Abstract
Background:
Chronic bacterial diarrheas are common intestinal infections that have been treated with antibiotics for many years. However, the excessive use of antibiotics has led to the development of resistance in the bacteria that cause these infections. As a result, there has been growing interest in using probiotics with antibacterial effects and a greater ability to survive stomach acidity to help control antibiotic-resistant bacteria.Objectives:
In the current study, the antimicrobial activity of Bacillus coagulans (a probiotic bacterium) against four different enteric pathogens (Escherichia coli, Salmonella typhi, Shigella flexneri, and Bacillus cereus), which play a significant role in the prevalence of bacterial diarrhea, has been investigated.Methods:
The antimicrobial effect of the cell-free supernatant of Bacillus coagulans against pathogens (E. coli, S. typhi, Sh. flexneri, and B. cereus) was studied using agar well-diffusion, agar disc-diffusion, as well as minimum inhibitory concentration (MIC) and minimum bactericidal concentration (MBC) methods. In the MIC test, the concentrations of B. coagulans cell-free supernatant examined were 100, 50, 25, 12.5, 6.25, and 3.1 µg per mL. Each bacterial strain was tested in four replicates.Results:
The antimicrobial activity of B. coagulans supernatant was not observed in either the agar well-diffusion or agar disc-diffusion results. However, MIC results showed that different concentrations of B. coagulans supernatant significantly inhibit the growth of E. coli (MIC = 25 µg/mL, P < 0.0001), S. typhi (MIC = 50 μg/mL, P < 0.0001), S. flexneri (MIC = 3.1 μg/mL, P < 0.0001), and B. cereus (MIC = 100 µg/mL, P < 0.0001).Conclusions:
The MBC results showed that the minimum inhibitory concentration of the supernatant had no bactericidal effects and did not completely prevent the growth of all four pathogens. Although the MIC results indicated the antibacterial activity of B. coagulans against all four pathogens, further studies are required to draw more accurate conclusions.Keywords
Bacillus coagulans Probiotic Antimicrobial Activity Human Enteric Pathogens
1. Background
The gastrointestinal (GI) microbiome is a complex of microbes - naturally inhabit in the human GI tract- that its alteration is in association with immunologic disequilibrium and consequently GI diseases (1, 2). The pivotal role of probiotics to maintain the immunologic equilibrium in the GI tract has been revealed and probiotic effectiveness for functional GI disorders has been reported (2). Probiotics are live microorganisms, available in forms of food and dietary supplements that their administration in adequate amounts is beneficial for the host (3).
To obtain probiotics with high safety and more functionality, the microorganisms should be carefully monitored; and resistance to pancreatic enzymes, stomach acidity, bile, lysozyme, and antibiotics are the most important factors to select beneficial probiotics (3). Lactobacilli, lactic acid bacteria, as well as Bifidobacterium spp, are the most prevalent probiotics which are normally found in the GI tract (4). But there is a growing interest in probiotics with spore-formation characteristics which can survive in adverse environmental conditions (3, 5, 6).
Some spore-forming bacteria, such as Clostridium spp. and Bacillus spp., that are able to encapsulate, can be found not only in GI tract, but in water, soil, and dust as well (7), making these spore-forming probiotics more accessible rather than lactic acid bacteria. Moreover, spores of these probiotics have more opportunity to survive against stomach acidity and consequently to proliferate and colonize in the GI tract (8, 9). Peptides and lipopeptide antibiotics, as well as bacteriocins and non-modified bacteriocins are antimicrobial substances which produce by Bacillus spp.; these antimicrobials have broad-spectrum potential against human and animal pathogens (10). Besides, improved nutritional values and growth, boosted immunity levels, and prevented GI tract diseases - such as inflammatory bowel disease, irritable bowel syndrome and diarrhea - are other advantages of Bacillus spp. (11); leading to considerable success of these probiotics to control of pathogens especially drug-resistant strains, during the past several years (12, 13).
Among the Bacillus spp., Bacillus coagulans (B. coagulans) - gram-positive, spore-forming, aerobic or facultatively anaerobic, lactic acid-producing bacterium - is regarded as non-pathogenic and safe for human consumption (3). This economically important species is becoming increasingly remarkable in the field of probiotics to decrease harmful effects of processing and environment conditions on the survival of bacterial cells and to assure their functionality in the human body. To date, a number of B. coagulans strains are introduced to the food industry as probiotics (14) and some reports have revealed the significant roles of these probiotics as alternatives to Lactobacillus spp for use by human and animal (15, 16).
