20 minutes to determine the within (intra) session reliability of the assessor. During the second session, a single measurement was carried out (T3) which was compared to T1 to determine the inter-session reliability.Results:
Repeated measure analysis of variance (ANOVA) did not reveal significant difference in the amplitude of the MEPs obtained across the three time periods (P = 0.196) demonstrating agreement in the MEPs and hence the reliability of the assessor. Additionally, the intraclass correlation coefficient (ICC) between T1 and T2; and T1 and T3 were 0.952 (P < 0.001) and 0.833 (P = 0.001) respectively further indicating the within and between sessions reliability of the assessor.Conclusions:
The agreement between the three measured MEPs amplitude and the significant ICC demonstrates the reliability of the assessor in this study to use TMS for research. We suggest that the intra-rater reliability of new TMS operators should be established using the methodology in this report prior to main TMS studies.Keywords
Motor Evoked Potentials Transcranial Magnetic Stimulation Intra-rater Reliability Corticospinal Excitability
1. Background
Transcranial magnetic stimulation (TMS) is a non-invasive technique used to deliver magnetic fields across the scalp and skull to activate the neurons within the brain (1). When applied at sufficient intensity over the primary motor cortex (M1), TMS produces a twitch in the muscle corresponding to the stimulated part of the M1 which can be recorded as a motor evoked potential (MEP) using surface electromyography over the target muscle (1, 2). Motor evoked potentials provide an important measure of corticospinal excitability (CSE) or the responsiveness of the corticospinal neurons and pathways (3). Components of the MEP such as the MEP amplitude have been used as outcome measures for the assessment of CSE changes in different areas of research relating to the central nervous system (4). They are employed in clinical research to comprehend the alterations in the functional integrity of the corticospinal pathway caused by brain diseases and/or recovery, with the aim of developing potential diagnostic, prognostic, and treatment monitoring parameters for these conditions (5, 6). Additionally, research using interventions like non-invasive brain stimulation, exercise training, and other rehabilitation techniques to modulate brain function and improve human performance frequently rely on measures of corticospinal excitability as biomarkers (7-11). These biomarkers are instrumental in comprehending the neuroplastic changes in the brain that underlie the functional improvements linked to these interventions (2, 10-13). Similarly, CSE serves as a valuable tool for understanding the physiology behind several sensory motor processes and how they are influenced by factors such as age, gender, sleep, arousal, motivation, and fatigue (2, 14-18).
A common feature of the studies using TMS to assess CSE for different research purposes is the need to carry out repeated measurements of CSE parameters within and across different sessions of the studies (4). For instance, the use of TMS to understand the changes in the functional integrity of the corticospinal neurons after certain rehabilitation techniques aimed at improving functional abilities of patients with brain diseases would require multiple assessments of the CSE (10-12). These assessments encompass pre-to-post intervention measurements of the CSE, as well as measurements at different time periods after the experimental and control interventions over several weeks or months to enable appropriate comparisons that would reveal the positive effects or otherwise of the interventions (9-11). This need for multiple assessments brings the question of the test-retest reliability of the CSE measures especially in relation to the intra-rater reliability or the reliability of the person using TMS to elicit MEPs (4). In many ways, this concern becomes particularly pertinent when the individual is new to the TMS device and technique. Several factors, including the TMS device, TMS device operator, participants, and recording or analysis set-up, can influence the elicited MEPs in TMS research (4, 19, 20). It is therefore crucial to tightly control these factors to ensure that observed changes in MEPs over time accurately reflect the effects of the research intervention rather than errors resulting from these factors (4). The validity and reliability of the TMS device is usually already assessed and confirmed by the manufacturer before making it commercially available (4). Therefore, the test-retest reliability of TMS induced MEPs for assessment of CSE is largely dependent on the remaining three factors (4, 19, 20). In addition to ensuring that the TMS rater has avoided any errors that may affect the elicited MEPs during the measurement, he/she also has a duty to ensure adequate control of the participant’s related factors as well as the set-up related factors during both the recording and analysis phases (4). Thus, the TMS operator has a central role to play in controlling the remaining factors that may affect the accuracy of the elicited MEPs in studies involving repeated TMS. However, in the majority of TMS studies, the intra-rater reliability is rarely reported suggesting that this may not be done in most studies (4). Given that researchers, especially graduate students, who are using TMS to induce MEPs for various research objectives, might have limited familiarity with both the device and the technique, it becomes necessary to adequately train them and also assess the reliability of the MEPs they evoke during preliminary sessions (both within session and across different sessions), before embarking on their primary research studies. In view of this, the main aim in this study was to investigate the intra-rater within- and between-session reliability of TMS induced MEPs for assessment of CSE by a newly trained operator. Furthermore, we present the methodology used and discussed the limitations of not ensuring the reliability of newly trained TMS operators. This could significantly contribute to promoting analogous practices, thereby improving the precision of changes in corticospinal excitability when conducting repeated measurements in various research types that involve transcranial magnetic stimulation.
