Abstract
Background:
Renal injury is the main side effect of cisplatin (CP), an anticancer drug. It has been shown that pretreatment with single-dose oxygen (0.5 to six hours) could reduce CP-induced renal toxicity in rats.Objectives:
The present study aimed to compare the effects of pretreatment with single-dose and intermittent O2 on CP-induced nephrotoxicity.Materials and Methods:
Adult male rats were allocated to seven groups (eight rats in each group). The rats were kept in normal air or hyperoxic environment (O2, 80%) for either a single six-hour period or intermittent six hours per day for seven days and then were subjected to intraperitoneal injection of saline or CP (5 mg/kg) at 48 hours, 72 hours, or seven days after exposure to O2. Three days after CP (or Saline) injection, renal function tests, renal tissue injury scores, and cleaved Caspase-3 and Bax/Bcl-2 genes expression (as markers of renal cell apoptosis) were assessed.Results:
Treatment with the 6-hour single-dose O2 reduced renal injury significantly when CP was administrated 48 hours after O2 pretreatment. Pretreatment with intermittent seven days of six hours per day had no protective effects and even relatively worsened renal injury when CP was injected 48 hours or 72 hours after the last session of O2 pretreatment. The beneficial effects of pretreatment with O2 on renal structure and function were seen if CP was administrates seven days after pretreatment with intermittent O2.Conclusions:
The pattern of pretreatment with O2 could change this potential and highly protective strategy against CP-induced nephropathy to an ineffective or even mildly deteriorating one. Therefore, O2 administration before CP injection to patients with cancer, for therapeutic purposes or as a preconditioning approach, should be performed and investigated with caution until exact effects of different protocols has been determined in human.Keywords
1. Background
It is well known that Cis-diamminedichloroplatinum (II) or cisplatin (CP) is a major chemotherapeutic drug for treatment of testicular, ovarian, and cervical cancers as well as many types of other solid tumors such as head and neck cancers (1, 2). However, nephrotoxicity, which is the main adverse effect of CP administration, limits the drug utilization in cancer treatment.
It has been proposed that CP nephrotoxicity is partially due to direct cytotoxicity caused by reactive oxygen species (ROS) and induction of apoptosis (3). To the best of our knowledge, many strategies have been applied for reduction of CP-induced nephrotoxicity in animal models and humans including hydration (4), administration of L-arginine (5) and antioxidant agents such as N-acetyl cysteine (6), melatonin (7), vitamin C and E (8), and many herbal extracts (9-11). We have shown previously that pretreatment with nearly pure oxygen (95% O2) is able to protect rat kidney from CP-induced nephropathy (12). Likewise, it has been shown in animal models that O2 pretreatment is able to attenuate ischemia-reperfusion injuries in different vital organs such as brain (13, 14), heart (15, 16), kidney (17, 18), spinal cord (19, 20), and liver (21, 22). Recently, it has been determined that hyperoxic preconditioning could reduce hypoxia-induced apoptosis in cultured mesenchymal stem cells (23). In addition, the negative effect of deceased-donor hypoxia and beneficial effects of living donor hyperoxic preconditioning on kidney graft function have been determined in some clinical studies (24, 25); however, some others failed to show such an association (26). Short-term pretreatment with O2 partially increases ROS production in different tissues (27), and it seems that these beneficial effects of pretreatment with O2 are related to upregulation of endogenous cellular defense mechanisms such as antioxidant systems and heat shock proteins (12, 20, 28).
On the other hand, a previous study showed that intermittent O2 exposure could induce more degrees of tolerance to ischemia in rat brain in comparison with prolonged O2 pretreatment (14). Moreover, delayed cardioprotective effects of hyperoxic preconditioning against ischemia-reperfusion injury could be prolonged by intermittent administration of O2 (29). It should be noted that although long-term administration of pure or nearly pure O2 could be toxic itself, short term O2 pretreatment is a safe protocol that can be easily applied in clinical practice (30).
2. Objectives
The aim of the present study was to compare the effects of single-dose (six hours) 80% O2 pretreatment with intermittent one (six hours per day for seven days) on CP-induced nephrotoxicity in a rat model. In other words, we aimed to examine whether pretreatment seven days of intermittent six hours per day O2 would enhance protective level, prolong the protective window, or worsen CP-induced nephrotoxicity in this rat model of CP-induced acute renal failure.