2. Objectives
Identification of probiotic activity alongside the antibacterial characteristics of B. coagulans strains is crucial for selecting the most effective and highest-yielding microorganisms (3). Therefore, the aim of the current study was to explore the antibacterial activity and efficacy of B. coagulans against Escherichia coli (E. coli), Salmonella typhi (S. typhi), and Sh. flexneri—all gram-negative bacteria—as well as Bacillus cereus (B. cereus), a gram-positive bacterium. These enteric pathogens play a significant role in the prevalence of bacterial diarrhea in humans.
3. Methods
3.1. Materials
The lyophilized B. coagulans (ATCC G8 S4) as the probiotic strain, and E. coli (ATCC 29255), S. typhi (ATCC 14029), Sh. flexneri (ATCC 12022), and (ATCC 11778) as the test strains, were purchased from the Industrial Microorganisms Collection Center (Iran). All media components, including tryptic soy broth (TSB), eosin methylene blue (EMB), Mueller-Hinton broth (MHB), Mueller-Hinton agar (MHA), and nutrient agar (NA), were purchased from Merck (Germany). All chemicals, including tryptone, soy, dipotassium hydrogen phosphate (K2HPO4), dextrose, peptone, lactose, eosin Y, methylene blue, agar, beef extract, yeast, sodium chloride (NaCl), acid hydrolysate of casein, meat infusion, soluble starch, and antibiotic assay discs, were purchased from Sigma Aldrich (USA).
3.2. Bacterial Preparation
Initially, all lyophilized bacterial strains were separately cultured in TSB medium, which contained tryptone (17 g/L), soy (3 g/L), NaCl (5 g/L), K2HPO4 (2.5 g/L), and dextrose (2.5 g/L), and incubated at 37°C for 18 - 24 hours. The cell suspension of each bacterium was then transferred onto agar plates and re-incubated at 37°C for 18 - 24 hours. Following this, colonies of B. coagulans and B. cereus were separately cultivated on NA medium, which contained peptone (5 g/L), beef extract (1 g/L), yeast (2 g/L), sodium chloride (5 g/L), and agar (15 g/L). E. coli was cultivated on EMB medium, which contained peptone (10 g/L), lactose (10 g/L), K2HPO4 (2 g/L), eosin Y (0.4 g/L), methylene blue (0.065 g/L), and agar (15 g/L). Both EMB and NA media were used for the cultivation of S. typhi and Sh. flexneri isolates. The morphological characteristics of the bacteria, such as shape, size, and color, as well as their motility and purity, were assessed under a light microscope. Finally, TSB medium supplemented with 30% glycerol was used for storing all pure strains at −20°C until further study.
3.3. Preparation of Cell Free Supernatant from Bacillus coagulans Cultivate
To obtain the cell-free supernatant from B. coagulans, the frozen stock culture of this bacterium was placed at room temperature for 5 minutes and then stirred in a vortex to homogenize the culture. One milliliter of the culture was then inoculated into 5 mL of TSB medium, homogenized, and incubated at 37°C for 18 - 24 hours. After incubation, 1 mL of the overnight culture was centrifuged at 8,000 g for 7 minutes. The precipitate was discarded, and the cell-free supernatant was sterilized using a 0.45-µm filter. The antimicrobial effects of this sterile culture supernatant were studied in subsequent steps.
3.4. Evaluation of Antimicrobial Efficiency of Cell Free Supernatant Obtained from Bacillus coagulans Cultivate
Fresh EMB and NA culture media (as described earlier) were used to prepare the bacterial suspensions from the frozen stock cultures of E. coli, S. typhi, Sh. flexneri, and B. cereus. The turbidity of the bacterial suspensions was compared to a 0.5 McFarland standard (17). The antimicrobial efficiency of the cell-free supernatant from the probiotic isolate (B. coagulans) against the selected pathogenic bacteria (E. coli, S. typhi, Sh. flexneri, and B. cereus) was evaluated using both agar well-diffusion and agar disc-diffusion methods as follows:
- Agar well-diffusion method: This method was performed using MHA medium, which contained beef extract (2 g/L), casein hydrolysate (17.5 g/L), starch (1.5 g/L), and agar (17 g/L). A suspension of the pathogenic bacteria (0.5 McFarland standard) was used to lawn the MHA plates with sterilized swabs. Two uniform wells, each with a 6 mm diameter, were made on the MHA plates, and the bottom of each well was sealed with 20 µL of sterile molten agar. The first well was filled with 150 µL of TSB medium as a control, while the second well was filled with 150 µL of the cell-free probiotic supernatant as the test sample. The plates were then incubated at 37°C for 18 - 24 hours.