2. Methods
2.1. Training of the New Operator (Rater)
The new rater in this study is a PhD student who will use TMS to study the effects of a particular intervention on corticospinal excitability as part of his PhD research. Considering that the new rater has no prior experience in using TMS, there is a need to adequately train him and establish the reliability of MEPs he evoked both within and across sessions prior to the main study. As a first step, the rater was equipped with adequate theoretical knowledge of the TMS through several teaching and demonstration sessions over a two-week period (3 × 2 hours sessions per week) (19, 20). During these sessions, several topics including the basic principles of transcranial magnetic stimulation, the procedure for assessment of corticospinal and cortico-cortical excitability using single and paired pulse TMS, safety considerations when using TMS, as well as the various TMS parameters and CSE outcome measures were described to the rater.
Subsequently, the rater was taken through practical sessions where he conducted the various steps that he learnt during the theoretical sessions. These sessions were repeated six times over another two weeks period under close supervision of the laboratory head up to the point where the rater was deemed confident in TMS assessment of CSE (19, 20). Thereafter, the rater was allowed to independently perform other practical sessions on as many people as possible over another four weeks to further build his competence and confidence in the assessment method (19, 20). From this point, the reliability study was started to assess the intra and inter-session reliability of the rater.
2.2. Participants
Fourteen (10 males, 4 females; age: 32 ± 5.8 years) participants attended two TMS sessions at least 48 hours apart (19, 20). All participants were right-handed and had no known neurological or psychological symptoms. Participants were also not taking any medications (including caffeine) that could influence corticospinal excitability. The participants completed a TMS safety questionnaire before the first session to ensure that they were safe for the study procedures, including questions relating to previous history of seizure or epileptic fit, presence of metal in the head such as surgical clip and other implanted devices such as cardiac pacemaker. Written informed consent was provided by each participant prior to inclusion in the study and all study procedures were conducted in accordance with the Helsinki declaration. The study was approved by the Monash University Human Research Ethics Committee (project ID: 27394).
2.3. Procedure
In the first session, two TMS assessments were conducted with an interval of 20 minutes between the first (T1) and the second (T2) assessments. Throughout the 20-minute resting period, participants were instructed to refrain from any contraction of the target muscle (right first dorsal interosseous (FDI) muscle) as well as any hand or finger movements. Participants subsequently attended the second session for the third TMS assessment (T3), after a minimum gap of 48 hours (2 days) from the initial session (Figure 1).
Study set-up. T1 = First transcranial magnetic stimulation (TMS) assessment of corticospinal excitability (CSE), T2 = Second TMS assessment of CSE, T3 = Third TMS assessment of CSE. Intra-session reliability = Reliability of the motor evoked potentials (MEPs) elicited at T1 and T2. Inter-session reliability = Reliability of the MEPs elicited at T1 and T3.
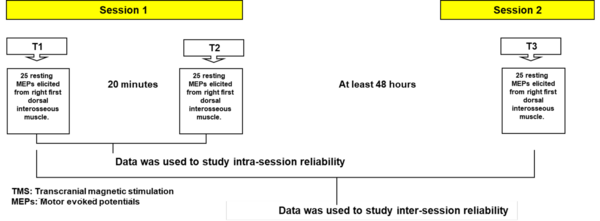
The first and second assessments (T1 and T2) were used to investigate the intra-session reliability of the rater, while the first and third assessments (T1 and T3) were used to assess the inter-session reliability of the rater (19, 20). In this way, the intra-rater within and between sessions reliability was established. Participants were asked to ensure adequate sleep the night before, avoid drugs that may affect their CNS function as well as participation in rigorous physical activity before and in between the two sessions. Before each session, participants were asked questions relating to these factors to ensure adequate compliance (4). Additionally, all assessments were done at approximately the same time of the day to control circadian effects.
