Abstract
Context:
During a literature search, we found data indicating how lactate affects cancer patients.Evidence Acquisition:
This review discusses metabolism in tumors, the lactate production pathway, and its effects on the host body.Result:
Research has described high lactate concentration as an undesirable clinical condition, and lactic acidosis contributes to the death of patients or some metastatic cancers.Conclusions:
Lactate can lead to angiogenesis, metastasis in the tumor, and resistance to radiation therapy and chemotherapy, especially immunosuppression. It may be possible to reduce the mortality of this disease by affecting treatment worldwide.Keywords
1. Context
Nowadays, cancer is one of the serious public health problems around the world. It is now clear that elevated lactate levels are one of the common symptoms of many cancers. This review article utilizes 18 articles from the PubMed and Google Scholar databases. Research in the field of cancer display How this compound can affect cancer patients, and perhaps by controlling its high production, it relieves a great deal in treating cancer.
Lactate, a 3-carbon hydroxyl carboxylic acid, is produced by glycolysis in the cytoplasm under anaerobic conditions from pyruvate and conversion of NADH to NAD+ (1). Up to 40% of circulating lactate can be generated by skeletal muscle, which the liver and kidney generally receive from the bloodstream and turn into glucose (2). This terminal compound of pyruvate metabolism is carried out by the lactate dehydrogenase (LDH) enzyme (3). In this review, we will present the major findings of the articles about one of the most metabolites in cancer patients.
2. Evidence Acquisition
It is commonly acknowledged that lactic acidosis is a pathological condition, with a serum lactate concentration or lactic acid remaining above 5 mmol/L, while its normal concentration is 1 to 2 mmol/L (4). Blood lactate levels reflect the balance between its production and clearance. However, lactate is produced about 1500 mmol daily under normal physiological conditions in skeletal muscle (25%), skin (25%), brain (20%), intestine (10%), and red blood cells (20%) (5) and also in patients from other tissues such as lung, leukocyte, and visceral organs (6). Furthermore, its clearance is carried out in the blood mainly by the liver (60%) and the kidneys (30%), and the heart (10%) (7). Hyperlactemia (increased lactate) can occur under hypoxic or anemia conditions, and hypolactemia (reduced lactate) due to liver or kidney and heritable disorders or due to drug toxicity (8).
The following steps occur in its oxidation: (1) Lactate is passed into the cell by the monocarboxylate transporters (MCTs) or produced within the cell; (2) LDH enzyme is required to convert lactate to pyruvate in both directions then the pyruvate enters the tricarboxylic acid (TCA) cycle (9).
It is estimated that lactate provides up to 40% of the oxidative substrate required by the heart at rest, which increases by up to 60% with activity (10). It acts as a buffer between glycolysis and oxidative metabolism that, in the process, is exchanged as fuel between cells and tissues at different glycolytic and oxidative levels (11).
It should be noted that the brain is an extremely complex organ that changes the amount of energy it needs throughout life and increases during childhood but changes throughout life due to oxidative phosphorylation (12). Although glucose is a significant substrate in normal brain tissue, it has recently been shown that this tissue has a high ability to absorb lactate after exercise (13). Monocarboxylate transporters transport lactate and pyruvate through the plasma membrane. As well as this transition was possible in both pathways (14) along with lactate transfer, which also transports protons to maintain acidity inside and outside the cell (15). Fourteen subgroups of MCT have been identified in various body tissues and reported in various cancers expressing and regulating MCT1, MCT2, MCT3, and MCT4. MCT1 and MCT4 are expressed in skeletal muscle and MCT1 in the heart (16), MCT1, MCT2, and MCT3 in the brain (17).
2.1. Cancer
It has been shown that cancer cell metabolism has changed compared to normal cells, which is known as the Warburg effect (18). In these aberrant cells, glycolytic levels were much higher than in normal tissues, and they performed anaerobic glycolysis even in large amounts of oxygen. The effect of Warburg has revealed that some cancers cause loss of mitochondrial activity (19), which reducing mitochondrial activity is a valuable advantage to maintaining cancer cell survival by inhibiting apoptosis (20). High glucose uptake is a hallmark of cancer cells providing the energy and precursor needed to biosynthesize other molecules and proliferate in cancer cells (21). The increased expression of glucose transporters and glycolytic enzymes results from oncogenic signaling and their complex transcriptional networks (22).
It is also very important to remember glutaminolysis is another major pathway for energy production in cancer cells that ultimately leads to increased lactate production and facilitates the synthesis of macromolecules in tumor cell proliferation (23). However, numerous studies have shown that cancer cells use glycolysis and mitochondrial oxidative metabolism to meet their metabolic needs (24).