- Agar disc-diffusion method: In this method, a suspension of the pathogenic bacteria was used to lawn the MHA plates. For each plate, two blank discs with a diameter of 6 mm were impregnated with 150 µL of the cell-free probiotic supernatant and placed on the lawned MHA as test discs. Additionally, one disc impregnated with TSB was used as a negative control, and two antibiotic discs served as positive controls. Ciprofloxacin and sulfamethoxazole antibiotic discs were used as positive controls for gram-negative bacteria (E. coli, S. typhi, Sh. flexneri), while ciprofloxacin and ceftriaxone discs were used for the gram-positive bacterium (B. cereus). The plates were then incubated at 37°C for 18 - 24 hours.
3.5. Determination of Minimum Inhibitory Concentration and Minimum Bactericidal Concentration of Bacillus coagulans Cell Free Supernatant on Pathogenic Bacteria
As a previous step, bacterial suspensions were prepared from the frozen stock cultures of E. coli, S. typhi, Sh. flexneri, and B. cereus. Each bacterial suspension was then inoculated into MHB (containing meat infusion (17.5 g/L), acid hydrolysate of casein (17.5 g/L), and starch (1.5 g/L)), and the turbidity was compared to the 0.5 McFarland standard.
- Broth microdilution method: The minimum inhibitory concentration (MIC) of the B. coagulans cell-free supernatant was determined using the broth microdilution method in accordance with the CLSI standard protocol (18) on 96-well plates. For each bacterial strain, the first well was filled with 100 μL of bacterial suspension and 100 μL of B. coagulans cell-free supernatant to test the effect of the pure supernatant without MHB culture medium. Wells 2 - 6 were filled with 100 μL of MHB, and after the addition of 100 μL of supernatant to well 2, serial dilutions (50, 25, 12.5, 6.25, and 3.1 µg/mL) were made across wells 2 - 6. Then, 100 μL of bacterial suspension (containing 105 cells/μL in MHB) was added to each well. Well 7, containing 100 μL of bacterial suspension and 100 μL of MHB, served as the positive control, while well 8, containing 100 μL of cell-free supernatant and 100 μL of MHB, served as the negative control. The well plates were incubated at 37°C for 18 - 24 hours, and bacterial growth was assessed by measuring the optical density at 600 nm using a spectrophotometer. The experiment for each bacterial strain was performed in four replicates, and the lowest concentration at which there was no color change was considered the MIC.
- Minimum bactericidal concentration (MBC): The MBC value was determined by sub-culturing 10 µL from wells with no visible turbidity onto freshly prepared nutrient agar media, followed by incubation at 37°C for 18 - 24 hours. The MBC was defined as the lowest concentration of cell-free supernatant at which no bacterial growth was observed after 24 hours of incubation.
3.6. Statistical Analysis
All measurements in the current study were carried out four times, and the obtained data represent the average of the four replications. GraphPad Prism 8 was used for statistical analysis and to create the charts. Data were analyzed using a one-way analysis of variance (ANOVA) followed by the Tukey post hoc test, with significance levels denoted as * P < 0.05, ** P < 0.01, *** P < 0.001, and **** P < 0.0001.
4. Results
4.1. Growth and Morphological Characteristics of Bacteria Colonies
All pathogenic strains (E. coli, S. typhi, Sh. flexneri, and B. cereus), as well as the probiotic strain (B. coagulans), exhibited normal colony morphology with appropriate growth and were free of contamination.
4.2. Antimicrobial Effects of Bacillus coagulans on Pathogenic Bacteria
The agar well-diffusion test showed that the probiotic supernatant did not create any zone of inhibition around the wells for any of the three gram-negative pathogens (E. coli, S. typhi, and Sh. flexneri) after 18 - 24 hours of incubation, indicating the growth of these strains on the culture medium. Thus, no antibacterial effects of the probiotic were observed against these pathogens. Similarly, no zone of inhibition was observed around the gram-positive B. cereus pathogen. The viability of the pathogens was also confirmed by the growth of bacteria around the control well in each test. The results of the agar well-diffusion test are presented in Figure 1A-D.
Agar well-diffusion test results of probiotic isolate Bacillus coagulans against; A, Escherichia coli; B, Salmonella typhi; C, Shigella flexneri; and D, B.cereus. In each plate, the numbers 1 and 2 show control and test, respectively. It is evident that there is no discernible antibacterial impact of B. coagulans noted on the pathogens.
