Abstract
Background:
Recent data have suggested that nonalcoholic fatty liver disease (NAFLD) can occur in normal-weight subjects. This study examined the association of body fat percentage (BF%) with NAFLD and its risk factors in normal-weight individuals.Objectives:
The present study aimed to explain the association of body fat with NAFLD and its cardiometabolic risk factors.Methods:
A total of 59 subjects with body mass index (BMI) within the range of 18.5 - 24.9 kg/m2 were selected from referrals to two major university polyclinics in Shiraz, Iran, from April to June 2019. Fatty liver grade, anthropometric characteristics, body composition, and cardiometabolic risk factors were measured in this study.Results:
Waist circumference (P = 0.012), fat mass (P < 0.001), triglycerides (TG) (P = 0.027), very-low-density lipoprotein (VLDL) (P = 0.007), and TG/high-density lipoprotein cholesterol (HDL-C) ratio (P = 0.003) increased; however, skeletal muscle mass decreased (P < 0.001) across the tertiles of BF%. The average of fatty liver grade was similar in the first and second tertiles; nevertheless, the fatty liver grade of participants in the third tertile was significantly higher (1.3 ± 0.9 vs. 0.4 ± 0.7; P = 0.005). In ordinal regression analysis, BF% (1.13; 95% CI: 1.04 - 1.22; P = 0.003), BMI (1.95; 95% CI: 1.02 - 3.74; P = 0.045), VLDL (1.77; 95% CI: 1.00 - 3.12; P = 0.049), and TG/HDL-C ratio (2.21; 95% CI: 1.26 - 3.86; P = 0.006) had positive associations with NAFLD; nonetheless, HDL-C (0.33; 95% CI: 0.16 - 0.67; P = 0.002) and dietary cholesterol (0.97; 95% CI: 0.95 - 0.997; P = 0.028) had inverse associations with NAFLD after the adjustments for age, gender, BMI, and physical activity.Conclusions:
The results of this study suggested that within normal weight ranges, NAFLD occurs more frequently in individuals with higher BF%. In addition, BF% can be used as an important marker in NAFLD screening.Keywords
Non-alcoholic Fatty Liver Disease NAFLD Body Composition Body Fat Fatty Liver Grade
1. Background
Nonalcoholic fatty liver disease (NAFLD) is increasingly becoming a public health concern worldwide (1). The global prevalence of NAFLD is 25.2%, with the highest (31.8%) and lowest (13.5%) prevalence in the Middle East and Africa, respectively. The NAFLD is caused by excessive fat accumulation in hepatocytes without an external cause, such as alcohol, hepatotoxic medications, or viral infections (1). The NAFLD is related to metabolic diseases, such as type 2 diabetes and cardiovascular diseases (2). The NAFLD has been accepted as the hepatic manifestation of metabolic syndrome, a bunch of metabolic disorders originating from overweight and obesity (3, 4).
The link between obesity and NAFLD has been well established (5). Obesity contributes to the initiation, progression, and development of NAFLD to more complicated disease conditions, such as nonalcoholic steatohepatitis, cirrhosis, and liver cancer (5). However, recent studies have suggested that NAFLD also develops in lean subjects (6, 7). Even normal-weight subjects may develop the risk factors for NAFLD. The results of a meta-analysis revealed that lean individuals with NAFLD had higher plasma glucose and lipids, insulin resistance, blood pressure, and waist circumference compared to lean subjects without NAFLD (6). A prospective cohort showed that the risk of developing metabolic comorbidities in lean subjects with NAFLD is similar to that of non-lean subjects with NAFLD (7).
Lean subjects may have excess abdominal fat, and this can increase NAFLD risk (6). Excess adipose tissue, particularly in intra-abdominal areas, is supposed to contribute to metabolic comorbidities in NAFLD (8). Fat tissue functions as a source of free fatty acids and adipokines, both of which are involved in insulin resistance which is one of the core components in the initiation of NAFLD (9). Therefore, this study intended to determine the association of body fat percentage (BF%) with NAFLD and its cardiometabolic risk factors in normal-weight individuals. This study examined fatty liver grade, body composition, and cardiometabolic risk factors in a group of subjects with normal body mass index (BMI).
2. Objectives
This study was designed to explain the relationship of body fat and NAFLD and its cardiometabolic risk factors in normal weight individuals.
3. Methods
3.1. Study Design
This cross-sectional study was performed during April to June 2019. The sample size was determined at 53 with the assumption of hypothetical NAFLD in subjects with low and moderate versus high BF% of 20.4% and 63.9%, respectively (10) (a two-sided confidence level of 95%, power of 80%, and case to control ratio of 0.5). The patients were recalled via poster advertisements in university polyclinics and hospitals. Upon recruitment, the study protocol was explained, and written consent was obtained from all the participants. The trial was reviewed and approved by the Ethics Committee of Shiraz University of Medical Sciences, Fars, Iran (approval code: IR.SUMS.REC.1398.314). Moreover, the study was performed according to the guidelines of the 1964 Helsinki Declaration and its later amendments.