3. Materials and Methods
3.1. Experimental Design
A total of 56 rats were randomly allocated to seven groups with eight animals in each group: group 1, “Air + Saline”; group 2, “O2 + Saline”; group 3, “six hours of O2 and intraperitoneal CP after 48 hours (6hO2 48hLCP)”; group 4, “seven days of intermittent O2 and intraperitoneal CP after 48 hours (7dayO2 48hLCP)”; group 5, “seven days of intermittent O2 and intraperitoneal CP after 48 hours (7dayO2 72hLCP)”; group 6, “seven days of intermittent O2 and intraperitoneal CP after seven days (7dayO2 7dayLCP)”; and group 7 (control), “Air + CP”. In group 3, animals were exposed to hyperoxic environment for six hours. Four other groups, i.e. group 2 and groups 4 through 6, were kept in the hyperoxic chamber intermittently six hours per day for seven days. Groups 1 and 7 were kept in the same chambers with normal airflow six hours per day for seven days. In Groups 3, 4, and 7, 5 mg/kg CP (Sigma-Aldrich, USA) was injected intraperitoneally (IP) 48 hours after hyperoxic pretreatment and in groups 5 and 6, CP administration was done respectively 72 hours and seven days after O2 pretreatment. Groups 1 and 2 received IP normal saline instead of CP. After 48 hours of CP or saline injection, each animal was removed to a metabolic cage for 24 hours urine collection and 72 hours after CP injection, the animals were anesthetized with an IP injection of diazepam (5 mg/kg) and ketamine (50-75 mg/kg) and blood samples were obtained from the aorta. Plasma samples were kept in (-24℃) until analysis. The kidneys were removed after laparotomy and half of the left kidney of each rat was kept in 10% neutral buffered formalin after a coronal section. The other renal tissue samples were kept in (-70℃) freezer (Jal Tajhiz Co, Iran) until related experiments.
3.2. Animals and Oxygen Exposure
The used experimental protocol in this study was approved by the Lorestan University of Medical Sciences Ethics Committee. Adult male Wistar rats (241.3 ± 4.3 g) were obtained from Shahid Beheshti University of Medical Sciences animal house and had access to food and water ad libitum throughout the study. Pre-exposure to O2 was achieved by placing the rats in specific chambers (35 × 25 × 20 cm) as was described previously (12). After about ten to 15 minutes of O2 inflow, a hyperoxic environment with about 80% O2 was achieved. This was continuously monitored by an O2 meter (Lutron DO-5510, Taiwan). Animals of groups 1 and 7 (“Air + Saline” and “Air + CP”) were kept in the same chambers for similar periods but with continuous room air delivery by an aquarium air pump.
3.3. Assessment of Renal Function
Plasma urea and plasma creatinine (Cr) were respectively measured by Berthelot procedure (ZistChem kit, Iran) and Jaffe reaction (Pars Azmoon kit, Iran) using an autoanalyzer (Hitachi 902, Japan). Creatinine clearance (CLCr) as an indicator of glomerular filtration rate (GFR) was also calculated by standard formula:
(UCr × V)/PCr
In which UCr and PCr are urine and plasma Cr concentrations, respectively, and V is the volume of collected urine in 24 hours by metabolic cage. Plasma and urine sodium levels were analyzed by an electrolyte analyzer (Convergent, Germany) and fractional excretion of sodium (FENa) was calculated according to standard formula:
[(UNa × V)/(PNa × CLCr)] × 100
In which UNa and PNa are urine and plasma Na concentrations, respectively, and V is the collected urine volume in 24 hours.
3.4. Histological Assessments
Tissue samples were fixed in 10% neutral buffered formalin, embedded in paraffin, sectioned at 5 µm, and stained with hematoxylin-eosin (H&E).
Acute tubular necrosis (ATN) of epithelium was assessed in full thickness of cortex, which divided to three distinct areas including, subcapsular region (supracortex), cortex, and corticomedullary junction (subcortex) were scored according to the degree of epithelial damage using a zero through five grading system: Zero, no lesion; one, ≤ 10% ATN; two, 11% to 25% ATN; three, 26% to 50% ATN; four, 51% to 75% ATN; and five, 76% to 100% ATN.
The karyomegaly percentage was evaluated as the average percent of cells with large nuclei per each tubule, which were chosen randomly in seven fields in each divided regions of cortex.
3.5. Western Blot Analysis
About 150 mg of each rat kidney cortex was homogenized in ice cold lysis buffer (20-mM Tris buffer, pH 7.5, containing 10 µg/mL aprotinin; 1 mM phenylmethylsulfonyl fluoride, 150 mM NaCl, 5% sodium dodecyl sulfate, and 2% NP-40). The homogenate was centrifuged at 14000 rpm for 20 minutes at 4℃. The resulting supernatant was collected. Protein concentrations were measured using the Bradford method (Bio-Rad Laboratories). Then 20 μg of protein from each sample was suspended in a loading buffer, separated on a 10% polyacrylamide gel, and transferred to nitrocellulose membrane (Hybond ECL, GE Healthcare Bio-Sciences Corp. NJ, USA) via electrophoresis. Membranes were blocked with blocking buffer (5% skimmed milk, 150 mM NaCl, 20 mM Tris–HCl at pH 7.5, and 0.1% Tween 20) at 4℃ overnight. The primary used antibodies were a rabbit monoclonal Caspase-3 antibody (Cell Signaling Technology, Danvers, MA, USA; 1:1000 overnight at 4℃), a rabbit polyclonal Bax antibody, and a mouse monoclonal Bcl-2 antibody (Santa Cruz Biotechnology, Delaware Ave. Santa Cruz, USA; 1:1000 for 3 h at room temperature). After three 5-minute washing steps with washing buffer (150 mM NaCl, 20 mM Tris–HCl at pH 7.5, and 0.1% Tween 20), the membrane was incubated with a horseradish peroxidase-conjugated secondary antibody (1:150,00; GE Healthcare Bio-Sciences Corp. NJ, USA) for 60 minutes at room temperature. All antibodies were diluted in blocking buffer. After another three 5-minute washing steps with washing buffer the membrane was incubated with an ECL Western blotting system (Amersham Biosciences, Buckinghamshire, UK) and then exposed to Lumi Film Chemiluminescence Detection film (Roche Applied Science, Mannheim, Germany). Quantification was performed with relative density using LabWork, an image-processing program (UVP, Cambridge, UK). β-Actin immunoblotting (mouse monoclonal antibody from Cell Signaling Technology, Danvers, MA, USA; 1:1000) was used as a loading control.