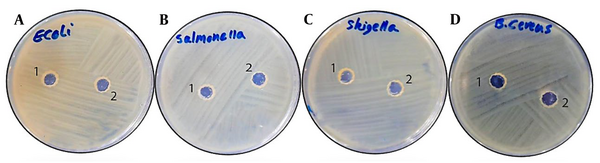
The agar disk-diffusion test for the antibacterial effects of B. coagulans against the three gram-negative pathogens (E. coli, S. typhi, and Sh. flexneri) also showed no zone of inhibition around the disks containing the supernatant, demonstrating the growth of all three bacteria in the presence of the B. coagulans supernatant. Additionally, no zone of inhibition was observed around the negative control disk containing the TSB culture medium, further confirming the viability of the bacteria. In contrast, a zone of inhibition (25 - 28 mm in diameter) was observed around the disks containing ciprofloxacin and sulfamethoxazole (positive controls), indicating the inhibition of bacterial growth by these antibiotics.
Similarly, the growth of the gram-positive B. cereus pathogen was not inhibited in the presence of the supernatant, and no zone of inhibition was observed around the disk containing the B. coagulans supernatant. The disk impregnated with TSB (negative control) also confirmed the viability of the bacteria by not creating a zone of inhibition. In contrast, antibiotic disks containing ceftriaxone and ciprofloxacin (positive controls) caused the formation of zones of inhibition (24 - 26 mm in diameter), preventing bacterial growth. The results of the agar disk-diffusion test are presented in Figure 2A-D.
Disc-diffusion test results of a probiotic isolate Bacillus coagulans (B. coagulans) against A, Escherichia coli; B, Salmonella typhi; C, Shigella flexneri; and D, Bacillus cereus. The tests on each plate display numbers 1 and 2, representing discs impregnated with the cell-free probiotic’s supernatant, while number 3 represents the negative control (disc impregnated with tryptic soy broth (TSB), and AB indicates positive controls (including two antibiotic discs). It is clear that there is no observable antibacterial effect of B. coagulans on the pathogens.
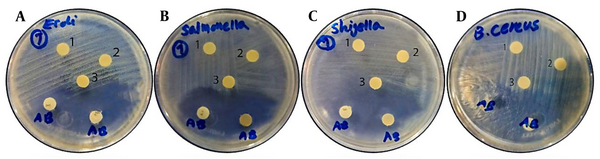
4.3. Minimum Inhibitory and Bactericidal Concentrations of Bacillus coagulans Against Pathogenic Bacteria
The MIC results demonstrated that different concentrations of the probiotic’s supernatant significantly inhibited the growth of E. coli at 100, 50, and 25 µg/mL (P < 0.0001), 12.5 µg/mL (P = 0.0003), and 6.2 µg/mL (P = 0.04). The 25 µg/mL concentration had the highest inhibitory effect on the growth of E. coli. The growth of S. typhi was also significantly affected by the probiotic’s supernatant at concentrations of 100 µg/mL (P < 0.0001), 50 µg/mL (P < 0.0001), and 25 µg/mL (P = 0.015), with the MIC determined to be 50 µg/mL.
The minimum inhibitory concentration of the probiotic’s supernatant against Sh. flexneri was observed at 3.1 µg/mL. In fact, all tested concentrations (100, 50, 25, 12.5, 6.2, and 3.1 µg/mL, P < 0.0001) of the probiotic had a significant inhibitory effect on the growth of Sh. flexneri.
Interestingly, the MIC results for the probiotic’s supernatant against B. cereus were different. The supernatant showed a significant inhibitory effect at concentrations of 100 µg/mL (P < 0.0001) and 25 µg/mL (P < 0.001), but not at 50 µg/mL or at 12.5, 6.2, and 3.1 µg/mL. Therefore, the MIC for B. cereus was considered to be 100 µg/mL. Table 1 and Figure 3A-D present the MIC of B. coagulans supernatant against E. coli, S. typhi, Sh. flexneri, and B. cereus.
The Objective Observations of Minimum Inhibitory Concentration and Minimum Bactericidal Concentration Tests Conducting on Bacillus coagulans Supernatant Against Pathogens a,b, c, d
Pathogen | MIC | MBC | |||||||
---|---|---|---|---|---|---|---|---|---|
Concentration (µg/mL) | Controls | ||||||||
100 | 50 | 25 | 12.5 | 6.25 | 3.1 | P | N | ||
Escherichia coli | + | + | + | + | + | - | - | + | - |
Salmonella typhi | + | + | + | - | - | - | - | + | - |
Shigella flexneri | + | + | + | + | + | + | - | + | - |
Bacillus cereus | + | - | + | - | - | - | - | + | - |
Minimum inhibitory concentration (MIC) of Bacillus coagulans against Escherichia coli, Salmonella typhi, Shigella flexneri, and B.cereus. The symbols * P < 0.05, *** P < 0.001, and **** P < 0.0001 are used to denote statistically significant P-values. Minimum inhibitory concentration results showed that different concentration of probiotic’s supernatant significantly inhibit the growth of pathogens. For E. coli, the MIC is 6.2, for S. typhi, the MIC is 25, for Sh. flexneri, the MIC is 3.1, and for B.cereus the MIC is 25 µg/mL. PC: Positive control, NC: Negative control, C: Concentration.