3.2. Participants
The participants were selected from referrals to two major university polyclinics in Shiraz, Iran. The inclusion criteria were healthy individuals with normal weight (BMI: 18.5 - 24.9 kg/m2), absence of metabolic diseases (e.g., diabetes, thyroid problems, and cardiovascular diseases), no history of hepatitis and other hepatic and gallbladder disorders, and no history of consuming alcohol or medications affecting the lipid profile, blood pressure, and glucose, or predisposition to fatty liver (e.g., valproic acid and methotrexate).
3.3. Liver Ultrasound
The fatty liver grade was assessed in the fasting state by liver ultrasound (Medison-Accuvix-V10). The degree of echogenicity, visualization of the diaphragm, borders of the liver vasculature, and visualization of the posterior portion of the right hepatic lobe were used to assess the severity of fatty liver (grades 1 to 3).
3.4. Anthropometric and Blood Pressure Measurements
Height was measured using a wall-fixed tape to the nearest 0.1 cm. Bodyweight was measured while participants were in light clothes to the nearest 0.1 kg using a digital scale (GlamorBS-801, Hitachi, China). Body composition indices, including BF%, fat mass, and muscle mass, were determined by a bioelectric impedance analyzer (BIA) (InBody, Biospace Co., South Korea). Basal metabolic rate (BMR) and waist circumference were estimated by the BIA device. With an interval of at least 5 min, blood pressure was measured twice in each visit using a digital monitor (Beurer BM 44, Beurer GmbH, Germany); the mean values were considered the patient final blood pressure. Physical activity was assessed by a validated International Physical Activity Questionnaire (IPAQ). The IPAQ is considered a cost-effective method with good reliability and acceptable validity, designed as a seven-item questionnaire assessing the duration and frequency of vigorous, moderate, and walking activities of the past 7 days. Metabolic equivalent hours per day (MET-h per day) was defined as the unit to represent this measure. To obtain a continuous variable score from the IPAQ (MET minutes a week), walking would be considered to be 3.3 METs, moderate physical activity to be 4 METs, and vigorous physical activity to be 8 METs. (11).
3.5. Biochemical Assessments
After 12-h overnight fasting, the blood samples were collected from all the participants. The serum was separated immediately and stored at -70°C for later analysis. Fasting blood glucose, low-density lipoprotein cholesterol (LDL-C), high-density lipoprotein cholesterol (HDL-C), triglycerides (TG), total cholesterol, alanine transaminase (ALT), and aspartate transaminase were determined using commercial kits (Pars Azmun, Tehran, Iran) and an auto-analyzer (BT1500, Biotecnica Instruments, Italy). Very-low-density lipoprotein (VLDL) was estimated by dividing TG by 5.
3.6. Dietary Intake Assessment
Dietary intake was assessed by a Validated Food Frequency Questionnaire (FFQ) with 160 food and drink items (12). The FFQ contained commonly consumed foods by Iranians with standard serving sizes. The participants were asked to report the consumption of each food item on a daily, weekly, or monthly basis within the past year. Portion sizes were then converted to grams. For dietary analysis, the nutrient composition of the consumed foods was determined by the modified Nutritionist IV software (version 3.5.2).
3.7. Statistical Analysis
Data analysis was performed using SPSS software (version 21; IBM, Armonk, NY, USA). Data normality was checked using the Shapiro-Wilk test. Furthermore, the abnormally distributed data (i.e., all except for blood pressure, total cholesterol, body weight, and body fat) were log-transformed before analysis. The participants were categorized into three groups based on the gender-specific tertiles of BF%. Between the gender-specific tertiles of BF%, parameters were compared using analysis of covariance (ANCOVA) adjusted for age, gender, BMI, and physical activity. Similarly, data comparison between different grades of fatty liver (grade 0 [healthy subjects] and grades 1 - 3 of liver steatosis) was made using ANCOVA and age, gender, BMI, and physical activity as the covariates. Nutrient intake between the tertiles of BF% was compared by one-way analysis of variance. Ordinal regression was used to explore the association between BF% and fatty liver grade. Again, the adjustments were made with the addition of age, gender, BMI, and physical activity in the model. STATA software (version 12.0; StataCorp, USA) was used to carry out ordinal regression analysis. A P-value of less than 0.05 was considered statistically significant.
4. Results
The data of 59 subjects were collected in this study. Demographics, anthropometrics, blood lipids, liver enzymes, and fatty liver grades were compared between the gender-specific tertiles of BF% (Table 1). There was no difference in age, gender, height, weight, and BMI among the tertiles of BF%. However, waist circumference (P = 0.012) and fat mass (P < 0.001) significantly increased; nevertheless, skeletal muscle mass and BMR significantly decreased (P < 0.001) across the tertiles. The TG (P = 0.027), VLDL (P = 0.007), and TG/HDL-C ratio (P = 0.003) also showed a significant increase across the tertiles. Fasting blood glucose, total cholesterol, LDL-C, blood pressure, and liver enzymes did not differ between the tertiles of BF%. The average of the fatty liver grade was similar in the first and second tertiles; nonetheless, the fatty liver grade of participants in the third tertile was significantly higher (1.3 ± 0.9 vs. 0.4 ± 0.7; P = 0.005).