2.4. Electromyography
During each session, participants were positioned comfortably on a chair to ensure that they were relaxed, with their head well positioned and the right forearm supported in a pronated position on the arm rest of the chair (20, 21). To ensure good surface contact and minimize skin resistance, the participant’s skin over the FDI muscle and the dorsum of the hand was abraded and cleaned (22). First dorsal interosseous location was determined through palpation during a manually resisted abduction of the second digit (20). Two pre-gelled self-adhesive bipolar Ag/AgCl disposable surface electrodes were then placed over the FDI with an inter-electrode distance of 2 cm and a ground electrode was placed over the dorsum of the hand. These electrodes were used to record the electromyographic (EMG) activity from the FDI during induction of MEPs. First dorsal interosseous was chosen because the newly trained rater is going to elicit MEPs from the same muscle in his subsequent study using TMS. This muscle is commonly used to evaluate CSE due to the strong corticospinal projection to the spinal motor neuron pool innervating it and therefore ease of stimulation (23). Electromyographic signals were filtered (10 – 500 Hz), amplified (× 1000) and sampled at 1000 Hz. All data were recorded on PC using LabChartTM software (ADInstruments, Australia) via a laboratory analogue-digital interface (PowerLab, ADInstruments, Australia) for later off-line analysis.
2.5. Induction of MEPs by TMS
During each session, MEPs were elicited from the right FDI at rest through single pulse magnetic stimulation of the right FDI M1 administered via 70 mm figure-of-eight magnetic coil (Magstim Company Limited, UK). The vertex was first located by measuring the mid-point between the nasion and the inion and the two preauricular areas. 5 cm was then measured medio-laterally from the vertex to locate part of the left (dominant) M1 corresponding to the right FDI. The coil was then placed over this area and oriented at 45° to the midline with the handle of the coil pointing backward to induce current flow in the posterior-anterior direction (20, 24). The coil was then carefully moved by about 1 cm in each one of these four directions (i.e., anteriorly, posteriorly, medially, and laterally) eliciting 3 MEPs in each site and taking note of the size of the MEP (226). The site that produced MEPs with largest peak-to-peak amplitude was used as the hotspot for the stimulation of the target muscle (FDI) (227). This area was marked with a non-permanent marker to ensure consistent placement of the coil throughout the entire session (20). Subsequently, the marked was cleaned with wipes after each session.
The resting motor threshold (RMT) was then determined through Adaptive threshold-hunting based on parameter estimation by sequential testing (PEST) method using a freely available computer program called motor threshold assessment tool (MTAT). The stimulator output (test intensity) was then set at 120% of the RMT and 25 MEPs with inter-pulse interval of 6 seconds were recorded during each of the three TMS assessment time points (T1, T2 and T3).
2.6. Statistical Analysis
Statistical analyses were conducted using the statistical package for the social sciences (SPSS) version 28 (IBM Corp., Armonk, NY, United States). Motor evoked potential values were calculated by measuring the peak-to-peak amplitude and the average of 25 MEPs for each data collection period (T1, T2 and T3) were calculated. The data were screened for normal distribution using the Shapiro-Wilk test and visual inspection of histograms. Because our data was found to be positively skewed, log transformation was performed to correct the skewness before conducting the analysis (20). To assess the agreement between the repeated measurements (T1, T2 and T3), one-way repeated measure analysis of variance (RM-ANOVA) was carried out. Before interpreting RM-ANOVA statistical outcomes, sphericity was verified by Mauchly’s test. Additionally, intra-class correlation coefficient (ICC) were calculated to investigate the correlation between the MEP amplitude values obtained from the three-testing periods (intra-session reliability: T1 and T2; inter-session reliability: T1 and T3). This was to determine the within- and between-session reliability of the TMS-induced MEPs by the operator. Intra-class correlation coefficient values range between 0 and 1, with larger value indicating stronger correlation.
3. Results
All participants completed both session one and two. The mean interval between the first and the second session was 6.71 ± 3.02 days. The mean RMT in the first and second sessions were 37.50 ± 7.94 and 36.79 ± 6.86 percent of the stimulator output respectively.
3.1. Reliability of TMS-evoked MEPs
3.1.1. Intra-session Reliability
The ICC between the MEP sizes at T1 and T2 was 0.952 (P < 0.001) indicating excellent correlation between the values and hence the intra-session reliability of the rater (Table 1).
Results of the Intraclass Correlation Coefficient Calculation Between the Motor Evoked Potential Amplitudes Measured at T1 and T2
Intraclass Correlation | 95 % Confidence Interval | F Test with True Value 0 | |||||
---|---|---|---|---|---|---|---|
Lower Bound | Upper Bound | Value | df1 | df2 | Sig | ||
Values | 0.952 | 0.852 | 0.985 | 21.019 | 13 | 13 | < 0.001 |
3.1.2. Inter-session Reliability
The ICC between the MEP sizes at T1 and T3 was 0.833 (P = 0.001) similarly indicating strong and significant between sessions reliability of the rater (Table 2).