3.6. Tissue Preparation for Antioxidant Analysis
The frozen kidney of each rat was selected for antioxidant assay. The samples were thawed and homogenized in cold phosphate buffer (0.1 M, pH 7.4, containing 5 mM EDTA) on liquid nitrogen (31) and debris were removed by centrifugation (Centrifuge 5415 R; Rotofix 32A, Germany). Supernatants were used for measurement of protein concentration, antioxidant enzymes activities, and thiobarbituric acid reactive substances (TBARS) concentration. Protein contents of tissue homogenates were determined using a colorimetric method as described by Lowry (32).
3.7. Measurement of Glutathione Peroxidase and Superoxide Dismutase Activities
Glutathione Peroxidase (GPx) and Superoxide Dismutase (SOD) activities were evaluated by spectrophotometry with Randox GPx and SOD detection kits (Randox Laboratories, Crumlin, UK) according to the manufacturer’s instructions, as was described previously (33). The activities were expressed as unit per milligram of tissue protein (U/mg protein).
3.8. Measurement of Catalase Activity
Tissue catalase (CAT) activity was assayed using the method described by Claiborne (34). The reaction mixture (1 mL) consisted of 50 mM of potassium phosphate (pH 7.0), 19 mM of H2O2, and a 20 to 50 µL of the tissue sample. The reaction was initiated by the addition of H2O2. Absorbance changes were measured at 240 nm for 30 seconds. The molar extinction coefficient for H2O2 is 43.6M−1∙cm−1. The CAT activity was expressed as the unit per milligram of tissue protein. The unit is defined as consumed micromoles of H2O2 per minute.
3.9. Measurement of Lipid Peroxidation
The amount of lipid peroxidation was indicated by the content of TBARS in renal tissue. Tissue TBARS determined by following the production of thiobarbituric acid reactive substances as described previously (35) and was reported by Aydinoz et al. (36). TBARS results were expressed as nanomole per milligram of tissue protein (nmol/mg protein).
3.10. Statistical Analysis
Pathological necrosis scores in different cortical regions were expressed as median (range) and were compared by Mann-Whitney U test. Other data were shown as mean ± standard error of means (SEM) and were compared by one-way ANOVA followed by LSD post hoc test. SPSS (v. 19, IBM Corporation, Somers, NY, USA) was used for statistical analysis and graphs were drawn by GraphPad Prism 5 (GraphPad Software, San Diego, CA). P value < 0.05 was considered as statistically significant.
4. Results
4.1. Renal Function Tests
4.1.1. Plasma Creatinine and Urea Levels and Creatinine Clearance
Three renal function tests (plasma Cr and urea levels and CLCr) showed that injection of 5 mg/kg of single-dose CP in this animal model, led to significant deterioration of kidney function in "Air+CP" group after three days in comparison to "Air+Saline" group (P < 0.005). In addition, oxygen administration per se (in its highest dose, i.e. six hours per day for seven days) had no effect on renal function and there were no significant differences between "Air+Saline" and "O2+ Saline" groups in above mentioned renal function tests.
Administration of single-dose O2 to group 3 (6hO2 48hLCP) for six hours led to considerable and significant reduction in both blood urea and Cr levels in this group in comparison with “Air + CP” (P < 0.005) but it showed no significant differences with “Air + Saline” and “O2+Saline” groups. The difference in CLCr was insignificant between “6hO2 48hLCP” and “Air + CP” groups (P = 0.089); moreover, there was no significant differences between group 3 and any of groups 1 and 2 (P = 0.18 and P = 0.47, respectively). Seven days of six hours per day administration of O2 had no significant effect on any of renal function tests if CP was injected 48 hours or 72 hours after last session of O2 administration (no differences between groups 4 or 5 and group 7). Some degree of renal function amelioration was seen if CP was administrated seven days after this protocol of O2 administration (significant or marginally significant reduction in plasma urea and Cr levels, respectively, in comparison with group 7,). CLCr in “7dayO2 7dayLCP” group was not significantly different from “Air + CP” group but it was significantly and marginally significantly less than group 1 (P = 0.015) and group 2, respectively (P = 0.063). There was an insignificant deterioration of all these three renal function indicators in groups 4 and 5 in comparison to the control group (Figure 1).