Tertile 1 (N = 20) | Tertile 2 (N = 20) | Tertile 3 (N = 19) | P-Valuec | |
---|---|---|---|---|
Age, y | 34.9 ± 12.0 | 35.0 ± 10.2 | 39.2 ± 13.6 | 0.453 |
Gender | ||||
Male | 3 (15) | 3 (15) | 2 (10.5) | 0.900 |
Female | 17 (85) | 17 (85) | 17 (89.5) | |
Height, cm | 164.0 ± 8.5 | 162.7 ± 6.5 | 161.6 ± 6.8 | 0.601 |
Weight, kg | 58.1 ± 10.2 | 60.0 ± 5.8 | 60.1 ± 5.8 | 0.646 |
BMI, kg/m2 | 21.5 ± 2.4 | 22.7 ± 1.8 | 23.0 ± 1.7 | 0.067 |
Waist circumference, cm | 69.6 ± 4.2 | 76.1 ± 3.9 | 78.3 ± 12.0 | 0.012 |
Skeletal muscle mass, kg | 28.8 ± 6.8 | 26.2 ± 3.5 | 23.0 ± 3.6 | < 0.001 |
Fat mass, kg | 6.9 ± 2.6 | 12.8 ± 1.7 | 18.5 ± 2.9 | < 0.001 |
Basal metabolic rate, kcal | 1478 ± 233 | 1389 ± 123 | 1270 ± 116 | < 0.001 |
Fasting blood glucose, mg/dL | 85.0 ± 9.3 | 83.7 ± 9.1 | 82.8 ± 9.8 | 0.993 |
Triglycerides, mg/dL | 85.4 ± 27.7 | 88.8 ± 31.2 | 122.3 ± 52.7 | 0.027 |
Total cholesterol, mg/dL | 138.8 ± 29.4 | 145.4 ± 20.0 | 149.9 ± 33.8 | 0.469 |
LDL cholesterol, mg/dL | 76.1 ± 25.0 | 89.2 ± 22.2 | 80.9 ± 20.0 | 0.053 |
VLDL, mg/dL | 16.7 ± 4.9 | 17.7 ± 6.3 | 24.7 ± 10.4 | 0.007 |
HDL cholesterol, mg/dL | 56.4 ± 12.8 | 49.4 ± 10.5 | 49.2 ± 15.6 | 0.060 |
Triglycerides/HDL cholesterol | 1.6 ± 0.7 | 1.9 ± 0.9 | 2.7 ± 1.2 | 0.003 |
Systolic BP, mmHg | 115.3 ± 10.7 | 115.4 ± 9.8 | 114.5 ± 13.6 | 0.901 |
Diastolic BP, mmHg | 72.0 ± 7.3 | 76.5 ± 7.7 | 74.6 ± 9.5 | 0.206 |
ALT, IU/L | 16.4 ± 11.0 | 17.4 ± 8.6 | 15.7 ± 6.3 | 0.942 |
AST, IU/L | 14.6 ± 3.1 | 15.2 ± 3.7 | 15.2 ± 4.5 | 0.968 |
The comparison of nutrient intake among BF% revealed that except for saturated fatty acids and cholesterol decreasing across BF% tertiles, no difference was observed in energy, macro-nutrients, and micro-nutrients between BF% tertiles (Table 2). Similar to BF% tertiles, fat mass (P = 0.002), TG (P = 0.027), VLDL (P = 0.018), and TG/HDL-C ratio (P = 0.001) increased across the classifications for fatty liver grades (i.e., grades 0 [healthy], 1, 2, and 3) (Table 3). On the other hand, HDL-C decreased across the categories (P = 0.015). After the adjustments for age, gender, BMI, and physical activity, the difference in TG and VLDL was no longer significant. However, the difference in fat mass, HDL-C, and TG/HDL-C ratio remained significant after controlling the aforementioned covariates. Systolic blood pressure also showed a significant difference, although no clear pattern was observed. The difference in BMI, waist circumference, fasting glucose, total cholesterol, LDL-C, and liver enzymes was not statistically significant.