2.2. Lactate and Cancer
Despite early promising data, the tumor microenvironment is a complex network of extracellular matrix molecules, soluble factors, adipocytes, and stromal cells composed of tumor endothelial cells, tumor-associated fibroblasts, and macrophages. In tumorigenesis, all of these components work together in an unusually acidic environment (19).
Among the microenvironment-soluble factors, a large amount of lactate is important for its effects on tumor and stromal cells (1). The pH of the extracellular medium decreases from 6 to 6.5, resulting in high lactate production by cancer cells (25). Pathology of the tumor microenvironment is under the influence of several key physiological factors: oxygen transport, vascular structure, nutrition, pH, and metabolite transport (26). Lactate has a critical role as the terminal compound of aerobic glycolysis in the rapid proliferation of tumor cells without resemblance to normal cells (27). Elevated lactate production is one of the dominant features of altered tumor metabolism; increased intracellular lactate must be released externally to maintain intracellular pH (28). It has been shown that this extracellular acidic environment is induced by lactate, facilitating tumor cell invasion, metastasis, and immunosuppression, increasing cell survival and resistance to apoptosis (29). On the other way, lactate is released at physiological levels at points of infection and inflammation by leukocyte activity (30). One study has shown that some cancer cells use lactate as a respiratory substrate and a lipogenic precursor in a culture medium (31). Lactate produced in hypoxic areas of the tumor can be used to generate energy by tumor cells in areas near a blood vessel (32).
It must be considered that this chemical material, as molecular signaling runs important roles in stimulating tumor inflammation and facilitating tumor angiogenesis (33). Increased lactate alters the tumor microenvironment and fuels cells of cancer cells, which are involved in acidosis, inflammation, angiogenesis, immunosuppression, and radiation resistance. Aerobic glycolysis and glutaminolysis are accepted as key markers of cancer, and both are involved in the metabolic acidosis of solid cancers that leads to the production and secretion of lactate. The extracellular pH of the tumor can be reduced to 6.5 - 6.5 instead of the pH of 7.4 in normal cells. This, in turn, is associated with weight loss, loss of appetite, and cachexia (34).
MCTs are found in the cell membranes of a variety of cells, such as tumor cells (35), erythrocytes (36), and neutrophils (37). MCT1 is expressed at low levels in most tissues, and MCT2 and MCT3 are restricted to some tissues. MCT2 is expressed predominantly in the liver, kidney, and neurons, and MCT3 in retinal epithelial pigments. High levels of MCT4 are found in white skeletal muscle fibers and, to a lesser extent, in the testes, lungs. Also, expresses MCT4 in some cells, such as chondrocytes, leukocytes, and astrocytes (38). Lactate is released by glycolytic cells, such as astrocytes, and can be transferred to other cells, such as neurons, for oxidative metabolism (39). Some tumors, such as glioma, breast, colorectal, gastric, cervical, and neuroblastoma, show increased expression of MCT1 and MCT4 (40). Of course, glioblastoma cells rely on MCT4 for proliferation and survival (41).
2.3. Lactate and Immune Cells
The previous finding demonstrated that high lactate levels have an undeniable role in stopping the entry of immune cells into the tumor, damage to the metabolism and function of cytotoxic T lymphocytes in the tumor microenvironment (42); it also prevents the release of T lymphocytes cytokines (43). It is estimated that tumor cells produce up to 40 times more lactate than normal cells (44). The tumor microenvironment contains neutrophils, macrophages, and dendritic cells that can produce lactate (45).
Neutrophils contain few mitochondria that rely on glycolysis for ATP production, while resting macrophages often metabolize glucose via glycolysis rather than oxidation but hexokinases and glucose 6 phosphate dehydrogenase are highly expressed in activated macrophages, which indicates that high pentose phosphate pathways are high in them (46). Macrophages M1 and M2 are different in terms of metabolism and immune function. M1 macrophages are the first line of defense against bacterial infections and obtain their required energy through glycolysis, and M2 macrophages use oxidative metabolism to affect tissue repair and wound healing (47). Macrophage phenotype changes from M1 to M2 during tumor progression (48).
Inactive dendritic cells use fatty acids and glucose for oxidative phosphorylation (49), but stimulation of TLR in these cells increases glucose uptake and lactate production (50), and dendritic cell activity stops when drugs inhibit glycolysis, and these cells rely on Warburg metabolism to survive (49). Stimulation of T lymphocytes results in a rapid increase in the expression of glucose transporters (GLUT1), glucose uptake, and glycolysis (51). In addition, by stimulating T lymphocytes, glutaminolysis increases and decreases fatty acid beta-oxidation (52).
Therefore, both glycolysis and glutaminolysis increase lactate production within the T lymphocytes. Several studies have shown that acidosis results in loss of function of T lymphocytes. The function of these cells can be resumed by buffering in physiological quantities (34).