Renal Function Tests
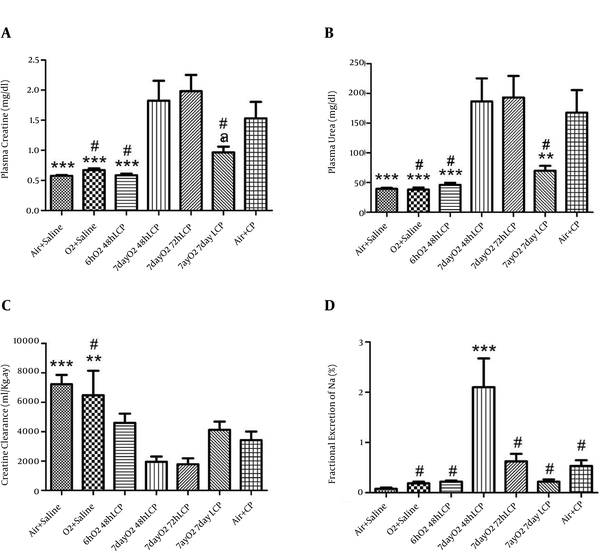
4.2. Fractional Excretion of Sodium
In comparison with control group, the changes pattern of all FENa levels in the first six groups was similar to the changes in plasma urea and Cr levels, but none of the differences were significant except that of group 4, which had a significantly higher FENa level than control group (Figure 1).
4.3. Western Blot Analysis
The results of cleaved Capase-3 gene translation and Bax/Bcl-2 ratio (as markers of renal cell apoptosis) by Western Blot Analysis were almost precisely in parallel with renal function tests, especially plasma urea and Cr levels. In other words, significant elevation of these markers of apoptosis by CP administration without O2 pretreatment (in group 7 in comparison with groups 1 and 2) and significant reduction of both of these markers in groups 3 and 6, but not in groups 4 and 5 was detected (Figure 2).
Western Blot Analysis of Cleaved Caspase-3/β-actin and Bax/Bcl2/β-actin Gene Expression
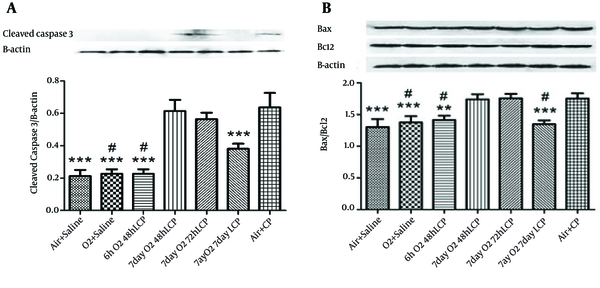
4.4. Histological Assessments
Subcapsular, cortical, and subcortical necrosis scores were significantly elevated by CP administration in group 7 in comparison with groups 1 and 2. There was also considerable and significant reduction of subcapsular and cortical necrosis scores in “6hO2 48hLCP” in comparison to control group. The reduction in subcortical necrosis score was insignificant (P = 0.1).
All three necrosis scores in group 6 were smaller than control group but the differences were insignificant. Except a significant reduction in cortical necrosis of group 4, similar to renal function tests, there were no significant differences between groups 4 or 5 in comparison with control group and even significant worsening of the subcortical necrosis in group 5 was seen.
The karyomegaly percentage was significantly higher in group 5 in comparison to all other groups and there was not any significant difference between any of other groups (Figures 3, 4 and 5).
Light Microscopy of Kidney Specimens (H & E, ×100) From Groups 2 Through 7, Respectively
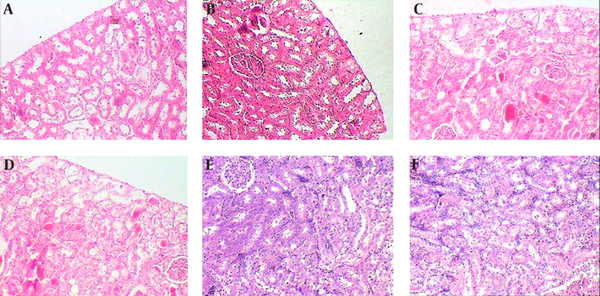
Light Microscopy of a Kidney Specimen (H&E, ×400) Showing Karyomegaly (Arrows) Three Days After Cisplatin Administration (This Was From Group 5).
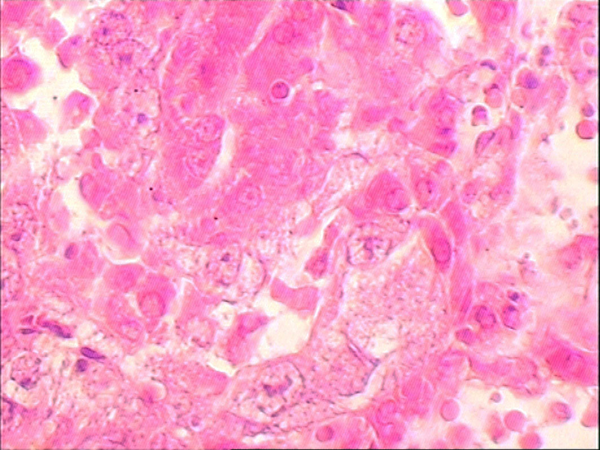
Histological Assessments
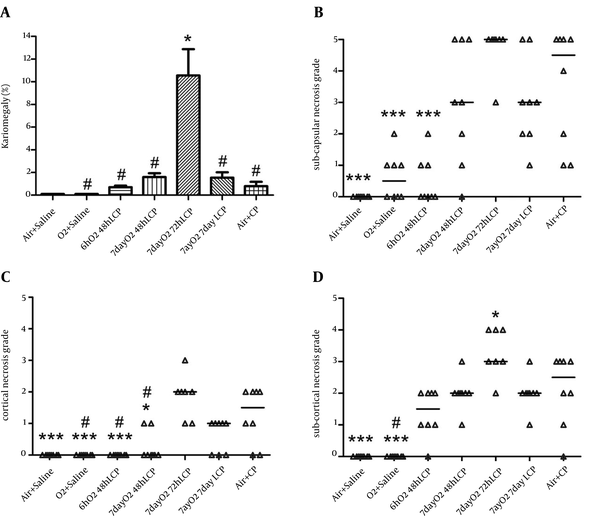
4.5. Renal Tissue Antioxidant Enzymes and Thiobarbituric Acid Reactive Substances Content
Catalase, GPx, and SOD enzymes activity levels and TBARS content are shown in Table 1. The differences between any of antioxidant enzyme levels were not significant. The content of TBARS was significantly different between groups by employing one-way ANOVA. (Between groups statistical analysis are shown in Table 1).
