Abstract
Background:
Platelet-leukocyte aggregates have been implicated in various infectious and inflammatory diseases. The Interferon-induced transmembrane protein 3 (IFITM3) protein plays a role in eliminating viral infections, but its role in the severity of COVID-19 is not well understood.Objectives:
We aimed to investigate the correlation between IFITM3 mRNA expression and platelet-monocyte complex levels with the severity of COVID-19, as well as various inflammatory and coagulation markers.Methods:
We conducted a cross-sectional study on 54 COVID-19 patients, classified into severe and mild/moderate subgroups. Demographics and laboratory findings were extracted from patients' medical records. We measured IFITM3 mRNA expression in patients' buffy coats using q-RT-PCR and used flow cytometry with CD61 and CD14 markers to measure platelet-monocyte aggregates.Results:
No significant difference was found in IFITM3 mRNA expression levels or platelet-monocyte complexes between severe and mild/moderate groups (P = 0.067 and P = 0.056). Lymphocyte counts were significantly higher in the mild/moderate subgroup (21.7 ± 8.9 vs 16.3 ± 10.9, P = 0.02), while neutrophil counts were significantly higher in severe patients (78.3 ± 12.2 vs 72.3 ± 9.9, P = 0.01). Additionally, levels of CRP and LDH were significantly higher in severe COVID-19 patients (P = 0.01 and P = 0.001, respectively). A strong positive correlation was observed between the hospitalization period and CRP, CRP with neutrophils and LDH, as well as between O2 saturation and lymphocytes (P < 0.001, P = 0.0003, P = 0.002, and P = 0.005, respectively).Conclusions:
Our findings suggest that IFITM3 gene expression and platelet-monocyte aggregate levels do not correlate with disease outcomes in COVID-19. However, further investigations with larger sample sizes are needed to better understand the mechanisms involved. Monitoring inflammatory and coagulation markers remains important for managing COVID-19 patients.Keywords
Coronavirus Disease 2019 (COVID-19) Platelet-Monocyte Aggregate Interferon-Inducible Transmembrane Protein 3 (IFITM3) Thrombotic Complications
1. Background
Coronavirus Disease 2019 (COVID-19), a viral infectious disease, generally causes severe respiratory syndrome and gastrointestinal and central nervous system complications in patients infected by the severe acute respiratory syndrome coronavirus 2 (SARS-CoV-2) (1). The COVID-19 pandemic has posed a severe global burden, claiming more than 0.8 million lives throughout the world between December 2019 and August 2020 (2, 3). Cytokine storms in COVID-19 patients can cause intensive inflammatory events and a highly pro-thrombotic environment, leading to thrombotic complications (4). Thromboembolic complications are considered life-threatening in COVID-19 patients (5).
The prothrombotic state observed in COVID-19 patients is multifactorial, and platelets play an indispensable role in initiating the thrombotic process. Therefore, platelet activities and functions serve as potential hematologic parameters for monitoring thrombotic complications in COVID-19 patients (6, 7). Studies have shown that platelets in COVID-19 patients secrete elevated levels of IL-1beta, soluble CD40L (8), serum circulating serotonin, and PF4 compared to healthy controls. Moreover, platelet activation cell markers, including P-selectin and CD63, are increased in critically ill COVID-19 patients, indicating a hyperactive phenotype of platelets (5).
Platelets play a crucial role in inflammation and immunity by modulating various leukocyte functions through the release of inflammatory mediators as well as direct cell-cell interaction. Previous studies have found that levels of circulating platelet-leukocyte aggregates increase in patients with inflammatory or thrombotic conditions (9).