Tertile 1 (N = 20) | Tertile 2 (N = 20) | Tertile 3 (N = 19) | P-Valuec | |
---|---|---|---|---|
Energy, kcal | 2318 ± 676 | 2375 ± 692 | 2364 ± 709 | 0.963 |
Carbohydrates, g/1000 kcal | 147.2 ± 14.3 | 147.9 ± 17.4 | 154.9 ± 17.5 | 0.277 |
Protein, g/1000 kcal | 33.5 ± 4.0 | 32.5 ± 4.2 | 30.9 ± 3.7 | 0.119 |
Fat, g/1000 kcal | 34.2 ± 6.2 | 34.5 ± 7.9 | 32.5 ± 7.1 | 0.629 |
Saturated fatty acids, g/1000 kcal | 9.5 ± 1.9 | 8.4 ± 1.9 | 7.4 ± 2.0 | 0.006 |
Polyunsaturated fatty acids, g/1000 kcal | 14.2 ± 3.3 | 16.2 ± 6.4 | 15.2 ± 3.3 | 0.389 |
Monounsaturated fatty acids, g/1000 kcal | 11.2 ± 2.4 | 11.1 ± 3.5 | 9.8 ± 2.0 | 0.211 |
Cholesterol, g/1000 kcal | 89.2 ± 28.5 | 83.1 ± 20.9 | 62.3 ± 19.3 | 0.002 |
Fiber, g/1000 kcal | 20.0 ± 3.7 | 22.8 ± 7.9 | 25.3 ± 10.0 | 0.107 |
Simple carbohydrates, g/1000 kcal | 27.2 ± 8.0 | 30.7 ± 10.5 | 32.1 ± 12.6 | 0.335 |
Vitamin A, µg/1000 kcal | 260.4 ± 106.0 | 269.0 ± 134.2 | 260.2 ± 93.1 | 0.249 |
Carotenoids, µg/1000 kcal | 1868 ± 1047 | 2181 ± 1323 | 2291 ± 1129 | 0.184 |
Vitamin E, mg/1000 kcal | 6.0 ± 2.1 | 7.8 ± 5.6 | 6.8 ± 2.5 | 0.326 |
Vitamin C, mg/1000 kcal | 69.3 ± 36.3 | 96.2 ± 57.5 | 86.7 ± 65.9 | 0.295 |
Folate, µg/1000 kcal | 256.8 ± 38.9 | 270.3 ± 55.8 | 268.4 ± 58.0 | 0.670 |
Vitamin B12, µg/1000 kcal | 1.17 ± 0.56 | 1.0 ± 0.36 | 0.84 ± 0.46 | 0.102 |
Cardiometabolic Risk Factors and Liver Enzymes in Different Fatty Liver Grade Classificationsa, b
Healthy (N = 32) | Grade 1 (N = 16) | Grades 2 and 3 (N = 11) | P-Valuec | P-Valued | |
---|---|---|---|---|---|
Age, y | 35.2 ± 12.0 | 34.8 ± 10.1 | 41.7 ± 13.9 | 0.249 | |
Gender | |||||
Male | 4 (12.5) | 2 (12.5) | 2 (18.2) | 0.884 | |
Female | 28 (87.5) | 14 (87.5) | 9 (81.8) | ||
Height, cm | 162.6 ± 6.8 | 163.2 ± 7.4 | 162.9 ± 8.9 | 0.957 | |
Weight, kg | 57.9 ± 6.3 | 60.1 ± 8.2 | 62.8 ± 9.2 | 0.155 | 0.281 |
BMI, kg/m2 | 21.9 ± 2.2 | 22.5 ± 1.9 | 23.6 ± 1.5 | 0.070 | 0.154 |
Waist circumference, cm | 72.9 ± 5.3 | 77.0 ± 6.2 | 76.0 ± 15.4 | 0.234 | 0.427 |
Skeletal muscle mass, kg | 26.3 ± 4.3 | 25.9 ± 5.3 | 25.7 ± 7.9 | 0.948 | 0.194 |
Fat mass, kg | 10.6 ± 4.6 | 13.9 ± 3.9 | 16.7 ± 6.4 | 0.002 | 0.008 |
Body fat, % | 18.3 ± 7.7 | 23.2 ± 6.6 | 27.4 ± 10.7 | 0.005 | 0.009 |
Basal metabolic rate, kcal | 1391 ± 151 | 1371 ± 179 | 1366 ± 282 | 0.901 | 0.125 |
Fasting blood glucose, mg/dL | 83.5 ± 9.6 | 81.6 ± 7.4 | 87.9 ± 10.9 | 0.255 | 0.130 |
Triglycerides, mg/dL | 89.9 ± 30.3 | 93.9 ± 47.5 | 127.3 ± 49.1 | 0.027 | 0.124 |
Total cholesterol, mg/dL | 147.2 ± 31.2 | 139.4 ± 25.4 | 144.6 ± 24.2 | 0.677 | 0.607 |
VLDL, mg/dL | 17.7 ± 5.9 | 18.9 ± 9.4 | 25.6 ± 9.6 | 0.018 | 0.084 |
LDL cholesterol, mg/dL | 86.4 ± 26.3 | 73.1 ± 13.6 | 82.0 ± 20.0 | 0.161 | 0.164 |
HDL cholesterol, mg/dL | 55.6 ± 14.2 | 50.1 ± 10.4 | 42.8 ± 8.9 | 0.015 | 0.009 |
Triglycerides/HDL cholesterol | 1.7 ± 0.6 | 2.1 ± 1.3 | 3.0 ± 1.0 | 0.001 | 0.007 |
Systolic BP, mmHg | 117.7 ± 10.1 | 109.1 ± 9.8 | 116.2 ± 14.1 | 0.038 | 0.040 |
Diastolic BP, mmHg | 75.7 ± 7.5 | 71.3 ± 8.3 | 75.4 ± 9.9 | 0.208 | 0.345 |
ALT, IU/L | 15.4 ± 6.9 | 15.1 ± 6.9 | 21.6 ± 13.8 | 0.095 | 0.099 |
AST, IU/L | 14.5 ± 3.4 | 14.7 ± 4.2 | 16.8 ± 3.8 | 0.187 | 0.522 |
Figure 1 depicts the percentage of subjects with no or different fatty liver grades in the tertiles of BF%. In the first and second BF% tertiles, the percentage of healthy individuals was relatively high; nevertheless, in the third tertile, there was a higher percentage of individuals with fatty liver grades 1 and 2.