B lymphocytes play an important role in antitumor immunity as antigen-presenting cells. In terms of metabolism, these cells are distinct from T-type lymphocytes that do not alter their metabolism from oxidative phosphorylation to glycolysis by stimulation. Even though these lymphocytes use both pathways, inhibition of glycolysis or deletion of GLUT1 in B lymphocytes suppresses antibody production (52).
As well, lactate alters monocyte function and consequently suppresses the immune system in the tumor (53). Studies have recently supported that NK cell activity is inhibited by lactate produced from the tumor or low extracellular pH (54).
3. Results
Tumor cells can secrete anti-inflammatory cytokines and suppress the immune cell population in the tumor microenvironment, inhibiting immune responses (55). On the other hand, the microenvironment of the tumor environment can act as a trigger for pain in cancer patients (56); it also contributes to the metastasis of some cancers (57). Lactic acid stimulates metalloproteinases-9 (MMP-9) in murine B16 melanoma (58), VEGF-A in gliomas and glioblastoma cells (59), IL-8 expression in pancreatic adenocarcinomas (60), and ovarian carcinoma cells (61). Lactate per second activates angiogenesis through the VEGF/VEGFR2 signaling pathway and stimulation of endothelial cells through MCT-1, which initiates phosphorylation/degradation of IKβ, stimulation of NF-Kβ/IL-8 pathway, which induces cell migration and new vascular formation (62). Lactate indirectly facilitates the survival of hypoxic tumor cells in newly formed areas away from blood vessels (63), and it is highly correlated with cancer malignancy and will lead to poor survival in these patients (64). Exosomes are 30- to 100-nm microscopic cells of endocytotic origin containing microRNAs, proteins, metabolic enzymes, and structural proteins. Exosomes play important roles in cancer metastasis and carcinogenesis (65). Lactate may play a key role in releasing exosomes and being absorbed by other cells (66).
Another study suggests that lactate is a marker of poor blood flow and a measure of severe disease, and in an emergency, the predicted mortality may be hyperlactemia (serum lactate greater than 4 mmol/L), metabolic acidosis, along with symptoms of tissue anemia (67). In another study, lactate is a predictor of brain tumor grade, and elevated serum lactate levels may be useful as a non-invasive biomarker, which correlates with tumor grade or range of invasion and also can determine tumor progression or response to treatment (68). Research has characterized high lactate concentration as an undesirable clinical condition (69), and lactic acidosis contributes to the death of patients with some metastatic cancers (70).
Common standard treatments such as radiotherapy or some chemotherapy drugs are the induction of reactive oxygen species (ROS), which cause DNA/RNA damage, but lactate may cause cancer cells to resist these treatments. Targeting MCT will induce apoptosis of tumor cells due to intracellular acidosis or lactate accumulation and inhibition of its uptake by aerobic tumor cells. Therefore, angiogenesis, invasion, and tumor metastasis will be reduced (71). It is well known that inhibition of MCT4 can induce lactic acid accumulation within the cell, and consequently, cell death occurs in hypoxic tumor cells (72). The feasible role of lactate can be used as a biomarker to predict survival in cancer patients (73), and a study reported that lactate may be a quantitative biomarker in response to acute radiation therapy (74).
4. Conclusions
To put it in a nutshell, lactate, the most important metabolite, lead to angiogenesis in tumors, metastasis, resistance to radiation therapy and chemotherapy, and immunosuppression (Figure 1). Encouragingly, the levels of this effective substance should be monitored carefully, and it may be possible to reduce the mortality of this disease by affecting treatment worldwide.
Roles of lactate
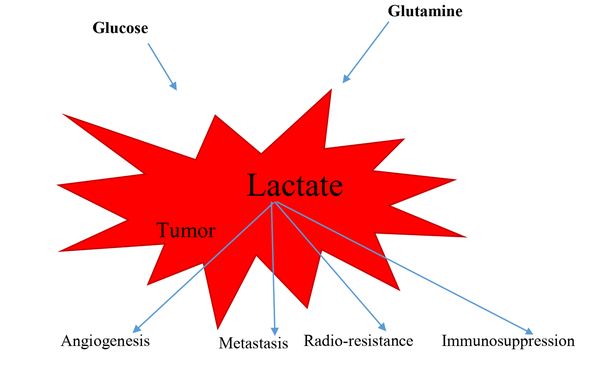
References
-
1.
Romero-Garcia S, Moreno-Altamirano MM, Prado-Garcia H, Sanchez-Garcia FJ. Lactate Contribution to the Tumor Microenvironment: Mechanisms, Effects on Immune Cells and Therapeutic Relevance. Front Immunol. 2016;7:52. [PubMed ID: 26909082]. [PubMed Central ID: PMC4754406]. https://doi.org/10.3389/fimmu.2016.00052.
-
2.