Platelet-leukocyte interactions lead to leukocyte mobilization to the inflammation zone, the release of pro-inflammatory mediators, and neutrophil extracellular traps (NET) by leukocytes, oxidative burst, and phagocytosis (10-14). The platelet-leukocyte aggregates are associated with various thrombotic and inflammatory conditions, including severe lung injuries, rheumatoid arthritis, inflammatory bowel disease, and cerebrovascular and cardiovascular disease (15-19). The most important receptors involved in platelet-leukocyte aggregates include P-selectin (CD-62p), CD41/CD61 complex (Glycoprotein IIb/IIIa), and C40L on the platelets, and PSGL-1, Mac-1, and CD40 on the leukocytes (20-22). Monocytes have been shown to have a higher affinity for platelet complexes than other leukocytes, and these complexes serve as a more sensitive marker for platelet activation than P-selectin (23). The interaction between monocytes and platelets among leukocytes represents the most significant binding affinity between P-selectin and PSGL-1 (24-26).
Platelet activation and platelet-leukocyte interaction have been established in the pathophysiology of various viral infections, such as the dengue virus, human immunodeficiency virus (HIV), and influenza virus (27-34). Recent studies have also reported that COVID-19 patients have a higher level of activated platelets and increased interaction of platelets with neutrophils, monocytes, and T cells (35). Moreover, platelet-monocyte aggregate formation results in the expression of tissue factor in monocytes via P-selectin/αIIb/β3 integrin-mediated signaling. The binding of tissue factor with FVII proceeds coagulation cascade activation (5).
Interferon-induced transmembrane protein 3 (IFITM3) is a small protein that plays crucial roles against viral infection in both acquired and innate immune responses (36-45). Interferon-induced transmembrane protein 3 protein is commonly expressed on endosomes and lysosomes and eliminates the fusion of the viral membrane with the host cell membrane in a wide range of capsid viruses like influenza, Ebola, and Marburg (46). Moreover, IFITM3 is active against vesicular stomatitis virus, HIV, respiratory syncytial virus, and Zika virus (47, 48). In addition, IFITM3 is involved in several cellular processes like differentiation, apoptosis, cell adhesion, and modulation of immune cells which can boost immunity against influenza A, West Nile, and dengue viruses, and SARS-CoV (36, 39, 49, 50).
Given the significant social and economic burden of COVID-19, along with the important roles of platelet-monocyte aggregates in initiating inflammation and thrombosis, as well as the pivotal role of IFITM3 in virus elimination, this study sought to explore the association of platelet-monocyte aggregates and IFITM3 mRNA levels with coagulation tests and disease progression in patients with severe and mild/moderate COVID-19.
2. Objectives
By investigating the relationship between these biomarkers and COVID-19 severity, we hope to gain a better understanding of the underlying mechanisms of the disease and identify potential targets for future treatments. This study may also help to identify patients who are at higher risk for thrombotic complications and guide clinical decision-making.
3. Methods
3.1. Study Setting and Subjects
This cross-sectional study was conducted on 54 COVID-19 patients admitted to Ali-Asghar Hospital, Shiraz, Iran, from 2020 to 2021. The sample size was calculated according to the formula n = (Z1-α/2+Z1-β)2/cr2+3. The inclusion criteria were a positive PCR test for SARS-CoV-2, age over 18, and hospitalization time of less than 72 hours before sampling. Patients with a history of comorbidities, including cardiovascular disease, previous respiratory disease, cancer, infection with other acute or chronic viral infections like HIV, HCV, and influenza, were excluded from our study. Moreover, patients who were pregnant or under previous treatment with anticoagulants and antiplatelet regimens were excluded. The included patients were divided into two subgroups: Severe and mild/moderate, according to the WHO classification of COVID-19 severity based on SpO2 (mild/moderate > 94%, severe < 94%). Written informed consent was obtained from all eligible patients before their inclusion in the study.
3.2. Assessment of Medical Records of the Patients
Due to incomplete clinical files, some patients had to be excluded, leaving a final count of 54 patients. The general characteristics of the patients, including age, gender, and hospitalization period, were extracted from their medical records. Moreover, clinical tests including PT, PTT, INR, CBC indices (WBC, RBC, Hg, Lymphocyte, Neutrophil, and platelet count), O2 saturation, LDH, and C-reactive protein (CRP) were obtained from the medical records of the patients.