Some Antioxidant Enzymes Activities and Thiobarbituric Acid Reactive Substances (TBARS) in Renal Tissue a
SOD, U/mg Pr | GPX, U/mg Pr | Catalase, U/mg Pr | TBARS, nmol/mg Pr | |
---|---|---|---|---|
Air + Saline | 1.62 ± 0.1 | 1.52 ± 0.3 | 2.28 ± 0.5 | 69.52 ± 5.4 |
O2 + Saline | 2.19 ± 0.2 | 2.01 ± 0.4 | 3.92 ± 0.8 | 76.54 ± 5.5 b |
6 h O2 48 h LCP | 2.21 ± 0.3 | 1.69 ± 0.3 | 3.23 ± 0.8 | 55.85 ± 6.8 b, c |
7 day O2 48h LCP | 1.81 ± 0.1 | 1.78 ± 0.2 | 3.58 ± 0.5 | 54.40 ± 6.1 c |
7 day O2 72 h LCP | 1.80 ± 0.1 | 1.81 ± 0.3 | 3.63 ± 0.7 | 52.73 ± 5.7 c |
7 day O2 7 day LCp | 2.2 ± 0.2 | 1.64 ± 0.1 | 3.22 ± 1 | 43.61 ± 4.1 d |
Air + CP | 2.25 ± 0.2 | 1.28 ± 0.2 | 2.96 ± 0.7 | 72.67 ± 5.7 b |
5. Discussion
The present study in an in vivo rat model showed good protective effects of six-hour pretreatment with single dose of 80% O2 on CP-induced nephrotoxicity when CP was injected 48 hours after O2 administration. Pretreatment with intermittent six hours per day O2 for seven days had no protective effects and a few indicators of renal injury showed deterioration of kidney structure or function if CP was administered 48 hours or 72 hours after the last session of O2 administration. There were some degrees of protection against CP-induced renal injury when the interval between the last day of intermittent O2 and CP administration was increased to seven days. Nearly all measured parameters, i.e. renal function tests, histological injury scores, and gene expression of some indicators of cellular apoptosis, supported the above conclusion.
CP-induced renal cell death involves multiple pathways one of which is oxidant stress (36). The proposed mechanism for this beneficial effect of hyperoxic pretreatment is upregulation of endogenous defense mechanisms against ROS-induced renal injury. Indeed sublethal elevated amount of ROS, which is a result of hyperoxic exposure (37), will probably induce various protective mechanisms against ROS-induced injury in different organs. Our present data did not support the idea that these defense mechanisms are antioxidant enzymes like SOD, CAT, or GPx, because there were no significant upregulation of their activities in renal tissue of groups with protected kidneys. Of course a transient increased activity of these antioxidants and similar enzymes could be proposed, which might be disappeared on the day of assessments. Alternatively, other protective defense mechanisms such as heat shock proteins expression and anti-inflammatory mechanisms should be considered for future researches. The protective effect of O2 pretreatment (single dose 0.5 to six hours or intermittent three hours per day for two days) was shown in our previous work with favorable results of all regimes, especially for the last mentioned protocol (12). The protection in previous works was observed when administering CP 24 hours after O2 (≥ 95%) exposure (12) and the protective effect of a single-dose 80% O2 pretreatment (for two hours) was observed only after 48 hours after O2 pretreatment and disapeared after 72 hours (our unpublished data). Recently, the protective effects of hyperoxic preconditioning on hypoxia-induced apoptosis of mesenchymal stem cells has been reported (23) and we showed the protective effect of pretreatment with O2 on CP-induced injury of cultured human embryonic renal tubular cells (38). In addition, Saadat et al. showed mild amelioration of CP toxicity by hyperoxic preconditioning in patient with solid tumors. They used intermittent exposure to 60% O2 for two hours at 48, 24, and six hours before intravenous administration of CP and despite acute transient adverse effect on renal function, they reported improvement of renal function after 30 days (39). It seems that enough hyperoxia-induced ROS production has a good triggering effect on protective mechanisms. Nevertheless, these mechanisms might be decreased or even reversed if the oxidative load by O2 pretreatment is higher than a threshold level. Reduction of antioxidant pool due to prolonged or elevated level of ROS exposure might explain this paradoxical effect of single-dose versus intermittent hyperoxic pre-exposures.