Adeva-Andany M, Lopez-Ojen M, Funcasta-Calderon R, Ameneiros-Rodriguez E, Donapetry-Garcia C, Vila-Altesor M, et al. Comprehensive review on lactate metabolism in human health. Mitochondrion. 2014;17:76-100. [PubMed ID: 24929216]. https://doi.org/10.1016/j.mito.2014.05.007.
-
3.
Aduen J, Bernstein WK, Khastgir T, Miller J, Kerzner R, Bhatiani A, et al. The use and clinical importance of a substrate-specific electrode for rapid determination of blood lactate concentrations. JAMA. 1994;272(21):1678-85. [PubMed ID: 7966896].
-
4.
Sheriff DS. Lactic acidosis and small cell carcinoma of the lung. Postgrad Med J. 1986;62(726):297-8. [PubMed ID: 3012499]. [PubMed Central ID: PMC2418670]. https://doi.org/10.1136/pgmj.62.726.297.
-
5.
Philp A, Macdonald AL, Watt PW. Lactate--a signal coordinating cell and systemic function. J Exp Biol. 2005;208(Pt 24):4561-75. [PubMed ID: 16326938]. https://doi.org/10.1242/jeb.01961.
-
6.
De Backer D, Creteur J, Zhang H, Norrenberg M, Vincent JL. Lactate production by the lungs in acute lung injury. Am J Respir Crit Care Med. 1997;156(4 Pt 1):1099-104. [PubMed ID: 9351608]. https://doi.org/10.1164/ajrccm.156.4.9701048.
-
7.
Levy B. Lactate and shock state: the metabolic view. Curr Opin Crit Care. 2006;12(4):315-21. [PubMed ID: 16810041]. https://doi.org/10.1097/01.ccx.0000235208.77450.15.
-
8.
Okorie ON, Dellinger P. Lactate: biomarker and potential therapeutic target. Crit Care Clin. 2011;27(2):299-326. [PubMed ID: 21440203]. https://doi.org/10.1016/j.ccc.2010.12.013.
-
9.
Pinheiro C, Longatto-Filho A, Ferreira L, Pereira SM, Etlinger D, Moreira MA, et al. Increasing expression of monocarboxylate transporters 1 and 4 along progression to invasive cervical carcinoma. Int J Gynecol Pathol. 2008;27(4):568-74. [PubMed ID: 18753962]. https://doi.org/10.1097/PGP.0b013e31817b5b40.
-
10.
Stanley WC. Myocardial lactate metabolism during exercise. Med Sci Sports Exerc. 1991;23(8):920-4. [PubMed ID: 1956265].
-
11.
Brooks GA. Cell-cell and intracellular lactate shuttles. J Physiol. 2009;587(Pt 23):5591-600. [PubMed ID: 19805739]. [PubMed Central ID: PMC2805372]. https://doi.org/10.1113/jphysiol.2009.178350.
-
12.
Goyal MS, Hawrylycz M, Miller JA, Snyder AZ, Raichle ME. Aerobic glycolysis in the human brain is associated with development and neotenous gene expression. Cell Metab. 2014;19(1):49-57. [PubMed ID: 24411938]. [PubMed Central ID: PMC4389678]. https://doi.org/10.1016/j.cmet.2013.11.020.
-
13.
Ide K, Schmalbruch IK, Quistorff B, Horn A, Secher NH. Lactate, glucose and O2 uptake in human brain during recovery from maximal exercise. J Physiol. 2000;522 Pt 1(Pt 1):159-64. [PubMed ID: 10618160]. [PubMed Central ID: PMC2269743]. https://doi.org/10.1111/j.1469-7793.2000.t01-2-00159.xm.
-
14.
Poole RC, Halestrap AP. Transport of lactate and other monocarboxylates across mammalian plasma membranes. Am J Physiol. 1993;264(4 Pt 1):C761-82. [PubMed ID: 8476015]. https://doi.org/10.1152/ajpcell.1993.264.4.C761.
-
15.
Brand A, Singer K, Koehl GE, Kolitzus M, Schoenhammer G, Thiel A, et al. LDHA-Associated Lactic Acid Production Blunts Tumor Immunosurveillance by T and NK Cells. Cell Metab. 2016;24(5):657-71. [PubMed ID: 27641098]. https://doi.org/10.1016/j.cmet.2016.08.011.
-
16.
McCullagh KJ, Poole RC, Halestrap AP, O'Brien M, Bonen A. Role of the lactate transporter (MCT1) in skeletal muscles. Am J Physiol. 1996;271(1 Pt 1):E143-50. [PubMed ID: 8760092]. https://doi.org/10.1152/ajpendo.1996.271.1.E143.
-
17.