3.3. Sample Preparation
Four milliliters of venous blood samples were collected in two tubes, 2 ml each, with K2EDTA for each patient according to standard protocol. One series of tubes was assigned for flow cytometry. Another series was centrifuged at 3000 rpm for 7 minutes, and buffy coats were obtained in 1.5 mL micro-tubes and stored at -20°C until subsequent use.
3.4. Quantitative Real-time RT-PCR (q-RT-PCR)
To evaluate the mRNA expression level of the IFITM3 gene in the patients, q-RT-PCR was performed. First, RBCs in buffy coat samples were removed by adding RBC lysing solution and then washed with PBS three times. Then, the total RNA of buffy coat samples was isolated using Trizol (Invitrogen) according to the manufacturer’s protocol. The quality and quantity of extracted RNA were evaluated using Nanodrop (Thermo ScientificTM) and agarose gel electrophoresis. Next, single-strand cDNA synthesis was conducted using Easy cDNA Synthesis Kit (ParstousTM, Iran) according to the manufacturer’s instructions. To check the accuracy of the cDNA synthesis reaction, qPCR with a housekeeping gene primer, GAPDH, was carried out for all synthesized cDNA samples (the sequence and product size of the primers are shown in Table 1). After that, q-PCR was performed using the Cycler Rotor Gene Q instrument (Qiagen, Germany) with SYBR Green Master Mix (ParstousTM, Iran). The reaction contained SYBR Green (10 µL), cDNA (1 µL), forward and reverse primer (20 pmol), and nuclease-free water to a final volume of 20 µL. The thermocycling program included an initial heat activation and denaturation at 94°C for 10 minutes, followed by 40 cycles of 95°C for 15 seconds, an annealing step for 30 seconds at 60°C, and an extension step for 30 seconds at 72°C. The mRNA expression level of IFITM3 was normalized with the GAPDH mRNA expression level. Melting curve and amplification plot analysis were performed to check qPCR reaction specificity. Moreover, the PCR products were electrophoresed on 2% agarose gel to evaluate the primers’ specificity. The relative expression level was calculated using the 2-∆Ct method as explained elsewhere. The reactions were conducted with at least two replicates and in the presence of no template control and reference gene in each experiment. All qPCR experiments were conducted according to standard protocol.
The Sequence and Product Size of the Primers
Genes | Sequences (5’ to 3’) | PCR Product Size (bp) |
---|---|---|
IFITM3 | 173 | |
Forward | ATAGCATTCGCCTACTCCGTG | |
Reverse | CCATAGGCCTGGAAGATCAGCA | |
GAPDH | 110 | |
Forward | CACCAGGGCTGCTTTTAACTCTGGA | |
Reverse | CCTTGACGGTGCCATGGAATTTGC |
3.5. Evaluation of Platelet-Monocyte Aggregates by Flow Cytometry
First, 50 μL of whole blood of COVID-19 patients were incubated with FITC-labeled anti-CD14 (Clone: HCD14, BioLegend) along with a PE-labeled anti-CD61 antibody (Clone: VIPL2, BD Biosciences, CA, USA) for 20 minutes in the dark at room temperature. Then, red blood cells were lysed with 1x ammonium chloride. Finally, samples were analyzed on BD FACSCalibur™ flow cytometer (BD Biosciences, CA, USA). The detection results were plotted on a CD14 vs. SSC dot plot for mononuclear cells (MNCs). Then, the CD14+ cells were plotted on a CD14 vs. CD61 dot plot. The CD14+CD61+ complexes were considered platelet-monocyte aggregates. Finally, data were processed using FlowJo TM v10 software.
3.6. Statistical Analysis
The data were analyzed using GraphPad Prism 9. The Shapiro test was used to check the normal distribution of the data. To determine the difference in the mean of the data, t-tests or Mann-Whitney tests were used for parametric and non-parametric data, respectively. The data were considered significant when the P-value was below 0.05. The correlation of variables was evaluated using the Spearman test. The r-value of 1 and -1 was considered a strong positive and reverse correlation, respectively.