Percentage of patients with different grades of fatty liver in tertiles of body fat percentage; P-value assessed by chi-square reported as 0.008.
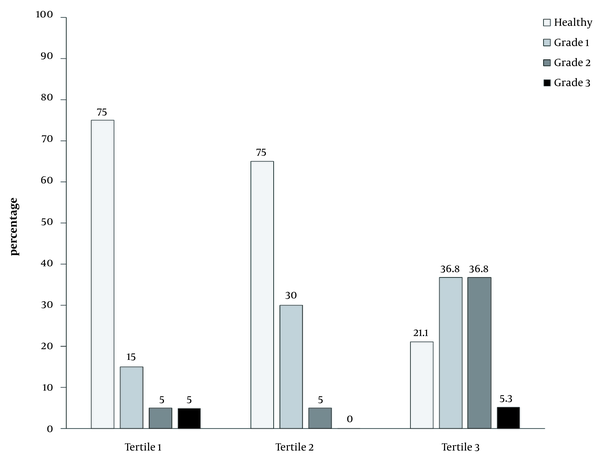
Ordinal regression analysis revealed substantial associations between fatty liver grade and some of the previously examined risk factors. Body fat, BF%, BMI, serum TG, VLDL, and TG/HDL-C ratio had positive associations with NAFLD; nonetheless, serum HDL-C and dietary cholesterol intake had inverse associations (Table 4). After the adjustments for age, gender, BMI, and physical activity, significant associations remained for BF% (1.13; 95% CI: 1.04 - 1.22; P = 0.003), BMI (1.95; 95% CI: 1.02 - 3.74; P = 0.045), VLDL (1.77; 95% CI: 1.00 - 3.12; P = 0.049), HDL-C (0.33; 95% CI: 0.16 - 0.67; P = 0.002), TG/HDL-C ratio (2.21; 95% CI: 1.26 - 3.86; P = 0.006), and dietary cholesterol intake (0.97; 95% CI: 0.95 - 0.997; P = 0.028).
Ordinal Regression Analysis of Associations Between Fatty Liver Grade and Several Cardiometabolic Risk Factorsa
Unadjusted Model | Adjusted Modelb | |||
---|---|---|---|---|
Odds Ratio | P-Value | Odds Ratio | P-Value | |
Body fat percentage | 1.12 (1.05 - 1.21) | 0.002 | 1.13 (1.04 - 1.22) | 0.003 |
Body fat | 1.23 (1.09 - 4.53) | 0.001 | 1.22 (1.07 - 1.40) | 0.003 |
Skeletal muscle mass | 0.91 (0.54 - 1.54) | 0.728 | 0.51 (0.26 - 1.02) | 0.058 |
BMI | 1.85 (1.07 - 3.19) | 0.027 | 1.95 (1.02 - 3.74) | 0.045 |
Waist circumference | 1.76 (0.93 - 3.32) | 0.084 | 1.33 (0.71 - 2.49) | 0.368 |
Fasting blood glucose | 1.23 (0.72 - 2.09) | 0.456 | 1.48 (0.81 - 2.72) | 0.205 |
Triglycerides | 1.70 (1.05 - 2.76) | 0.032 | 1.64 (0.95 - 2.84) | 0.078 |
Total cholesterol | 0.99 (0.97 - 1.01) | 0.302 | 0.98 (0.96 - 1.01) | 0.182 |
VLDL | 1.77 (1.09 - 2.88) | 0.021 | 1.77 (1.00 - 3.12) | 0.049 |
LDL cholesterol | 0.64 (0.36 - 1.13) | 0.125 | 0.56 (0.29 - 1.08) | 0.082 |
HDL cholesterol | 0.42 (0.23 - 0.78) | 0.006 | 0.33 (0.16 - 0.67) | 0.002 |
Triglycerides/HDL cholesterol | 2.31 (1.36 - 3.91) | 0.002 | 2.21 (1.26 - 3.86) | 0.006 |
Systolic blood pressure | 0.97 (0.92 - 1.01) | 0.164 | 0.95 (0.90 - 1.00) | 0.054 |
Diastolic blood pressure | 0.97 (0.92 - 1.03) | 0.391 | 0.98 (0.92 - 1.04) | 0.490 |
ALT | 1.44 (0.91 - 2.30) | 0.121 | 1.55 (0.94 - 2.55) | 0.089 |
AST | 1.54 (0.92 - 2.59) | 0.101 | 1.35 (0.79 - 2.32) | 0.271 |
5. Discussion
The results of this study showed that although no significant difference was observed in the BMI of the participants between BF% tertiles, TG and VLDL increased; however, skeletal muscle mass and BMR decreased across the BF% tertiles. Fatty liver grade had a positive association with BMI, body fat content and percentage, VLDL, and TG/HDL-C ratio, and an inverse association with HDL-C and dietary cholesterol.