Philp NJ, Yoon H, Grollman EF. Monocarboxylate transporter MCT1 is located in the apical membrane and MCT3 in the basal membrane of rat RPE. Am J Physiol. 1998;274(6):R1824-8. [PubMed ID: 9841555]. https://doi.org/10.1152/ajpregu.1998.274.6.R1824.
-
18.
Warburg O. On respiratory impairment in cancer cells. Science. 1956;124(3215):269-70. [PubMed ID: 13351639].
-
19.
Alfarouk KO, Shayoub ME, Muddathir AK, Elhassan GO, Bashir AH. Evolution of Tumor Metabolism might Reflect Carcinogenesis as a Reverse Evolution process (Dismantling of Multicellularity). Cancers (Basel). 2011;3(3):3002-17. [PubMed ID: 24310356]. [PubMed Central ID: PMC3759183]. https://doi.org/10.3390/cancers3033002.
-
20.
Wang C, Youle RJ. The role of mitochondria in apoptosis*. Annu Rev Genet. 2009;43:95-118. [PubMed ID: 19659442]. [PubMed Central ID: PMC4762029]. https://doi.org/10.1146/annurev-genet-102108-134850.
-
21.
Hanahan D, Weinberg RA. Hallmarks of cancer: the next generation. Cell. 2011;144(5):646-74. [PubMed ID: 21376230]. https://doi.org/10.1016/j.cell.2011.02.013.
-
22.
Vander Heiden MG, DeBerardinis RJ. Understanding the Intersections between Metabolism and Cancer Biology. Cell. 2017;168(4):657-69. [PubMed ID: 28187287]. [PubMed Central ID: PMC5329766]. https://doi.org/10.1016/j.cell.2016.12.039.
-
23.
Levine AJ, Puzio-Kuter AM. The control of the metabolic switch in cancers by oncogenes and tumor suppressor genes. Science. 2010;330(6009):1340-4. [PubMed ID: 21127244]. https://doi.org/10.1126/science.1193494.
-
24.
Koppenol WH, Bounds PL, Dang CV. Otto Warburg's contributions to current concepts of cancer metabolism. Nat Rev Cancer. 2011;11(5):325-37. [PubMed ID: 21508971]. https://doi.org/10.1038/nrc3038.
-
25.
Xie H, Hanai J, Ren JG, Kats L, Burgess K, Bhargava P, et al. Targeting lactate dehydrogenase--a inhibits tumorigenesis and tumor progression in mouse models of lung cancer and impacts tumor-initiating cells. Cell Metab. 2014;19(5):795-809. [PubMed ID: 24726384]. [PubMed Central ID: PMC4096909]. https://doi.org/10.1016/j.cmet.2014.03.003.
-
26.
Lee JW, Bae SH, Jeong JW, Kim SH, Kim KW. Hypoxia-inducible factor (HIF-1)alpha: its protein stability and biological functions. Exp Mol Med. 2004;36(1):1-12. [PubMed ID: 15031665]. https://doi.org/10.1038/emm.2004.1.
-
27.
Warburg O. On the origin of cancer cells. Science. 1956;123(3191):309-14. [PubMed ID: 13298683]. https://doi.org/10.1126/science.123.3191.309.
-
28.
Vander Heiden MG, Cantley LC, Thompson CB. Understanding the Warburg effect: the metabolic requirements of cell proliferation. Science. 2009;324(5930):1029-33. [PubMed ID: 19460998]. [PubMed Central ID: PMC2849637]. https://doi.org/10.1126/science.1160809.
-
29.
Sonveaux P, Vegran F, Schroeder T, Wergin MC, Verrax J, Rabbani ZN, et al. Targeting lactate-fueled respiration selectively kills hypoxic tumor cells in mice. J Clin Invest. 2008;118(12):3930-42. [PubMed ID: 19033663]. [PubMed Central ID: PMC2582933]. https://doi.org/10.1172/JCI36843.
-
30.
Vaishnavi SN, Vlassenko AG, Rundle MM, Snyder AZ, Mintun MA, Raichle ME. Regional aerobic glycolysis in the human brain. Proc Natl Acad Sci U S A. 2010;107(41):17757-62. [PubMed ID: 20837536]. [PubMed Central ID: PMC2955101]. https://doi.org/10.1073/pnas.1010459107.
-
31.
Chen YJ, Mahieu NG, Huang X, Singh M, Crawford PA, Johnson SL, et al. Lactate metabolism is associated with mammalian mitochondria. Nat Chem Biol. 2016;12(11):937-43. [PubMed ID: 27618187]. [PubMed Central ID: PMC5069139]. https://doi.org/10.1038/nchembio.2172.
-
32.
Boidot R, Vegran F, Meulle A, Le Breton A, Dessy C, Sonveaux P, et al. Regulation of monocarboxylate transporter MCT1 expression by p53 mediates inward and outward lactate fluxes in tumors. Cancer Res. 2012;72(4):939-48. [PubMed ID: 22184616]. https://doi.org/10.1158/0008-5472.CAN-11-2474.