4. Results
4.1. Patient Characteristics
This cross-sectional study included 54 hospitalized COVID-19 patients. According to the WHO classification for COVID-19 severity, 38.9% (n = 21) of the patients were classified as severe, and 61.1% (n = 33) as mild/moderate. Among those with severe COVID-19, 11 patients were male and 10 were female, while those with mild/moderate form comprised 15 males and 18 females. Furthermore, the majority (88.9%, n = 48) of the patients were in non-ICU wards, but six patients were in the ICU. Two subjects with severe COVID-19 expired during the study period. The overall average age of the patients was 49 ± 16.3 years, with a higher mean age observed in those with severe form compared to mild/moderate (52 ± 14.5 vs. 41 ± 17.38, respectively).
As expected, the lymphocyte count was significantly higher in the mild/moderate group (21.7 ± 8.9 vs. 16.3 ± 10.9, P = 0.02), while the neutrophil count was significantly higher in severe patients (78.3 ± 12.2 vs. 72.3 ± 9.9, P = 0.01). Furthermore, the levels of CRP and LDH were significantly higher in severe COVID-19 patients (P = 0.01 and P = 0.001, respectively). O2 saturation level was significantly higher in the mild/moderate group compared to severe patients (94.8 ± 0.9 vs. 85.4 ± 11.6, P < 0.001) (Table 2).
Patients’ Characteristics and Their General Laboratory Findings
Parameter | Severe (n = 21) | Mild/Moderate (n = 33) | Total (n = 54) | P-Value | |||
---|---|---|---|---|---|---|---|
Mean (± SD) | Min-Max | Mean (± SD) | Min-Max | Mean (± SD) | Min-Max | ||
Age | 52 ± 14.5 | 32-86 | 47 ± 17.38 | 21 - 84 | 49 ± 16.3 | 23 – 86 | 0.28 |
HP (days) | 10.48 ± 10.1 | 4-36 | 4.9 ± 1.0 | 3 - 7 | 7 ± 6.8 | 3 - 36 | 0.17 |
O2 saturation | 85.4 ± 11.6 | 51-93 | 94.8 ± 0.9 | 93 - 97 | 91.1 ± 8.5 | 51 - 97 | < 0.001 |
WBC (count × 103) | 6.6 ± 3.0 | 3.1-15.4 | 5.3 ± 1.7 | 2.5 - 9 | 5.8 ± 2.3 | 15.4 - 2.50 | 0.16 |
Lymphocyte | 16.3 ± 10.9 | 5.6 - 38.6 | 21.7 ± 8.9 | 7.5 - 39.9 | 19.6 ± 10.0 | 5.6 - 39.9 | 0.02 |
Neutrophil | 78.3 ± 12.2 | 53.6 - 92.2 | 72.3 ± 9.9 | 52.2-84.3 | 74.7 ± 11.1 | 52.2 - 92.2 | 0.01 |
RBC (count × 106) | 4.8 ± 0.7 | 2.8 - 6.3 | 4.7 ± 0.5 | 3.7-5.9 | 4.8 ± 0.6 | 2.8 - 6.31 | 0.93 |
Hg (mg/dL) | 13.7 ± 2.1 | 8.5 - 17.3 | 14.0 ± 1.6 | 10.9-19.7 | 13.9 ± 1.8 | 8.5 - 19.7 | 0.82 |
PLT count (count × 103) | 182 ± 70 | 51 - 360 | 184 ± 60 | 75-329 | 183 ± 63 | 51 - 360 | 0.91 |
PT (second) | 15.2 ± 2.8 | 11.5 - 22.9 | 15 ± 1.5 | 13-18 | 15.1 ± 2 | 11.5 - 22.9 | 0.76 |
INR (IU) | 1.3 ± 0.3 | 0.8 - 2.3 | 1.2 ± 0.2 | 1-1.6 | 1.2 ± 0.2 | 0.8 - 2.3 | 0.82 |
PTT (second) | 35 ± 8.9 | 22 - 65 | 37.3 ± 17.1 | 25-120 | 36.4 ± 14.5 | 22 - 120 | 0.9 |
CRP | 58.8 ± 32.9 | 3 - 90 | 36 ± 28.2 | 3-88 | 44.9 ± 31.9 | 3 - 90 | 0.01 |
LDH | 633 ± 157 | 279 - 859 | 498 ± 158 | 228-987 | 549 ± 169 | 228 - 987 | 0.001 |
4.2. Interferon-Induced Transmembrane Protein 3 (mRNA Expression)
To evaluate the mRNA expression level of the IFITM3 gene, qRT-PCR was carried out. As shown in Figure 1, no significant difference was observed in the expression of the IFITM3 gene between severe and mild/moderate COVID-19 patients (P = 0.067).