5.1. BMI and Body Fat
Although the participants had normal BMI, there was a significant association between BMI and fatty liver grade. Body fat also indicated such an association with fatty liver grade. The results of regression analysis confirmed that BMI had a stronger association with fatty liver grade than body fat (1.95 vs. 1.22). However, BMI and body fat have a synergistic association with fatty liver (13). In other words, each of BMI and body fat correlates with NAFLD; nevertheless, their associations with NAFLD become stronger if they occur concurrently.
Although BMI, waist circumference, and whole and visceral fat correlate with the incidence of fatty liver (10), visceral fat is considered the primary determinant of hepatic fat accumulation (14, 15). According to the results of a cross-sectional study, overweight subjects with central adiposity are at increased risk of NAFLD, compared to BMI-matched subjects with less central adiposity (16). Due to the vicinity of intra-abdominal adipose tissue to the liver, free fatty acids released from these areas are thought to have a critical role in fat deposition in hepatocytes (17). In contrast to visceral fat, subcutaneous fat has been associated with the regression of NAFLD (15). The BIA device does not specify fat masses accumulated in visceral or subcutaneous areas; nonetheless, the current study reported waist circumference as an indicator of visceral fat. There was no association between waist circumference and fatty liver grade, although there was a trend for a positive association in the unadjusted model of regression analysis.
5.2. Skeletal Muscle Mass
In this study, there was a reduction in skeletal muscle mass across BF% tertiles, indicating that the increase of fat mass accompanied muscle loss. However, the association between skeletal muscle loss and NAFLD was not significant, although there was a trend toward the presence of an inverse association; nevertheless, some previous studies have reported such a relationship. For instance, a cross-sectional study conducted by Shida et al. (18) reported a close link between decreased muscle mass coupled with increased visceral fat and NAFLD risk. Accordingly, Mizuno et al. (19) reported a decline of skeletal muscle from the early stages of NAFLD and an increase in skeletal muscle to fat mass ratio predictive of ALT reduction in patients with NAFLD. In the current study, there was also no association between liver transaminases and body composition measurements (the data not presented). The lack of an association between muscle mass and either NAFLD or transaminases in the present study might be due to the inclusion criteria for recruiting the participants as they were healthy with normal transaminases; however, in the aforementioned studies, subjects were NAFLD patients with relatively high levels of transaminases.
5.3. VLDL-C and HDL-C
The results of this study showed that VLDL and, to a larger extent, TG/HDL-C ratio had associations with NAFLD; nevertheless, HDL-C had an inverse association. The NAFLD is associated with large and triglyceride-over enriched VLDL particles (20). Poulsen et al. (21) using a tracer technique showed that that the kinetics of VLDL is changed in NAFLD as patients with NAFLD cannot suppress VLDL secretion to compensate for the increased hepatic fat content. However, the increase in hepatic VLDL-TG export is inadequate to normalize intrahepatic TG content (22). The results of previous studies are in line with the findings of the current study. For instance, extensive cross-sectional studies have observed an association between TG/HDL-C and NAFLD (23, 24). Similarly, a cohort study suggested that TG/HDL-C may predict the incidence of NAFLD (25).
The alteration of VLDL/TG and HDL-C occur in opposite directions. The overproduction of VLDL is the core event in lipoprotein changes during metabolic syndrome and NAFLD (26). Circulating cholesteryl ester transfer protein (CETP) exchanges TG of VLDL with cholesteryl ester of HDL particles, thereby decreasing HDL-C concentration (27). In this regard, patients with NAFLD have shown higher CETP activity, compared to individuals without NAFLD (20).
5.4. Strengths and Limitations
This has been the first study examining the association between NAFLD and body fat in normal-weight individuals. Additionally, an adjusted statistical analysis was performed to control the confounding variables. The small sample size was a drawback of the study, causing abnormality in data distribution. Accordingly, the log transformation was performed to correct this abnormality. Furthermore, ultrasound is not the gold standard technique for the diagnosis of NAFLD, and the results need to be checked with more reliable methods, such as magnetic resonance imaging. This method was used due to budget constraints. Moreover, body composition results should be confirmed by more accurate techniques, such as dual X-ray absorptiometry, because the measurements of BIA can be affected by factors such as hydration status, environment temperature, and exercise.
5.5. Conclusions
The results of this study suggested that within normal weight ranges, NAFLD occurs more frequently in individuals with higher BMI and BF%. There was also a positive association between NAFLD with VLDL and TG/HDL-C ratio and a negative association between NAFLD and HDL-C concentration. It is required to perform further studies to confirm these results with more accurate techniques for the diagnosis of NAFLD and assessment of body composition.
Acknowledgements
References
-
1.