-
33.
Doherty JR, Cleveland JL. Targeting lactate metabolism for cancer therapeutics. J Clin Invest. 2013;123(9):3685-92. [PubMed ID: 23999443]. [PubMed Central ID: PMC3754272]. https://doi.org/10.1172/JCI69741.
-
34.
Fischer K, Hoffmann P, Voelkl S, Meidenbauer N, Ammer J, Edinger M, et al. Inhibitory effect of tumor cell-derived lactic acid on human T cells. Blood. 2007;109(9):3812-9. [PubMed ID: 17255361]. https://doi.org/10.1182/blood-2006-07-035972.
-
35.
Carpenter L, Halestrap AP. The kinetics, substrate and inhibitor specificity of the lactate transporter of Ehrlich-Lettre tumour cells studied with the intracellular pH indicator BCECF. Biochem J. 1994;304 ( Pt 3)(Pt 3):751-60. [PubMed ID: 7818477]. [PubMed Central ID: PMC1137398]. https://doi.org/10.1042/bj3040751.
-
36.
Halestrap AP, Denton RM. Specific inhibition of pyruvate transport in rat liver mitochondria and human erythrocytes by alpha-cyano-4-hydroxycinnamate. Biochem J. 1974;138(2):313-6. [PubMed ID: 4822737]. [PubMed Central ID: PMC1166210]. https://doi.org/10.1042/bj1380313.
-
37.
Simchowitz L, Textor JA. Lactic acid secretion by human neutrophils. Evidence for an H+ + lactate- cotransport system. J Gen Physiol. 1992;100(2):341-67. [PubMed ID: 1402785]. [PubMed Central ID: PMC2229137]. https://doi.org/10.1085/jgp.100.2.341.
-
38.
Halestrap AP. The monocarboxylate transporter family--Structure and functional characterization. IUBMB Life. 2012;64(1):1-9. [PubMed ID: 22131303]. https://doi.org/10.1002/iub.573.
-
39.
Lee Y, Morrison BM, Li Y, Lengacher S, Farah MH, Hoffman PN, et al. Oligodendroglia metabolically support axons and contribute to neurodegeneration. Nature. 2012;487(7408):443-8. [PubMed ID: 22801498]. [PubMed Central ID: PMC3408792]. https://doi.org/10.1038/nature11314.
-
40.
Fang J, Quinones QJ, Holman TL, Morowitz MJ, Wang Q, Zhao H, et al. The H+-linked monocarboxylate transporter (MCT1/SLC16A1): a potential therapeutic target for high-risk neuroblastoma. Mol Pharmacol. 2006;70(6):2108-15. [PubMed ID: 17000864]. https://doi.org/10.1124/mol.106.026245.
-
41.
Lim KS, Lim KJ, Price AC, Orr BA, Eberhart CG, Bar EE. Inhibition of monocarboxylate transporter-4 depletes stem-like glioblastoma cells and inhibits HIF transcriptional response in a lactate-independent manner. Oncogene. 2014;33(35):4433-41. [PubMed ID: 24077291]. [PubMed Central ID: PMC4087088]. https://doi.org/10.1038/onc.2013.390.
-
42.
Singer K, Kastenberger M, Gottfried E, Hammerschmied CG, Buttner M, Aigner M, et al. Warburg phenotype in renal cell carcinoma: high expression of glucose-transporter 1 (GLUT-1) correlates with low CD8(+) T-cell infiltration in the tumor. Int J Cancer. 2011;128(9):2085-95. [PubMed ID: 20607826]. https://doi.org/10.1002/ijc.25543.
-
43.
Feder-Mengus C, Ghosh S, Weber WP, Wyler S, Zajac P, Terracciano L, et al. Multiple mechanisms underlie defective recognition of melanoma cells cultured in three-dimensional architectures by antigen-specific cytotoxic T lymphocytes. Br J Cancer. 2007;96(7):1072-82. [PubMed ID: 17342088]. [PubMed Central ID: PMC2360115]. https://doi.org/10.1038/sj.bjc.6603664.
-
44.
Holm E, Hagmuller E, Staedt U, Schlickeiser G, Gunther HJ, Leweling H, et al. Substrate balances across colonic carcinomas in humans. Cancer Res. 1995;55(6):1373-8. [PubMed ID: 7882338].
-
45.
Mantovani A. Macrophages, Neutrophils, and Cancer: A Double Edged Sword. New J Sci. 2014;2014:1-14. https://doi.org/10.1155/2014/271940.
-
46.
Kelly B, O'Neill LA. Metabolic reprogramming in macrophages and dendritic cells in innate immunity. Cell Res. 2015;25(7):771-84. [PubMed ID: 26045163]. [PubMed Central ID: PMC4493277]. https://doi.org/10.1038/cr.2015.68.