Whether hyperoxia exposure has protective effects or is nonprotective and even deteriorative relates to the type of tissue and injury. For example, we did not find any protection against ischemia-reperfusion injury in rats kidney with single-dose 0.5-, one-, two-, three-, or six-hour O2 exposure (17, 18) but the protection was seen with five days of one hour per day (17) or six days of 4 hours per day (18) O2 pretreatment. Similarly, intermittent six days of four hours per day O2 pre-exposure had better ischemic-tolerance effects than a prolonged 24-hour one in a rat model of cerebral ischemia-reperfusion injury (40). In addition, delayed cardioprotective effects of a single two-hour hyperoxic exposure was prolonged by intermittent exposure (three days of two hours per day) in Baharvand et al. study (29). In this regard, daily hyperbaric O2 therapy (60 minute at 2.5 atm for six days) reduced kidney injury (41, 42) but twice daily hyperbaric O2 therapy considerably augmented CP-induced acute renal failure (41). Moreover, in agreement with some results of the present study, four days of four hour per day O2 pretreatment had no protective effect against an eight-day course of gentamycin injections, but two hour per day for four days had good effects on both renal function and tubular necrosis scores (43).
It has been well determined that CP activates the intrinsic mitochondrial pathway of apoptosis in renal epithelial cells, and Caspases including activated Caspase-3 has essential role in CP-induced apoptosis (36). In our study, there was a high correlation between renal function results and renal tissue apoptosis markers, i.e. cleaved Caspase-3 and Bax/Bcl-2 ratio gene translation.
It has been determined that in vitro and in vivo administration of CP could largely increase both necrosis and apoptosis in renal tissue (12, 44, 45). The highest degree of necrosis in renal cortex in present study was in subcapsular region with a median degree of 4.5 in a five-grade scale. Similar to apoptosis, O2 pretreatment for either a single period of six hours or six hours per day for seven days was able to reduce this subcapsular necrosis score and necrosis grade in cortical and subcortical regions. However, the results were not as significant as those of apoptosis markers and some were insignificant.
Pre-exposure to O2 with optimum method has considerable results in reducing CP-induced renal injury in animal studies and the results are largely encouraging for designing further clinical trials in patients with cancer. Although there are even some evidences in recent human studies about this beneficial effect of O2 (39), some protocols, especially intermittent ones, might have unpredicted deteriorating effects on renal function. Another important concern is that cytotoxic effects of CP are exerted through many common pathways between tumor cells and renal epithelial cells. Therefore, strategies that reduce CP-induced nephropathy might have some undesirable consequences that decrease the anti-tumor action of this useful drug (36). It seems that additional in vitro and in vivo animal studies are needed to compare the possible protective effects of O2 pretreatment between different tumoral and renal cells before designing additional human studies regarding protective effects of hyperoxic preconditioning on CP-induced renal injury. In addition, different nephrotoxic effects of CP in patients with diabetes and possibly other patients and its sex-related nature should be considered in any clinical study (46, 47).
In conclusion, our data in an in vivo rat model showed favorable protective effects of single-dose six-hour O2 (80%) pretreatment on CP-induced nephrotoxicity. In addition, we concluded that protective or nonprotective effects of intermittent O2 pre-exposure (six hours a day for seven days) depend on the time interval between O2 and CP administration.
Acknowledgements
References
-
1.
Livingston RB. Cisplatin in the treatment of solid tumors: effect of dose and schedule. J National Cancer Inst. 1989;81(10):724-5.
-
2.
Pabla N, Dong Z. Cisplatin nephrotoxicity: mechanisms and renoprotective strategies. Kidney Int. 2008;73(9):994-1007.
-
3.
Yao X, Panichpisal K, Kurtzman N, Nugent K. Cisplatin nephrotoxicity: a review. Am J Med Sci. 2007;334(2):115-24.
-
4.
Santoso JT, Lucci JA, Coleman RL, Schafer I, Hannigan EV. Saline, mannitol, and furosemide hydration in acute cisplatin nephrotoxicity: a randomized trial. Cancer Chemother Pharmacol. 2003;52(1):13-8.
-
5.
Saleh S, El‐Demerdash E. Protective effects of L-arginine against cisplatin-induced renal oxidative stress and toxicity: role of nitric oxide. Basic Clin Pharmacol Toxicol. 2005;97(2):91-7.
-
6.
Nisar S, Feinfeld DA. N-acetylcysteine as salvage therapy in cisplatin nephrotoxicity. Renal Failure. 2002;24(4):529-33.
-
7.
Sener G, Satiroglu H, Kabasakal L, Arbak S, Oner S, Ercan F, et al. The protective effect of melatonin on cisplatin nephrotoxicity. Fundam Clin Pharmacol. 2000;14(6):553-60.
-
8.
Appenroth D, Frob S, Kersten L, Splinter FK, Winnefeld K. Protective effects of vitamin E and C on cisplatin nephrotoxicity in developing rats. Arch Toxicol. 1997;71(11):677-83.
-
9.