The relative mRNA expression of interferon-induced transmembrane protein 3 (IFITM3) gene in severe and mild/moderate COVID-19 patients. No significant difference was found
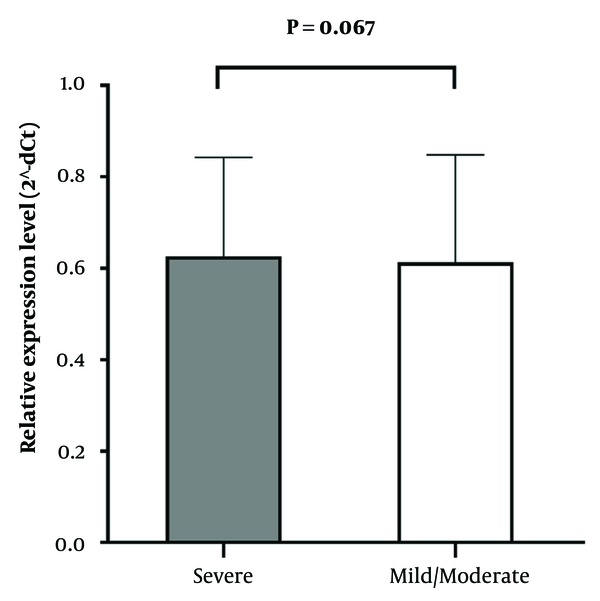
4.3. Platelet-Monocyte Aggregate Formation Level
A flow cytometry assay was carried out to detect CD61 and CD14 cell surface markers to measure the level of platelet-monocyte complex formation. Although the percentage of MNCs was significantly higher in mild/moderate COVID-19 patients compared to severe patients (P = 0.032), there was no significant difference in platelet-monocyte complex formation between the two study groups (P = 0.056) (Figures 2 and 3). The key receptors contributing to platelet-leukocyte aggregates encompass P-selectin (CD-62p), CD41/CD61 complex (Glycoprotein IIb/IIIa), and CD40L on platelets, as well as PSGL-1, Mac-1, and CD40 on leukocytes (20-22). Monocytes exhibit a greater affinity for platelet complexes compared to other leukocytes, making these complexes a more sensitive marker for platelet activation than P-selectin (23).
The comparison of platelet-monocyte aggregate formation level in severe and mild/moderate COVID-19 patients. No significant difference was found.