-
47.
Galvan-Pena S, O'Neill LA. Metabolic reprograming in macrophage polarization. Front Immunol. 2014;5:420. [PubMed ID: 25228902]. [PubMed Central ID: PMC4151090]. https://doi.org/10.3389/fimmu.2014.00420.
-
48.
Sica A, Larghi P, Mancino A, Rubino L, Porta C, Totaro MG, et al. Macrophage polarization in tumour progression. Semin Cancer Biol. 2008;18(5):349-55. [PubMed ID: 18467122]. https://doi.org/10.1016/j.semcancer.2008.03.004.
-
49.
Pearce EJ, Everts B. Dendritic cell metabolism. Nat Rev Immunol. 2015;15(1):18-29. [PubMed ID: 25534620]. [PubMed Central ID: PMC4495583]. https://doi.org/10.1038/nri3771.
-
50.
Jantsch J, Chakravortty D, Turza N, Prechtel AT, Buchholz B, Gerlach RG, et al. Hypoxia and hypoxia-inducible factor-1 alpha modulate lipopolysaccharide-induced dendritic cell activation and function. J Immunol. 2008;180(7):4697-705. [PubMed ID: 18354193]. https://doi.org/10.4049/jimmunol.180.7.4697.
-
51.
Frauwirth KA, Riley JL, Harris MH, Parry RV, Rathmell JC, Plas DR, et al. The CD28 signaling pathway regulates glucose metabolism. Immunity. 2002;16(6):769-77. [PubMed ID: 12121659]. https://doi.org/10.1016/s1074-7613(02)00323-0.
-
52.
Caro-Maldonado A, Wang R, Nichols AG, Kuraoka M, Milasta S, Sun LD, et al. Metabolic reprogramming is required for antibody production that is suppressed in anergic but exaggerated in chronically BAFF-exposed B cells. J Immunol. 2014;192(8):3626-36. [PubMed ID: 24616478]. [PubMed Central ID: PMC3984038]. https://doi.org/10.4049/jimmunol.1302062.
-
53.
Tran Janco JM, Lamichhane P, Karyampudi L, Knutson KL. Tumor-infiltrating dendritic cells in cancer pathogenesis. J Immunol. 2015;194(7):2985-91. [PubMed ID: 25795789]. [PubMed Central ID: PMC4369768]. https://doi.org/10.4049/jimmunol.1403134.
-
54.
Husain Z, Huang Y, Seth P, Sukhatme VP. Tumor-derived lactate modifies antitumor immune response: effect on myeloid-derived suppressor cells and NK cells. J Immunol. 2013;191(3):1486-95. [PubMed ID: 23817426]. https://doi.org/10.4049/jimmunol.1202702.
-
55.
Zou W. Immunosuppressive networks in the tumour environment and their therapeutic relevance. Nat Rev Cancer. 2005;5(4):263-74. [PubMed ID: 15776005]. https://doi.org/10.1038/nrc1586.
-
56.
de Oliveira AT, Pinheiro C, Longatto-Filho A, Brito MJ, Martinho O, Matos D, et al. Co-expression of monocarboxylate transporter 1 (MCT1) and its chaperone (CD147) is associated with low survival in patients with gastrointestinal stromal tumors (GISTs). J Bioenerg Biomembr. 2012;44(1):171-8. [PubMed ID: 22281667]. https://doi.org/10.1007/s10863-012-9408-5.
-
57.
Kato Y, Ozawa S, Tsukuda M, Kubota E, Miyazaki K, St-Pierre Y, et al. Acidic extracellular pH increases calcium influx-triggered phospholipase D activity along with acidic sphingomyelinase activation to induce matrix metalloproteinase-9 expression in mouse metastatic melanoma. FEBS J. 2007;274(12):3171-83. [PubMed ID: 17540003]. https://doi.org/10.1111/j.1742-4658.2007.05848.x.
-
58.
Kato Y, Nakayama Y, Umeda M, Miyazaki K. Induction of 103-kDa gelatinase/type IV collagenase by acidic culture conditions in mouse metastatic melanoma cell lines. J Biol Chem. 1992;267(16):11424-30. [PubMed ID: 1317866].
-
59.
Xu L, Fukumura D, Jain RK. Acidic extracellular pH induces vascular endothelial growth factor (VEGF) in human glioblastoma cells via ERK1/2 MAPK signaling pathway: mechanism of low pH-induced VEGF. J Biol Chem. 2002;277(13):11368-74. [PubMed ID: 11741977]. https://doi.org/10.1074/jbc.M108347200.
-
60.