Results of the Intraclass Correlation Coefficient Calculation Between the Motor Evoked Potential Amplitudes Measured at T1 and T3
Intraclass Correlation | 95 % Confidence Interval | F Test with True Value 0 | |||||
---|---|---|---|---|---|---|---|
Lower Bound | Upper Bound | Value | df1 | df2 | Sig | ||
Values | 0.833 | 0.478 | 0.496 | 5.973 | 13 | 13 | 0.001 |
3.2. Level of Agreement
One-way RM-ANOVA did not reveal significant effect of time on the measured MEP amplitudes (F (2,26) = 1.736, P = 0.196, ηp2 = 0.118). Therefore, there was agreement in the MEP amplitude obtained during the three testing periods, further indicating the reliability of the rater (Figure 2).
Motor evoked potential (MEP) amplitudes for T1, T2 and T3 presented as mean and SE.
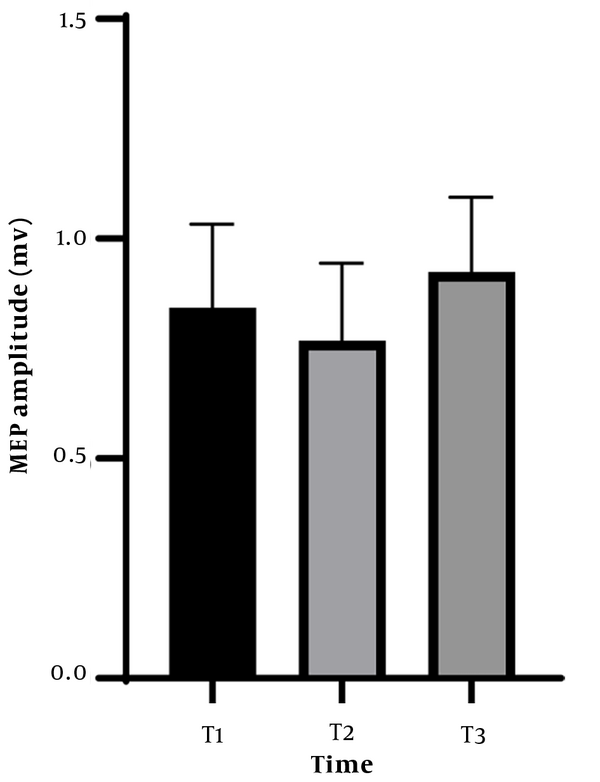
4. Discussion
The purpose of this study was to assess the intra-rater within and between session reliability of TMS-induced MEPs amplitude. Additionally, we aimed to outline the procedures followed during the assessment in order to promote similar practices among other new TMS operators.
The new rater in this study is a PhD student who will use TMS to study the effects of a particular intervention on corticospinal excitability as part of his PhD research. This study was undertaken with the objective of ensuring that any observed changes in the size of MEPs during subsequent TMS studies were genuinely attributable to the intervention rather than potential errors arising from inconsistent or suboptimal performance of the TMS technique by the rater. Results of the study revealed no significant difference between the MEP amplitudes measured at three time points indicating agreement between the three measurements. Similarly, a strong correlation was observed between the two measurements in the first session (T1 and T2) and between the first measurement in session 1 (T1) and the measurement during the second session (T3) indicating the within and between sessions reliability of the new user respectively. This is in line with the result of previous intra-rater reliability studies conducted using the same methodology described in this study (19, 20). Therefore, the methodology followed in the current and previously mentioned studies is an effective way of training and examining the intra-rater within and between session reliability of new TMS users (19, 20). In view of this, the rater in this study can be said to be reliable in assessing CSE using TMS and any changes in CSE observed in subsequent studies following intervention and assessment by the same rater can be confidently attributed to the effect of the intervention (4). Transcranial magnetic stimulation is increasingly being used to evaluate changes in corticospinal excitability in different areas of research relating to the central nervous system in both healthy and clinical populations (25-28). For instance, single and paired pulse TMS is commonly used before and after different types of interventions (e.g., other non-invasive brain stimulation techniques such as transcranial direct current stimulation and exercise training) to understand the brain mechanisms underlining the positive effects of these interventions on cognitive, motor, or affective symptoms in clinical populations in order to develop potential therapies or optimize existing treatment parameters (29-32). However, a significant aspect of the TMS-evoked MEP amplitude which is used as a measure of corticospinal excitability and brain function in these studies is its test-retest reliability (4, 20, 33). Several methodological factors can bring about significant variability in the MEP amplitudes measured at different time points using TMS thereby confounding the true effect of the intervention being investigated (4). Therefore, to ensure that any changes observed following a particular intervention truly reflect the effects of that intervention not other measurement errors, these factors need to be tightly controlled (4). Interestingly, the majority of these factors can be controlled by ensuring the reliability, competence and confidence of the TMS operator (4). Hence, it is critically important to adequately train and confirm the reliability of new TMS operators using the methodology described in this study prior to their use of TMS for assessment of CSE for research purposes. A