Younossi ZM, Koenig AB, Abdelatif D, Fazel Y, Henry L, Wymer M. Global epidemiology of nonalcoholic fatty liver disease-Meta-analytic assessment of prevalence, incidence, and outcomes. Hepatology. 2016;64(1):73-84. [PubMed ID: 26707365]. https://doi.org/10.1002/hep.28431.
-
2.
Rosato V, Masarone M, Dallio M, Federico A, Aglitti A, Persico M. NAFLD and Extra-Hepatic Comorbidities: Current Evidence on a Multi-Organ Metabolic Syndrome. Int J Environ Res Public Health. 2019;16(18). [PubMed ID: 31540048]. [PubMed Central ID: PMC6765902]. https://doi.org/10.3390/ijerph16183415.
-
3.
Sookoian S, Pirola CJ. Review article: shared disease mechanisms between non-alcoholic fatty liver disease and metabolic syndrome - translating knowledge from systems biology to the bedside. Aliment Pharmacol Ther. 2019;49(5):516-27. [PubMed ID: 30714632]. https://doi.org/10.1111/apt.15163.
-
4.
Dietrich P, Hellerbrand C. Non-alcoholic fatty liver disease, obesity and the metabolic syndrome. Best Pract Res Clin Gastroenterol. 2014;28(4):637-53. [PubMed ID: 25194181]. https://doi.org/10.1016/j.bpg.2014.07.008.
-
5.
Polyzos SA, Kountouras J, Mantzoros CS. Adipose tissue, obesity and non-alcoholic fatty liver disease. Minerva Endocrinol. 2017;42(2):92-108. [PubMed ID: 27711029]. https://doi.org/10.23736/S0391-1977.16.02563-3.
-
6.
Sookoian S, Pirola CJ. Systematic review with meta-analysis: risk factors for non-alcoholic fatty liver disease suggest a shared altered metabolic and cardiovascular profile between lean and obese patients. Aliment Pharmacol Ther. 2017;46(2):85-95. [PubMed ID: 28464369]. https://doi.org/10.1111/apt.14112.
-
7.
Niriella MA, Kasturiratne A, Pathmeswaran A, De Silva ST, Perera KR, Subasinghe S, et al. Lean non-alcoholic fatty liver disease (lean NAFLD): characteristics, metabolic outcomes and risk factors from a 7-year prospective, community cohort study from Sri Lanka. Hepatol Int. 2019;13(3):314-22. [PubMed ID: 30539516]. https://doi.org/10.1007/s12072-018-9916-4.
-
8.
Cordeiro A, Costa R, Andrade N, Silva C, Canabrava N, Pena MJ, et al. Does adipose tissue inflammation drive the development of non-alcoholic fatty liver disease in obesity? Clin Res Hepatol Gastroenterol. 2020;44(4):394-402. [PubMed ID: 32044284]. https://doi.org/10.1016/j.clinre.2019.10.001.
-
9.
Akhlaghi M. Non-alcoholic Fatty Liver Disease: Beneficial Effects of Flavonoids. Phytother Res. 2016;30(10):1559-71. [PubMed ID: 27307131]. https://doi.org/10.1002/ptr.5667.
-
10.
Ko YH, Wong TC, Hsu YY, Kuo KL, Yang SH. The Correlation Between Body Fat, Visceral Fat, and Nonalcoholic Fatty Liver Disease. Metab Syndr Relat Disord. 2017;15(6):304-11. [PubMed ID: 28481662]. https://doi.org/10.1089/met.2017.0001.
-
11.
Craig CL, Marshall AL, Sjostrom M, Bauman AE, Booth ML, Ainsworth BE, et al. International physical activity questionnaire: 12-country reliability and validity. Med Sci Sports Exerc. 2003;35(8):1381-95. [PubMed ID: 12900694]. https://doi.org/10.1249/01.MSS.0000078924.61453.FB.
-
12.
Esfahani FH, Asghari G, Mirmiran P, Azizi F. Reproducibility and relative validity of food group intake in a food frequency questionnaire developed for the Tehran Lipid and Glucose Study. J Epidemiol. 2010;20(2):150-8. [PubMed ID: 20154450]. [PubMed Central ID: PMC3900814]. https://doi.org/10.2188/jea.je20090083.
-
13.
Saida T, Fukushima W, Ohfuji S, Kondo K, Matsunaga I, Hirota Y. Effect modification of body mass index and body fat percentage on fatty liver disease in a Japanese population. J Gastroenterol Hepatol. 2014;29(1):128-36. [PubMed ID: 23980616]. https://doi.org/10.1111/jgh.12377.
-
14.
Umano GR, Shabanova V, Pierpont B, Mata M, Nouws J, Trico D, et al. A low visceral fat proportion, independent of total body fat mass, protects obese adolescent girls against fatty liver and glucose dysregulation: a longitudinal study. Int J Obes (Lond). 2019;43(4):673-82. [PubMed ID: 30337653]. https://doi.org/10.1038/s41366-018-0227-6.
-
15.