Shi Q, Abbruzzese JL, Huang S, Fidler IJ, Xiong Q, Xie K. Constitutive and inducible interleukin 8 expression by hypoxia and acidosis renders human pancreatic cancer cells more tumorigenic and metastatic. Clin Cancer Res. 1999;5(11):3711-21. [PubMed ID: 10589791].
-
61.
Xu L, Fidler IJ. Acidic pH-induced elevation in interleukin 8 expression by human ovarian carcinoma cells. Cancer Res. 2000;60(16):4610-6. [PubMed ID: 10969814].
-
62.
Bronte V. Tumor cells hijack macrophages via lactic acid. Immunol Cell Biol. 2014;92(8):647-9. [PubMed ID: 25091608]. https://doi.org/10.1038/icb.2014.67.
-
63.
Romero-Garcia S, Lopez-Gonzalez JS, Baez-Viveros JL, Aguilar-Cazares D, Prado-Garcia H. Tumor cell metabolism: an integral view. Cancer Biol Ther. 2011;12(11):939-48. [PubMed ID: 22057267]. [PubMed Central ID: PMC3280912]. https://doi.org/10.4161/cbt.12.11.18140.
-
64.
Brizel DM, Schroeder T, Scher RL, Walenta S, Clough RW, Dewhirst MW, et al. Elevated tumor lactate concentrations predict for an increased risk of metastases in head-and-neck cancer. Int J Radiat Oncol Biol Phys. 2001;51(2):349-53. [PubMed ID: 11567808]. https://doi.org/10.1016/s0360-3016(01)01630-3.
-
65.
Kharaziha P, Ceder S, Li Q, Panaretakis T. Tumor cell-derived exosomes: a message in a bottle. Biochim Biophys Acta. 2012;1826(1):103-11. [PubMed ID: 22503823]. https://doi.org/10.1016/j.bbcan.2012.03.006.
-
66.
Parolini I, Federici C, Raggi C, Lugini L, Palleschi S, De Milito A, et al. Microenvironmental pH is a key factor for exosome traffic in tumor cells. J Biol Chem. 2009;284(49):34211-22. [PubMed ID: 19801663]. [PubMed Central ID: PMC2797191]. https://doi.org/10.1074/jbc.M109.041152.
-
67.
Gunnerson KJ, Saul M, He S, Kellum JA. Lactate versus non-lactate metabolic acidosis: a retrospective outcome evaluation of critically ill patients. Crit Care. 2006;10(1):R22. [PubMed ID: 16507145]. [PubMed Central ID: PMC1550830]. https://doi.org/10.1186/cc3987.
-
68.
Mariappan R, Venkatraghavan L, Vertanian A, Agnihotri S, Cynthia S, Reyhani S, et al. Serum lactate as a potential biomarker of malignancy in primary adult brain tumours. J Clin Neurosci. 2015;22(1):144-8. [PubMed ID: 25172017]. https://doi.org/10.1016/j.jocn.2014.06.005.
-
69.
Dhup S, Dadhich RK, Porporato PE, Sonveaux P. Multiple biological activities of lactic acid in cancer: influences on tumor growth, angiogenesis and metastasis. Curr Pharm Des. 2012;18(10):1319-30. [PubMed ID: 22360558]. https://doi.org/10.2174/138161212799504902.
-
70.
Colombo GM, Del Vecchio LR, Sacco T, Cicchinelli M. Fatal lactic acidosis due to widespread diffusion of melanoma. Minerva Med. 2006;97(3):295. [PubMed ID: 16855524].
-
71.
Sattler UG, Mueller-Klieser W. The anti-oxidant capacity of tumour glycolysis. Int J Radiat Biol. 2009;85(11):963-71. [PubMed ID: 19895273]. https://doi.org/10.3109/09553000903258889.
-
72.
Morais-Santos F, Granja S, Miranda-Goncalves V, Moreira AH, Queiros S, Vilaca JL, et al. Targeting lactate transport suppresses in vivo breast tumour growth. Oncotarget. 2015;6(22):19177-89. [PubMed ID: 26203664]. [PubMed Central ID: PMC4662483]. https://doi.org/10.18632/oncotarget.3910.
-
73.
Hirschhaeuser F, Sattler UG, Mueller-Klieser W. Lactate: a metabolic key player in cancer. Cancer Res. 2011;71(22):6921-5. [PubMed ID: 22084445]. https://doi.org/10.1158/0008-5472.CAN-11-1457.
-
74.
Sandulache VC, Chen Y, Skinner HD, Lu T, Feng L, Court LE, et al. Acute Tumor Lactate Perturbations as a Biomarker of Genotoxic Stress: Development of a Biochemical Model. Mol Cancer Ther. 2015;14(12):2901-8. [PubMed ID: 26376962]. [PubMed Central ID: PMC4762176]. https://doi.org/10.1158/1535-7163.MCT-15-0217.