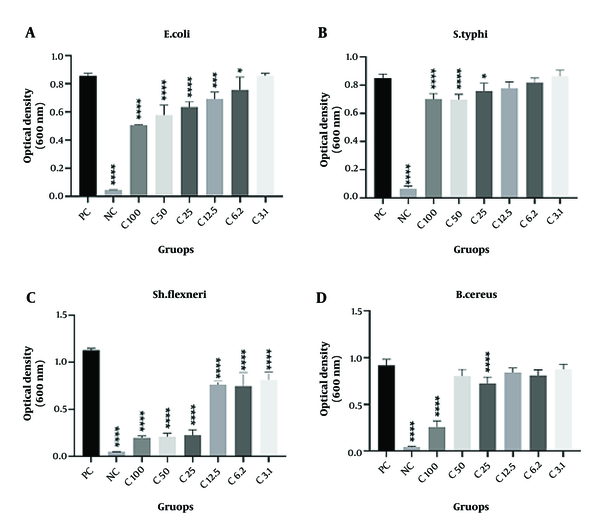
The MBC results indicated that the minimum inhibitory concentration of the supernatant did not have a bactericidal effect on E. coli, S. typhi, Sh. flexneri, and B. cereus, as it did not completely prevent the growth of these bacteria. Consequently, at the concentrations tested in this study, it was not possible to observe a bactericidal effect of the supernatant. The MBC results of B. coagulans supernatant against these four pathogens are also shown in Table 1.
5. Discussion
Intestinal infections are common in the GI tract and are usually caused by various microorganisms such as yeasts, molds, parasites, viruses, and bacteria. Viral and bacterial diarrheas are common symptoms of intestinal infection. Unlike viral diarrhea, which usually resolves after a period of illness, bacterial diarrhea (such as that caused by Shigella, E. coli, Salmonella, and Campylobacter) can sometimes become chronic and prolonged (19). A study by Assefa and Girma in 2019 on Salmonella and Shigella isolates in children with diarrhea showed a significant association between diarrhea in these children and infection by Salmonella (20). Another study conducted by Manhique-Coutinho et al. (as cited by Mwape et al.) in 2022 on the frequency of E. coli strains among children with diarrhea showed that out of 723 analyzed samples, 262 were infected with this pathogen (21). The gram-positive pathogen B. cereus has also been identified as a causative agent of some GI diseases, particularly emesis and diarrhea. This microorganism is commonly responsible for foodborne illness. The diarrheal type of B. cereus is associated with sauces, soups, meat, milk, and vegetables and has been isolated from a wide variety of foods such as seafood, infant foods, ready-to-serve foods, fresh vegetables, rice, and meat and milk products (22).
For many years, antibiotics have been used to treat chronic bacterial diarrhea, but excessive consumption has led to the development of antibiotic resistance in the bacteria causing diarrhea (23, 24). Consequently, in recent years, researchers have focused on probiotics as a suitable alternative to antibiotics due to their effectiveness in treating chronic infectious diarrhea caused by bacteria (2).
In the present study, we aimed to evaluate the antimicrobial efficacy of B. coagulans against three gram-negative (E. coli, S. typhi, and Sh. flexneri) and one gram-positive (B. cereus) enteric pathogens that play an important role in the prevalence of bacterial diarrhea. Bacillus coagulans is a spore-forming probiotic that exhibits characteristics of both the Bacillus and Lactobacillus genera. It produces L-lactic acid but does not produce gas from the fermentation of various sugars such as sucrose, raffinose, trehalose, maltose, and mannitol (14). Upon oral administration, spores of B. coagulans pass through the stomach and enter the duodenum, where they rapidly germinate and multiply (25). As a facultative anaerobe, germinated B. coagulans produces lactic acid in the small intestine and colon (26). Indeed, B. coagulans has been shown to improve GI tract ecology by restoring the quantity of favorable bacteria and antagonizing pathogenic microbes (27). This bacterium is slowly excreted through feces up to seven days after consumption is stopped (Keller, Van Dinter et al. 2017).
The therapeutic effect of B. coagulans is due to the secretion of coagulin, a type of bacteriocin with antibacterial activity against a broad spectrum of enteric microbes (14). Therefore, we prepared and used a cell-free supernatant from B. coagulans culture for our study. Using agar well-diffusion and agar disc-diffusion methods, we evaluated the antimicrobial efficiency of the cell-free supernatant of the probiotic isolate (B. coagulans) against selected pathogenic bacteria (E. coli, S. typhi, Sh. flexneri, and B. cereus). The agar well-diffusion testing showed that the probiotic supernatant did not create any zones of inhibition around the wells for E. coli, S. typhi, Sh. flexneri, and B. cereus, indicating no antibacterial effects of B. coagulans against these pathogens (Figure 1A-D).