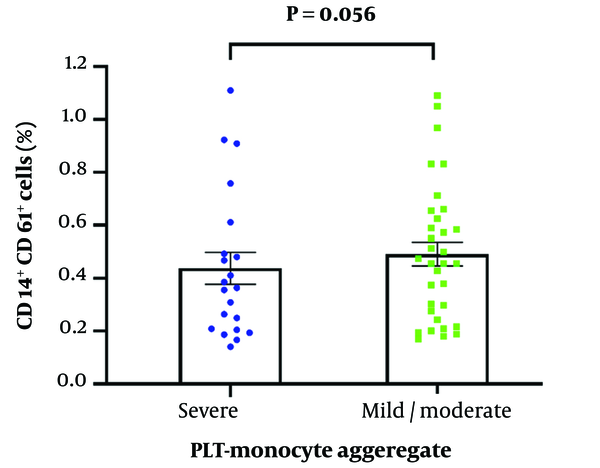
The comparison of monocyte cells level in severe and mild/moderate COVID-19 patients
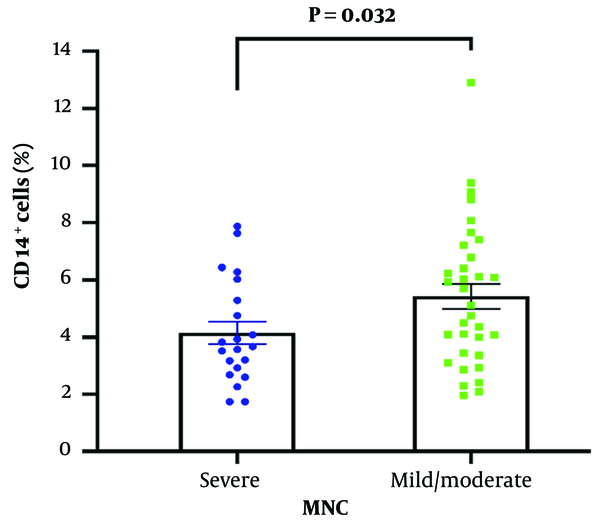
4.4. Correlation of Variables
The Spearman statistical test showed that lymphocyte and MNC counts were positively correlated with platelet-monocyte aggregate formation (P = 0.04, and P < 0.001, respectively), while the neutrophil count was inversely correlated with platelet-monocyte aggregate formation (P = 0.01, r = -0.32) (Figure 4). However, the IFITM3 mRNA level was not correlated with any parameter. A strong positive correlation was observed between the hospitalization period and CRP (P < 0.001), CRP with neutrophils and LDH (P = 0.0003 and P = 0.002, respectively), as well as between O2 saturation and lymphocytes (P = 0.005). The correlation values of other variables are shown in Figure 4 as well.
The correlation of the clinical and laboratory findings in COVID-19 patients
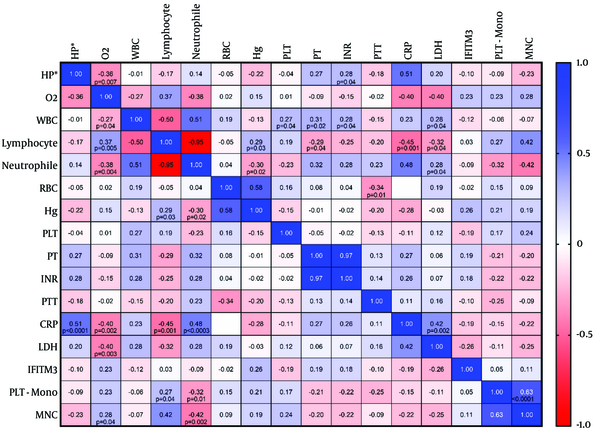
5. Discussion
In this study, we sought to investigate the potential correlation between IFITM3 mRNA expression levels and platelet-monocyte complex formation with COVID-19 severity, as well as various inflammatory and coagulation markers, in a cohort of 54 hospitalized COVID-19 patients. We aimed to gain insight into the underlying mechanisms of COVID-19 pathogenesis and identify potential targets for therapeutic interventions.
We determined the mRNA expression level of IFITM3 in the buffy coat of COVID-19 patients using qRT-PCR and found no significant difference between our two study groups. Previous studies have reported that IFITMs (1, 2, and 3) can eliminate viral pathogens (36-50). However, the pro- and anti-viral activities of IFITMs have been reported for coronaviruses. A toxicogenomic analysis reported that IFITM3 is upregulated in SARS-CoV-2-infected human bronchial epithelium (51).