Ali BH, Al Moundhri MS. Agents ameliorating or augmenting the nephrotoxicity of cisplatin and other platinum compounds: a review of some recent research. Food Chem Toxicol. 2006;44(8):1173-83. [PubMed ID: 16530908]. https://doi.org/10.1016/j.fct.2006.01.013.
-
10.
Beiranvand A, Rasoulian B, Alirezaei M, Hashemi P, Pilevarian AA, Ezatpour B, et al. Pretreatment with Olive Leaf Extract partially attenuates cisplatin-induced nephrotoxicity in rats. Yafteا. 2010;11(5):35-44.
-
11.
Karimi G, Khouei A, Omidi A, Kalantari M, Babaei J, Taghiabadi E, et al. Protective effect of aqueous and ethanolic extracts of Portulaca oleracea against cisplatin induced nephrotoxicity. Iran J Basic Med Sci. 2010;3(2):31-5.
-
12.
Rasoulian B, Jafari M, Mahbod M, Dehaj ME, Nowrozi M, Wahhabaghai H, et al. Pretreatment with oxygen protects rat kidney from cisplatin nephrotoxicity. Renal Failure. 2010;32(2):234-42.
-
13.
Bigdeli MR, Hajizadeh S, Froozandeh M, Heidarianpour A, Rasoulian B, Asgari AR, et al. Normobaric hyperoxia induces ischemic tolerance and upregulation of glutamate transporters in the rat brain and serum TNF-alpha level. Exp Neurol. 2008;212(2):298-306.
-
14.
Bigdeli MR, Hajizadeh S, Froozandeh M, Rasulian B, Heidarianpour A, Khoshbaten A. Prolonged and intermittent normobaric hyperoxia induce different degrees of ischemic tolerance in rat brain tissue. Brain Res. 2007;1152:228-33.
-
15.
Esmaili Dehaj M, Baharvand B, Rasoulian B, Foadaddini M, Asgari A, Noroozzadeh A, et al. Delayed protective effects of hyperoxia against cardiac arrhythmias and infarction in anesthetized rats. J Surg Res. 2009;151(1):55-61.
-
16.
Foadoddini M, Esmailidehaj M, Mehrani H, Sadraei SH, Golmanesh L, Wahhabaghai H, et al. Pretreatment with hyperoxia reduces in vivo infarct size and cell death by apoptosis with an early and delayed phase of protection. Eur J Cardiothorac Surg. 2011;39(2):233-40.
-
17.
Rasoulian B, Mohammadhosseniakbari H, Kadkhodaee M, Mofid M, Baqeri G, Bigdeli MR, et al. Preconditioning with oxygen attenuates rat renal ischemia-reperfusion injury. J Surg Res. 2008;146(2):282-8.
-
18.
Wahhabaghai H, Rasoulian B, Esmaili M, Mehrani HA, Mohammadhosseniakbari H, Mofid M, et al. Hyperoxia-induced protection against rat's renal ischemic damage: relation to oxygen exposure time. Ren Fail. 2009;31(6):514-21.
-
19.
Dong H, Xiong L, Zhu Z, Chen S, Hou L, Sakabe T. Preconditioning with hyperbaric oxygen and hyperoxia induces tolerance against spinal cord ischemia in rabbits. Anesthesiology. 2002;96(4):907-12.
-
20.
Nie H, Xiong L, Lao N, Chen S, Xu N, Zhu Z. Hyperbaric oxygen preconditioning induces tolerance against spinal cord ischemia by upregulation of antioxidant enzymes in rabbits. J Cereb Blood Flow Metab. 2006;26(5):666-74.
-
21.
Yu SY, Chiu JH, Yang SD, Yu HY, Hsieh CC, Chen PJ, et al. Preconditioned hyperbaric oxygenation protects the liver against ischemia-reperfusion injury in rats. J Surg Res. 2005;128(1):28-36.
-
22.
Liu Y, Sun XJ, Liu J, Kang ZM, Deng XM. Heme oxygenase-1 could mediate the protective effects of hyperbaric oxygen preconditioning against hepatic ischemia–reperfusion injury in rats. Clin Exp Pharmacol Physiol. 2011;38(10):675-82.
-
23.
Saini U, Gumina RJ, Wolfe B, Kuppusamy ML, Kuppusamy P, Boudoulas KD. Preconditioning mesenchymal stem cells with caspase inhibition and hyperoxia prior to hypoxia exposure increases cell proliferation. J Cell Biochem. 2013;114(11):2612-23.
-
24.
Einollahi B, Poor-Reza-Gholi F, Rezaeean S, Jahani Y, Nafar M, Najafizadeh K, et al. Deceased-donor hyperoxia deteriorates kidney graft function. Transpl Int.
-
25.
Montazeri K, Vakily M, Honarmand A, Kashefi P, Safavi M, Taheri S, et al. Short-time intermittent preexposure of living human donors to hyperoxia improves renal function in early posttransplant period: a double-blind randomized clinical trial. J Transplant. 2011;2011:204843.
-
26.
Rostami Z, Einollahi B, Ghadiani MH. Does living donor hyperoxia have an impact on kidney graft function after transplantation? Nephrourol Mon. 2013;5(3):835-9.
-
27.
Tahep ld P, Valen G, Starkopf J, Kairane C, Zilmer M, Vaage J. Pretreating rats with hyperoxia attenuates ischemia-reperfusion injury of the heart. Life Sci. 2001;68(14):1629-40.