Our results were contrary to a study conducted in 2017 by Maranni et al., who investigated the antimicrobial activity of B. pumilus, B. coagulans, B. licheniformis, B. endophyticus, and B. amyloliquefaciens against some standard pathogens as well as clinical antibiotic-resistant strains using spotting and well-diffusion methods. Their study showed antimicrobial activity of Bacillus strains against Acinetobacter, E. coli, Klebsiella, Salmonella, Shigella, Streptococcus, and Listeria as well as methicillin-resistant Staphylococcus aureus and vancomycin-resistant enterococci (28).
Our agar disk-diffusion testing also showed that the cell-free supernatant of B. coagulans had no antibacterial effects against the four selected pathogens (E. coli, S. typhi, Sh. flexneri, and B. cereus). This conclusion was drawn because no zones of inhibition were observed around the disks containing the supernatant in any of the tests. In contrast, zones of inhibition were observed around the positive control disks containing ciprofloxacin and sulfamethoxazole (Figure 2A-D), indicating the inhibition of growth of E. coli, S. typhi, Sh. flexneri, and B. cereus by these antibiotics. Consistent with our results, a study conducted in 2016 that investigated the antimicrobial activity of six different Bacillus strains (including B. coagulans) against eight food pathogens using the disk-diffusion method found that some of these strains had no antimicrobial effect on S. typhi and E. coli (29).
Similarly, a study conducted by Zhang et al. in 2022 on the antimicrobial effect of B. coagulans bacteriocin against Listeria monocytogenes using the MIC method showed that different dilutions of bacteriocin had no antibacterial effect on gram-negative pathogens, while an antibacterial effect of this bacteriocin on the gram-positive B. cereus was observed (30). Conversely, our MIC results showed that different concentrations of B. coagulans supernatant significantly inhibited the growth of the gram-negative pathogens E. coli (MIC = 25 µg/mL), S. typhi (MIC = 50 μg/mL), and Sh. flexneri (MIC = 3.1 μg/mL) (Figure 3A-C). However, our results for the gram-positive bacterium B. cereus were consistent with Zhang et al.'s findings; we observed a significant inhibitory effect of B. coagulans supernatant on B. cereus at concentrations of 100 and 25 µg/mL (MIC = 100 µg/mL) (Figure 3D).
Although the MIC testing demonstrated the antibacterial effects of B. coagulans supernatant on E. coli, S. typhi, Sh. flexneri, and B. cereus, the MBC results showed that the minimum inhibitory concentration of the supernatant had no bactericidal effect to completely prevent the growth of these pathogens (Table 1). This indicates that the concentrations of the supernatant used in this study were not sufficient to investigate its bactericidal effect on the selected pathogens.
5.1. Conclusions and Future Perspective
As an emerging method to control antibiotic-resistant bacteria, the use of probiotics with antibacterial effects has gained increasing attention in recent years. By producing substances such as bacteriocins, probiotics can serve as a suitable alternative to chemical remedies. Several studies have shown that different types of probiotics vary in their effectiveness and secretion of antibacterial substances. An ideal probiotic should be highly safe, functional, and capable of surviving adverse environmental conditions.
In this research, we focused on B. coagulans, a spore-forming, aerobic or facultatively anaerobic, lactic acid-producing, non-pathogenic, and economically important species known for its safety for human consumption. Using agar well-diffusion, agar disc-diffusion, MIC, and MBC methods, we investigated the antimicrobial effects of the cell-free supernatant from B. coagulans cultures against four enteric pathogens (E. coli, S. typhi, Sh. flexneri, and B. cereus) that cause diarrhea.
Although no antimicrobial activity of the probiotic was observed in either the agar well-diffusion or agar disc-diffusion tests, the MIC testing showed a significant antimicrobial effect against all four pathogens. However, no bactericidal effect of this probiotic was observed on the selected pathogens in the MBC testing. The negative results from the agar well-diffusion and agar disc-diffusion tests could be attributed to the lower secretion of antibacterial substances by this probiotic compared to lactic acid bacteria. Furthermore, the culture medium used, the type of pathogens, and the probiotic species significantly impacted the antimicrobial activity observed.
It is important to note that a single study cannot fully reveal all the advantages and beneficial effects of a probiotic strain. Therefore, we believe that further research, including various strains of this probiotic and a wider range of pathogens, could provide more accurate conclusions.