properly trained and reliable TMS operator would be able to accurately locate the hot spot during each assessment session and ensure that the TMS coil is properly positioned over the hot spot and kept on the same spot during multiple elicitations of the MEPs (4). Indeed, any misjudgement in precise identification of the hot spot or deviations from this spot during the assessment of the CSE will greatly affect the amplitude of the elicited MEPs (34-37). This underscores the importance of ensuring the intra-rater within and between sessions reliability before repeated TMS assessment of CSE by a new user (4). Additionally, several participant’s related factors such as head movement during testing, taking certain drugs or drinks and engaging in rigorous physical exercise/training programs in between sessions may bring about significant variability in the measured MEPs (4, 38-40). While the participant may not be aware of the influence of these factors on the measured CSE, a reliable TMS operator would already be aware of these factors and control them by instructing the participant against head movement during testing and monitoring for it (4). Similarly, he/she will instruct the participant to avoid, as well as report about the use of drugs, drinks or physical training between TMS sessions that may potentially affect the elicited MEPs (4). Other important factors that may significantly affect the reliability of the measured MEPs include the number of recorded MEPs, the inter-pulse interval between the TMS pulses, Intensity of the TMS stimuli, any background contraction, and normalization of MEPs to the size of the M-wave (when the motor neuron excitability at the spinal cord level could be changed by the intervention) (4, 19, 20, 41-44). A TMS operator that passed through the rigour of a reliability study as described in this study would be familiar with these recording and analysis factors that may affect MEP amplitudes and control them before hand in the research he/she is undertaking (4). Therefore, the TMS operator is responsible directly or indirectly for controlling the various factors that may affect CSE measurement using TMS to ensure that any observed changes in research projects are truly due to the effect of the interventions used (4). Hence, with the widespread and increasing use of TMS to assess corticospinal excitability in different research settings, it is critical to establish the reliability of the TMS operator before clinical trials or other research (e.g., graduate research students using TMS) as described in this study to ensure certainty of the reported findings.
4.1. Conclusions
The agreement between the three measured MEPs and the significant ICC demonstrates the reliability of the assessor in this study to use TMS for research. We suggest that the intra-rater reliability of new TMS operators should be established using the methodology reported here prior to main TMS studies to ensure that any changes in MEPs observed across multiple measurement are truly because of the intervention used in the studies not measurement errors arising from the assessor’s handling of the equipment or participants.
References
-
1.
Zewdie E, Kirton A. TMS Basics: Single and Paired Pulse Neurophysiology. In: Kirton A, Gilbert DL, editors. Pediatric Brain Stimulation: Mapping and Modulating the Developing Brain. London: Academic Press; 2016. p. 3-22. https://doi.org/10.1016/b978-0-12-802001-2.00001-1.
-
2.
Pearce AJ, Maller JJ. Applications of MEPs. In: Jaberzadeh S, editor. A Closer Look at Motor-Evoked Potential. New York: Nova; 2018.
-
3.
Ly JQM, Gaggioni G, Chellappa SL, Papachilleos S, Brzozowski A, Borsu C, et al. Circadian regulation of human cortical excitability. Nat Commun. 2016;7:11828. [PubMed ID: 27339884]. [PubMed Central ID: PMC4931032]. https://doi.org/10.1038/ncomms11828.
-
4.
Jaberzadeh S, Zoghi M. Factors Affecting the Reliability of MEP Amplitude. In: Jaberzadeh S, editor. A Closer Look at Motor-Evoked Potential. Hauppauge, NY: Nova Science Publishers; 2019. p. 53-75.
-
5.
Valls-Sole J. Transcranial Magnetic Stimulation (TMS) Clinical Applications: Diagnostics. In: Rotenberg A, Horvath J, Pascual-Leone A, editors. Transcranial Magnetic Stimulation. Neuromethods. Vol. 89. New York, NY: Humana Press; 2014. p. 259-92. https://doi.org/10.1007/978-1-4939-0879-0_13.
-
6.