-
28.
Bigdeli MR, Rasoulian B, Meratan AA. In vivo normobaric hyperoxia preconditioning induces different degrees of antioxidant enzymes activities in rat brain tissue. Eur J Pharmacol. 2009;611(1-3):22-9. [PubMed ID: 19303405]. https://doi.org/10.1016/j.ejphar.2009.03.034.
-
29.
Baharvand B, Dehaj ME, Foadaddini M, Rasoulian B, Poorkhalili K, Aghai HW, et al. Delayed cardioprotective effects of hyperoxia preconditioning prolonged by intermittent exposure. J Surg Res. 2010;160(1):53-9.
-
30.
Tinits P. Oxygen therapy and oxygen toxicity. Ann Emerg Med. 1983;12(5):321-8.
-
31.
Alirezaei M, Kheradmand A, Heydari R, Tanideh N, Neamati S, Rashidipour M. Oleuropein protects against ethanol-induced oxidative stress and modulates sperm quality in the rat testis. Mediterr J Nutr Metab. 2012;5(3):205-11.
-
32.
Lowry OH, Rosebrough NJ, Farr AL, Randall RJ. Protein measurement with the Folin phenol reagent. J Biol Chem. 1951;193(1):265-75.
-
33.
Alirezaei M, Jelodar G, Niknam P, Ghayemi Z, Nazifi S. Betaine prevents ethanol-induced oxidative stress and reduces total homocysteine in the rat cerebellum. J Physiol Biochem. 2011;67(4):605-12.
-
34.
Claiborne A. CRC handbook of methods for oxygen radical research: CRC Press;. 1985.
-
35.
Subbarao KV, Richardson JS, Ang LC. Autopsy samples of Alzheimer's cortex show increased peroxidation in vitro. J Neurochem. 1990;55(1):342-5.
-
36.
Miller RP, Tadagavadi RK, Ramesh G, Reeves WB. Mechanisms of cisplatin nephrotoxicity. Toxins. 2010;2(11):2490-518.
-
37.
Greger R, Windhorst U. Comprehensive Human Physiology: From Cellular Mechanisms to Integration. Berlin Heidelberg: Springer-Verlag; 1996.
-
38.
Kaeidi A, Rasoulian B, Hajializadeh Z, Pourkhodadad S, Rezaei M. Cisplatin toxicity reduced in human cultured renal tubular cells by oxygen pretreatment. Renal Failure. 2013;35(10):1382-6.
-
39.
Saadat A, Shariat Maghani SS, Rostami Z, Davoudi A, Davoudi F, Shafie A, et al. Normobaric hyperoxia preconditioning ameliorates cisplatin nephrotoxicity. Renal Failure. 2014;36(1):5-8.
-
40.
Bigdeli MR, Hajizadeh S, Froozandeh M, Rasulian B, Heidarianpour A, Khoshbaten A. Prolonged and intermittent normobaric hyperoxia induce different degrees of ischemic tolerance in rat brain tissue. Brain Res. 2007;1152:228-33.
-
41.
Aydinoz S, Uzun G, Cermik H, Atasoyu EM, Yildiz S, Karagoz B, et al. Effects of different doses of hyperbaric oxygen on cisplatin-induced nephrotoxicity. Renal Failure. 2007;29(3):257-63. [PubMed ID: 17497437]. https://doi.org/10.1080/08860220601166487.
-
42.
Atasoyu EM, Yildiz S, Bilgi O, Cermik H, Evrenkaya R, Aktas S, et al. Investigation of the role of hyperbaric oxygen therapy in cisplatin-induced nephrotoxicity in rats. Arch Toxicol. 2005;79(5):289-93. [PubMed ID: 15902426]. https://doi.org/10.1007/s00204-004-0627-3.
-
43.
Tavafi M, Ahmadvand H, Goodarzi S, Rasoulian B. Investigating the Effect of Pretreatment with Oxygen on Inhibition of Gentamycin-induced Nephrotoxicity in Rats. SSUJ. 2013;21(4):523-32.
-
44.
Lieberthal W, Triaca V, Levine J. Mechanisms of death induced by cisplatin in proximal tubular epithelial cells: apoptosis vs. necrosis. Am J Physiol Renal Physiol. 1996;270(4):F700-ّّF8.
-
45.
Ramesh G, Reeves WB. Salicylate reduces cisplatin nephrotoxicity by inhibition of tumor necrosis factor-alpha. Kidney Int. 2004;65(2):490-9.
-
46.
Soltani N, Nematbakhsh M, Eshraghi-Jazi F, Talebi A, Ashrafi F. Effect of Oral Administration of Magnesium on Cisplatin-Induced Nephrotoxicity in Normal and Streptozocin-Induced Diabetic Rats. Nephro Urol Mon. 2013;5(4):884.
-
47.
Eshraghi-Jazi F, Nematbakhsh M, Nasri H, Talebi A, Haghighi M, Pezeshki Z, et al. The protective role of endogenous nitric oxide donor (L-arginine) in cisplatin-induced nephrotoxicity: Gender related differences in rat model. J Res Med Sci. 2011;16(11):1389-96.