References
-
1.
Logan AC, Jacka FN, Prescott SL. Immune-Microbiota Interactions: Dysbiosis as a Global Health Issue. Current Allergy and Asthma Rep. 2016;16(2). https://doi.org/10.1007/s11882-015-0590-5.
-
2.
Wilkins T, Sequoia J. Probiotics for Gastrointestinal Conditions: A Summary of the Evidence. Am Fam Physician. 2017;96(3):170-8. [PubMed ID: 28762696].
-
3.
Altun GK, Erginkaya Z. Identification and characterization of Bacillus coagulans strains for probiotic activity and safety. LWT. 2021;151:112233.
-
4.
Zommiti M, Chikindas ML, Ferchichi M. Probiotics—Live Biotherapeutics: a Story of Success, Limitations, and Future Prospects—Not Only for Humans. Probiotics Antimicrobial Proteins. 2019;12(3):1266-89. [PubMed ID: 31376026]. https://doi.org/10.1007/s12602-019-09570-5.
-
5.
Cao J, Yu Z, Liu W, Zhao J, Zhang H, Zhai Q, et al. Probiotic characteristics of Bacillus coagulans and associated implications for human health and diseases. J Functional Foods. 2020;64:103643. https://doi.org/10.1016/j.jff.2019.103643.
-
6.
Hong HA, Huang J‐M, Khaneja R, Hiep LV, Urdaci MC, Cutting SM. The safety of Bacillus subtilis and Bacillus indicus as food probiotics. J Appl Microbiol. 2008;105(2):510-20.
-
7.
Mingmongkolchai S, Panbangred W. Bacillus probiotics: an alternative to antibiotics for livestock production. J Appl Microbiol. 2018;124(6):1334-46. [PubMed ID: 29316021]. https://doi.org/10.1111/jam.13690.
-
8.
Berikashvili V, Sokhadze K, Kachlishvili E, Elisashvili V, Chikindas ML. Bacillus amyloliquefaciens Spore Production Under Solid-State Fermentation of Lignocellulosic Residues. Probiotics Antimicrob Proteins. 2018;10(4):755-61. [PubMed ID: 29249066]. https://doi.org/10.1007/s12602-017-9371-x.
-
9.
Elisashvili V, Kachlishvili E, Chikindas ML. Recent Advances in the Physiology of Spore Formation for Bacillus Probiotic Production. Probiotics Antimicrob Proteins. 2019;11(3):731-47. [PubMed ID: 30515722]. https://doi.org/10.1007/s12602-018-9492-x.
-
10.
Popov IV, Algburi A, Prazdnova EV, Mazanko MS, Elisashvili V, Bren AB, et al. A Review of the Effects and Production of Spore-Forming Probiotics for Poultry. Animals. 2021;11(7). https://doi.org/10.3390/ani11071941.
-
11.
Lakshmi SG, Jayanthi N, Saravanan M, Ratna MS. Safety assesment of Bacillus clausii UBBC07, a spore forming probiotic. Toxicol Rep. 2017;4:62-71.
-
12.
Demin KA, Refeld AG, Bogdanova AA, Prazdnova EV, Popov IV, Kutsevalova OY, et al. Mechanisms of Candida Resistance to Antimycotics and Promising Ways to Overcome It: The Role of Probiotics. Probiotics Antimicrob Proteins. 2021;13(4):926-48. [PubMed ID: 33738706]. https://doi.org/10.1007/s12602-021-09776-6.
-
13.
Grant A, Gay CG, Lillehoj HS. Bacillus spp. as direct-fed microbial antibiotic alternatives to enhance growth, immunity, and gut health in poultry. Avian Pathol. 2018;47(4):339-51. [PubMed ID: 29635926]. https://doi.org/10.1080/03079457.2018.1464117.
-
14.
Adibpour N, Hosseininezhad M, Pahlevanlo A, Hussain MA. A review on Bacillus coagulans as a Spore-Forming Probiotic. Appl Food Biotechnol. 2019;6(2):91-100.
-
15.
Elshaghabee FMF, Rokana N, Gulhane RD, Sharma C, Panwar H. Bacillus As Potential Probiotics: Status, Concerns, and Future Perspectives. Front Microbiol. 2017;8:1490. [PubMed ID: 28848511]. [PubMed Central ID: PMC5554123]. https://doi.org/10.3389/fmicb.2017.01490.
-
16.
Konuray G, Erginkaya Z. Potential Use of Bacillus coagulans in the Food Industry. Foods. 2018;7(6). [PubMed ID: 29899254]. [PubMed Central ID: PMC6025323]. https://doi.org/10.3390/foods7060092.
-
17.