Ziemann U. Pharmaco-transcranial magnetic stimulation studies of motor excitability. In: Lozano AM, Hallett M, editors. Brain Stimulation. Handbook of Clinical Neurology. Vol. 116. Amsterdam: Elsevier; 2013. p. 387-97. https://doi.org/10.1016/b978-0-444-53497-2.00032-2.
-
7.
Bai Z, Zhang J, Fong KNK. Effects of transcranial magnetic stimulation in modulating cortical excitability in patients with stroke: a systematic review and meta-analysis. J Neuroeng Rehabil. 2022;19(1):24. [PubMed ID: 35193624]. [PubMed Central ID: PMC8862292]. https://doi.org/10.1186/s12984-022-00999-4.
-
8.
Arora T, Desai N, Kirshblum S, Chen R. Utility of transcranial magnetic stimulation in the assessment of spinal cord injury: Current status and future directions. Front Rehabil Sci. 2022;3:1005111. [PubMed ID: 36275924]. [PubMed Central ID: PMC9581184]. https://doi.org/10.3389/fresc.2022.1005111.
-
9.
Kim H, Yoo EY, Jung MY, Kim J, Park JH, Kang DH. The effects of mental practice combined with modified constraint-induced therapy on corticospinal excitability, movement quality, function, and activities of daily living in persons with stroke. Disabil Rehabil. 2018;40(20):2449-57. [PubMed ID: 28597693]. https://doi.org/10.1080/09638288.2017.1337817.
-
10.
Chaves AR, Devasahayam AJ, Riemenschneider M, Pretty RW, Ploughman M. Walking Training Enhances Corticospinal Excitability in Progressive Multiple Sclerosis-A Pilot Study. Front Neurol. 2020;11:422. [PubMed ID: 32581998]. [PubMed Central ID: PMC7287174]. https://doi.org/10.3389/fneur.2020.00422.
-
11.
Cherni Y, Tremblay A, Simon M, Bretheau F, Blanchette AK, Mercier C. Corticospinal Responses Following Gait-Specific Training in Stroke Survivors: A Systematic Review. Int J Environ Res Public Health. 2022;19(23):15585. [PubMed ID: 36497663]. [PubMed Central ID: PMC9737604]. https://doi.org/10.3390/ijerph192315585.
-
12.
Fisher BE, Wu AD, Salem GJ, Song J, Lin CH, Yip J, et al. The effect of exercise training in improving motor performance and corticomotor excitability in people with early Parkinson's disease. Arch Phys Med Rehabil. 2008;89(7):1221-9. [PubMed ID: 18534554]. [PubMed Central ID: PMC2989816]. https://doi.org/10.1016/j.apmr.2008.01.013.
-
13.
Chung CL, Mak MK, Hallett M. Transcranial Magnetic Stimulation Promotes Gait Training in Parkinson Disease. Ann Neurol. 2020;88(5):933-45. [PubMed ID: 32827221]. [PubMed Central ID: PMC8470277]. https://doi.org/10.1002/ana.25881.
-
14.
Fuglevand AJ, Lester RA, Johns RK. Distinguishing intrinsic from extrinsic factors underlying firing rate saturation in human motor units. J Neurophysiol. 2015;113(5):1310-22. [PubMed ID: 25475356]. [PubMed Central ID: PMC4346713]. https://doi.org/10.1152/jn.00777.2014.
-
15.
Uy J, Hordacre B. Variability of MEP amplitude and latency in health and disease. In: Jaberzadeh S, editor. A Closer Look at Motor-Evoked Potential. Hauppauge, NY: Nova Science Publishers; 2018. p. 33-51.
-
16.
Leocani L, Cohen LG, Wassermann EM, Ikoma K, Hallett M. Human corticospinal excitability evaluated with transcranial magnetic stimulation during different reaction time paradigms. Brain. 2000;123(Pt 6):1161-73. [PubMed ID: 10825355]. https://doi.org/10.1093/brain/123.6.1161.
-
17.
Greenhouse I, King M, Noah S, Maddock RJ, Ivry RB. Individual Differences in Resting Corticospinal Excitability Are Correlated with Reaction Time and GABA Content in Motor Cortex. J Neurosci. 2017;37(10):2686-96. [PubMed ID: 28179557]. [PubMed Central ID: PMC5354322]. https://doi.org/10.1523/JNEUROSCI.3129-16.2017.
-
18.