Kim D, Chung GE, Kwak MS, Seo HB, Kang JH, Kim W, et al. Body Fat Distribution and Risk of Incident and Regressed Nonalcoholic Fatty Liver Disease. Clin Gastroenterol Hepatol. 2016;14(1):132-8 e4. [PubMed ID: 26226099]. https://doi.org/10.1016/j.cgh.2015.07.024.
-
16.
Park SH, Kim BI, Kim SH, Kim HJ, Park DI, Cho YK, et al. Body fat distribution and insulin resistance: beyond obesity in nonalcoholic fatty liver disease among overweight men. J Am Coll Nutr. 2007;26(4):321-6. [PubMed ID: 17906183]. https://doi.org/10.1080/07315724.2007.10719618.
-
17.
Yu AH, Duan-Mu YY, Zhang Y, Wang L, Guo Z, Yu YQ, et al. Correlation between Non-Alcoholic Fatty Liver Disease and Visceral Adipose Tissue in Non-Obese Chinese Adults: A CT Evaluation. Korean J Radiol. 2018;19(5):923-9. [PubMed ID: 30174482]. [PubMed Central ID: PMC6082759]. https://doi.org/10.3348/kjr.2018.19.5.923.
-
18.
Shida T, Akiyama K, Oh S, Sawai A, Isobe T, Okamoto Y, et al. Skeletal muscle mass to visceral fat area ratio is an important determinant affecting hepatic conditions of non-alcoholic fatty liver disease. J Gastroenterol. 2018;53(4):535-47. [PubMed ID: 28791501]. https://doi.org/10.1007/s00535-017-1377-3.
-
19.
Mizuno N, Seko Y, Kataoka S, Okuda K, Furuta M, Takemura M, et al. Increase in the skeletal muscle mass to body fat mass ratio predicts the decline in transaminase in patients with nonalcoholic fatty liver disease. J Gastroenterol. 2019;54(2):160-70. [PubMed ID: 29948305]. https://doi.org/10.1007/s00535-018-1485-8.
-
20.
Lucero D, Miksztowicz V, Gualano G, Longo C, Landeira G, Alvarez E, et al. Nonalcoholic fatty liver disease associated with metabolic syndrome: Influence of liver fibrosis stages on characteristics of very low-density lipoproteins. Clin Chim Acta. 2017;473:1-8. [PubMed ID: 28802640]. https://doi.org/10.1016/j.cca.2017.08.006.
-
21.
Poulsen MK, Nellemann B, Stodkilde-Jorgensen H, Pedersen SB, Gronbaek H, Nielsen S. Impaired Insulin Suppression of VLDL-Triglyceride Kinetics in Nonalcoholic Fatty Liver Disease. J Clin Endocrinol Metab. 2016;101(4):1637-46. [PubMed ID: 26829441]. https://doi.org/10.1210/jc.2015-3476.
-
22.
Fabbrini E, Mohammed BS, Magkos F, Korenblat KM, Patterson BW, Klein S. Alterations in adipose tissue and hepatic lipid kinetics in obese men and women with nonalcoholic fatty liver disease. Gastroenterology. 2008;134(2):424-31. [PubMed ID: 18242210]. [PubMed Central ID: PMC2705923]. https://doi.org/10.1053/j.gastro.2007.11.038.
-
23.
Fan N, Peng L, Xia Z, Zhang L, Song Z, Wang Y, et al. Triglycerides to high-density lipoprotein cholesterol ratio as a surrogate for nonalcoholic fatty liver disease: a cross-sectional study. Lipids Health Dis. 2019;18(1):39. [PubMed ID: 30711017]. [PubMed Central ID: PMC6359827]. https://doi.org/10.1186/s12944-019-0986-7.
-
24.
Wu KT, Kuo PL, Su SB, Chen YY, Yeh ML, Huang CI, et al. Nonalcoholic fatty liver disease severity is associated with the ratios of total cholesterol and triglycerides to high-density lipoprotein cholesterol. J Clin Lipidol. 2016;10(2):420-5 e1. [PubMed ID: 27055973]. https://doi.org/10.1016/j.jacl.2015.12.026.
-
25.
Fukuda Y, Hashimoto Y, Hamaguchi M, Fukuda T, Nakamura N, Ohbora A, et al. Triglycerides to high-density lipoprotein cholesterol ratio is an independent predictor of incident fatty liver; a population-based cohort study. Liver Int. 2016;36(5):713-20. https://doi.org/10.1111/liv.12977.
-
26.
Adiels M, Olofsson SO, Taskinen MR, Boren J. Overproduction of very low-density lipoproteins is the hallmark of the dyslipidemia in the metabolic syndrome. Arterioscler Thromb Vasc Biol. 2008;28(7):1225-36. [PubMed ID: 18565848]. https://doi.org/10.1161/ATVBAHA.107.160192.
-
27.
Girona J, Amigo N, Ibarretxe D, Plana N, Rodriguez-Borjabad C, Heras M, et al. HDL Triglycerides: A New Marker of Metabolic and Cardiovascular Risk. Int J Mol Sci. 2019;20(13). [PubMed ID: 31252694]. [PubMed Central ID: PMC6651243]. https://doi.org/10.3390/ijms20133151.