Donay JL, Fernandes P, Lagrange PH, Herrmann JL. Evaluation of the inoculation procedure using a 0.25 McFarland standard for the BD Phoenix automated microbiology system. J Clin Microbiol. 2007;45(12):4088-9. [PubMed ID: 17913926]. [PubMed Central ID: PMC2168575]. https://doi.org/10.1128/JCM.01847-07.
-
18.
TopaÇ T, Dalyan CİLo B, AĞCa H, SaĞLam S, Efe K, Ener B. Candida İzolatlarının Antifungal Duyarlılığının Belirlenmesinde Klinik Laboratuvar Standartları Enstitüsü (CLSI) ve Avrupa Antimikrobiyal Duyarlılık Testleri Komitesi (EUCAST) Sıvı Mikrodilüsyon Yöntemlerinin Karşılaştırılması. Mikrobiyoloji Bulteni. 2018;52(1):35-48. https://doi.org/10.5578/mb.63991.
-
19.
Shen H, Zhang J, Li Y, Xie S, Jiang Y, Wu Y, et al. The 12 Gastrointestinal Pathogens Spectrum of Acute Infectious Diarrhea in a Sentinel Hospital, Shenzhen, China. Frontiers Microbiol. 2016;7. https://doi.org/10.3389/fmicb.2016.01926.
-
20.
Assefa A, Girma M. Prevalence and antimicrobial susceptibility patterns of Salmonella and Shigella isolates among children aged below five years with diarrhea attending Robe General Hospital and Goba Referral Hospital, South East Ethiopia. Trop Dis Travel Med Vaccines. 2019;5:19. [PubMed ID: 31832223]. [PubMed Central ID: PMC6864936]. https://doi.org/10.1186/s40794-019-0096-6.
-
21.
Mwape K, Bosomprah S, Chibesa K, Silwamba S, Luchen CC, Sukwa N, et al. Prevalence of Diarrhoeagenic Escherichia coli among Children Aged between 0-36 Months in Peri-Urban Areas of Lusaka. Microorganisms. 2023;11(11). [PubMed ID: 38004801]. [PubMed Central ID: PMC10673189]. https://doi.org/10.3390/microorganisms11112790.
-
22.
Ankolekar C, Rahmati T, Labbe RG. Detection of toxigenic Bacillus cereus and Bacillus thuringiensis spores in U.S. rice. Int J Food Microbiol. 2009;128(3):460-6. [PubMed ID: 19027973]. https://doi.org/10.1016/j.ijfoodmicro.2008.10.006.
-
23.
Ebeid T, Al-Homidan I, Fathi M, Al-Jamaan R, Mostafa M, Abou-Emera O, et al. Impact of probiotics and/or organic acids supplementation on growth performance, microbiota, antioxidative status, and immune response of broilers. Italian J Animal Sci. 2021;20(1):2263-73.
-
24.
Szkaradkiewicz AK, Karpinski T. Probiotics and prebiotics. J Biol Earth Sci. 2013;3(1).
-
25.
Cutting SM, Ricca E. Bacterial spore-formers: friends and foes. FEMS Microbiol Lett. 2014;358(2):107-9. [PubMed ID: 25168928]. https://doi.org/10.1111/1574-6968.12572.
-
26.
Keller D, Van Dinter R, Cash H, Farmer S, Venema K. Bacillus coagulans GBI-30, 6086 increases plant protein digestion in a dynamic, computer-controlled in vitro model of the small intestine (TIM-1). Benef Microbes. 2017;8(3):491-6. [PubMed ID: 28504581]. https://doi.org/10.3920/BM2016.0196.
-
27.
Majeed M, Majeed S, Nagabhushanam K, Natarajan S, Sivakumar A, Ali F. Evaluation of the stability of Bacillus coagulans MTCC 5856 during processing and storage of functional foods. Int J Food Sci Technol. 2016;51(4):894-901. https://doi.org/10.1111/ijfs.13044.
-
28.
Maranni MG, Khourasgani MR, Asadollahi MA, Shafiei R. Evaluation of antimicrobial activity of bacillus strains isolated from various resources. Evaluation. 2017;19(116):68-78.
-
29.
Avcı A, Üzmez S, Alkan FB, Bagana İ, Nurçeli E, Çiftçi E. Antimicrobial activity spectrums of some Bacillus strains from various sources. J Food. 2016;41(5).
-
30.
Zhang J, Gu S, Zhang T, Wu Y, Ma J, Zhao L, et al. Characterization and antibacterial modes of action of bacteriocins from Bacillus coagulans CGMCC 9951 against Listeria monocytogenes. Lwt. 2022;160. https://doi.org/10.1016/j.lwt.2022.113272.