Salehinejad MA, Ghanavati E, Reinders J, Hengstler JG, Kuo MF, Nitsche MA. Sleep-dependent upscaled excitability, saturated neuroplasticity, and modulated cognition in the human brain. Elife. 2022;11:e69308. [PubMed ID: 35666097]. [PubMed Central ID: PMC9225005]. https://doi.org/10.7554/eLife.69308.
-
19.
Vaseghi B, Zoghi M, Jaberzadeh S. Inter-pulse Interval Affects the Size of Single-pulse TMS-induced Motor Evoked Potentials: A Reliability Study. Basic Clin Neurosci. 2015;6(1):44-51. [PubMed ID: 27504156]. [PubMed Central ID: PMC4741267].
-
20.
Pellegrini M, Zoghi M, Jaberzadeh S. The effect of transcranial magnetic stimulation test intensity on the amplitude, variability and reliability of motor evoked potentials. Brain Res. 2018;1700:190-8. [PubMed ID: 30194017]. https://doi.org/10.1016/j.brainres.2018.09.002.
-
21.
Hashemirad F, Zoghi M, Fitzgerald PB, Jaberzadeh S. Reliability of Motor Evoked Potentials Induced by Transcranial Magnetic Stimulation: The Effects of Initial Motor Evoked Potentials Removal. Basic Clin Neurosci. 2017;8(1):43-50. [PubMed ID: 28446949]. [PubMed Central ID: PMC5396172]. https://doi.org/10.15412/J.BCN.03080106.
-
22.
Gilmore KL, Meyers JE. Using surface electromyography in physiotherapy research. Aust J Physiother. 1983;29(1):3-9. [PubMed ID: 25026429]. https://doi.org/10.1016/S0004-9514(14)60659-0.
-
23.
Frazer AK, Kidgell DJ. TMS-Induced Motor Evoked Potentials: Definitions and Physiology. In: Jaberzadeh S, editor. A Closer Look at Motor-Evoked Potential. Hauppauge, NY: Nova Science Publishers; 2019. p. 1-14.
-
24.
Dissanayaka TD, Zoghi M, Farrell M, Egan G, Jaberzadeh S. Methods for Determination of Motor Threshold. In: Jaberzadeh S, editor. A Closer Look at Motor-Evoked Potential. Hauppauge, NY: Nova Science Publishers; 2019. p. 15-31.
-
25.
Brigo F, Storti M, Benedetti MD, Rossini F, Nardone R, Tezzon F, et al. Resting motor threshold in idiopathic generalized epilepsies: a systematic review with meta-analysis. Epilepsy Res. 2012;101(1-2):3-13. [PubMed ID: 22542570]. https://doi.org/10.1016/j.eplepsyres.2012.03.020.
-
26.
Nardone R, Holler Y, Brigo F, Orioli A, Tezzon F, Schwenker K, et al. Descending motor pathways and cortical physiology after spinal cord injury assessed by transcranial magnetic stimulation: a systematic review. Brain Res. 2015;1619:139-54. [PubMed ID: 25251591]. https://doi.org/10.1016/j.brainres.2014.09.036.
-
27.
Spampinato C, Aguglia E, Concerto C, Pennisi M, Lanza G, Bella R, et al. Transcranial magnetic stimulation in the assessment of motor cortex excitability and treatment of drug-resistant major depression. IEEE Trans Neural Syst Rehabil Eng. 2013;21(3):391-403. [PubMed ID: 23559064]. https://doi.org/10.1109/TNSRE.2013.2256432.
-
28.
Neige C, Rannaud Monany D, Lebon F. Exploring cortico-cortical interactions during action preparation by means of dual-coil transcranial magnetic stimulation: A systematic review. Neurosci Biobehav Rev. 2021;128:678-92. [PubMed ID: 34274404]. https://doi.org/10.1016/j.neubiorev.2021.07.018.
-
29.
Bastani A, Jaberzadeh S. Within-session repeated a-tDCS: the effects of repetition rate and inter-stimulus interval on corticospinal excitability and motor performance. Clin Neurophysiol. 2014;125(9):1809-18. [PubMed ID: 24582469]. https://doi.org/10.1016/j.clinph.2014.01.010.
-
30.
Hendy AM, Kidgell DJ. Anodal-tDCS applied during unilateral strength training increases strength and corticospinal excitability in the untrained homologous muscle. Exp Brain Res. 2014;232(10):3243-52. [PubMed ID: 24942703]. https://doi.org/10.1007/s00221-014-4016-8.
-
31.
Bolognini N, Vallar G, Casati C, Latif LA, El-Nazer R, Williams J, et al. Neurophysiological and behavioral effects of tDCS combined with constraint-induced movement therapy in poststroke patients. Neurorehabil Neural Repair. 2011;25(9):819-29. [PubMed ID: 21803933]. https://doi.org/10.1177/1545968311411056.
-
32.
Nitsche MA, Jaussi W, Liebetanz D, Lang N, Tergau F, Paulus W. Consolidation of human motor cortical neuroplasticity by D-cycloserine. Neuropsychopharmacology. 2004;29(8):1573-8. [PubMed ID: 15199378]. https://doi.org/10.1038/sj.npp.1300517.
-
33.
Ammann C, Lindquist MA, Celnik PA. Response variability of different anodal transcranial direct current stimulation intensities across multiple sessions. Brain Stimul. 2017;10(4):757-63. [PubMed ID: 28420581]. [PubMed Central ID: PMC5511514]. https://doi.org/10.1016/j.brs.2017.04.003.
-
34.
Cacchio A, Cimini N, Alosi P, Santilli V, Marrelli A. Reliability of transcranial magnetic stimulation-related measurements of tibialis anterior muscle in healthy subjects. Clin Neurophysiol. 2009;120(2):414-9. [PubMed ID: 19135412]. https://doi.org/10.1016/j.clinph.2008.11.019.
-
35.
Kamen G. Reliability of motor-evoked potentials during resting and active contraction conditions. Med Sci Sports Exerc. 2004;36(9):1574-9. [PubMed ID: 15354040]. https://doi.org/10.1249/01.mss.0000139804.02576.6a.
-
36.
Ngomo S, Leonard G, Moffet H, Mercier C. Comparison of transcranial magnetic stimulation measures obtained at rest and under active conditions and their reliability. J Neurosci Methods. 2012;205(1):65-71. [PubMed ID: 22227444]. https://doi.org/10.1016/j.jneumeth.2011.12.012.
-
37.
Thomson RH, Cleve TJ, Bailey NW, Rogasch NC, Maller JJ, Daskalakis ZJ, et al. Blood oxygenation changes modulated by coil orientation during prefrontal transcranial magnetic stimulation. Brain Stimul. 2013;6(4):576-81. [PubMed ID: 23376041]. https://doi.org/10.1016/j.brs.2012.12.001.
-
38.
Conte A, Gilio F, Iezzi E, Frasca V, Inghilleri M, Berardelli A. Attention influences the excitability of cortical motor areas in healthy humans. Exp Brain Res. 2007;182(1):109-17. [PubMed ID: 17516055]. https://doi.org/10.1007/s00221-007-0975-3.
-
39.
Minelli A, Bortolomasi M, Scassellati C, Salvoro B, Avesani M, Manganotti P. Effects of intravenous antidepressant drugs on the excitability of human motor cortex: a study with paired magnetic stimulation on depressed patients. Brain Stimul. 2010;3(1):15-21. [PubMed ID: 20633426]. https://doi.org/10.1016/j.brs.2009.04.003.
-
40.
Ziemann U, Lonnecker S, Paulus W. Inhibition of human motor cortex by ethanol. A transcranial magnetic stimulation study. Brain. 1995;118(Pt 6):1437-46. [PubMed ID: 8595475]. https://doi.org/10.1093/brain/118.6.1437.
-
41.
Biabani M, Farrell M, Zoghi M, Egan G, Jaberzadeh S. The minimal number of TMS trials required for the reliable assessment of corticospinal excitability, short interval intracortical inhibition, and intracortical facilitation. Neurosci Lett. 2018;674:94-100. [PubMed ID: 29551425]. https://doi.org/10.1016/j.neulet.2018.03.026.
-
42.
Lefebvre R, Pepin A, Louis PF, Boucher JP. Reliability of the motor evoked potentials elicited through magnetic stimulation at three sites. J Manipulative Physiol Ther. 2004;27(2):97-102. [PubMed ID: 14970810]. https://doi.org/10.1016/j.jmpt.2003.12.004.
-
43.
Taylor JL, Allen GM, Butler JE, Gandevia SC. Effect of contraction strength on responses in biceps brachii and adductor pollicis to transcranial magnetic stimulation. Exp Brain Res. 1997;117(3):472-8. [PubMed ID: 9438716]. https://doi.org/10.1007/s002210050243.
-
44.
Ugawa Y, Terao Y, Hanajima R, Sakai K, Kanazawa I. Facilitatory effect of tonic voluntary contraction on responses to motor cortex stimulation. Electroencephalogr Clin Neurophysiol. 1995;97(6):451-4. [PubMed ID: 8536598]. https://doi.org/10.1016/0924-980x(95)00214-6.