Interferon-induced transmembrane proteins have been found to hinder the entry of human coronaviruses, including SARS-CoV-1, SARS-CoV-2, and MERS-CoV, in artificial experimental conditions where viral particles were pseudotyped with Spike (S) proteins and cell lines overexpressed IFITMs (39, 52-56). However, a recent study found that endogenous IFITM expression assists SARS-CoV-2 membrane fusion with host human primary lung cells. The study showed that genuine SARS-CoV-2 Spike proteins hijack IFITMs to promote efficient viral infection and suggested that targeting IFITMs could inhibit SARS-CoV-2 infection of human lung cells (55). The reason behind the contradictory effects of overexpressed and endogenous IFITMs is not yet completely understood. However, it has been reported that specific mutations in IFITM3 affecting its topology may change its inhibitory role to an enhancer of coronavirus infection (52, 57). Moreover, two SNPs of IFITM3 have been associated with the risk of acquiring SARS-CoV-2 infection (56).
The small population of the present study may prevent the finding of the possible relationship between IFITM3 gene expression level and the prognosis of COVID-19 patients. Another point to consider is that most of the mentioned studies have examined the role of IFITM3 protein in the resistance to virus entry in lung epithelial cells, but our study examined the expression of this gene in blood monocytes. It is important to consider, however, that our study had the advantage of involving human subjects rather than an in-vitro experiment. Further studies with larger pre-genotyped study subjects may shed light on the mechanisms behind IFITM3.
We used flow cytometry to detect CD14, a well-known monocyte marker, and CD61 (GPIIIa), a platelet/megakaryocyte marker, to evaluate the level of platelet-monocyte complex formation. Our findings showed that the level of PLT-Monocyte aggregates was not statistically different between our study groups.
Activated platelets are connected with monocytes in PLT-Monocyte aggregates via P-selectin (58). We know that monocytes have fundamental roles in thromboinflammatory disease progression by their pro-inflammatory and coagulation roles. Increased levels of PLT-Monocyte aggregates are documented in many inflammatory and infectious diseases (58). There are well-established studies reporting increased levels of PLT-Monocyte aggregates in COVID-19 with positive correlations with more severe outcomes and thrombotic events (59-62). Inconsistent results, which show no or negative correlation of PLT-Monocyte aggregates with disease severity, have also been documented (63, 64). One obstacle of our study is the absence of a normal control group. Normal subjects were included in most of the mentioned studies that report increased levels of PLT-Monocyte aggregates.
These controversial results may be attributed to differences in the virus species of SARS-CoV infecting the patients, the time of blood sampling, and the consumption of platelet inhibitors like aspirin. Additionally, platelet count may be a confounding factor for the level of platelet-monocyte aggregates.
As expected, lymphocytopenia was significantly higher in severe COVID-19 patients compared to mild/moderate patients. Furthermore, significantly higher levels of LDH and CRP in severe patients indicate a more intense inflammatory environment. These results are consistent with previous studies on COVID-19 patients (65) and support the findings of Xiang et al., who reported severe lymphocytopenia, inflammatory cytokine escalation, and elevated CRP and LDH in fatal COVID-19 patients (66). Xiang et al. also observed severe tissue damage, lymphocyte apoptosis, and SARS-CoV-2 RNA accumulation in the spleen and hilar lymph node. However, our findings for coagulation tests, including PT and PTT, were not statistically different between our two study populations, which is consistent with a previous investigation (67).
We also observed a relatively strong positive correlation between the hospitalization period and CRP, a well-known inflammation biomarker, as well as between CRP and neutrophil count and LDH. In contrast, we observed a strong reverse correlation between the hospitalization period and O2 saturation, O2 saturation and neutrophil count, and CRP and LDH. Furthermore, we found a negative correlation between lymphocyte count and CRP and LDH. These findings are consistent with previous studies (68, 69) and suggest that a more inflammatory environment is associated with more severe clinical outcomes in COVID-19 patients.
5.1. Conclusions
Overall, the present study did not find any significant correlations between IFITM3 gene expression and platelet-monocyte aggregate levels with disease outcomes in COVID-19 patients. The research aimed to investigate the relationship between these biomarkers and COVID-19 severity to gain a better understanding of the disease mechanisms and potential treatment targets. While previous studies have shown the role of IFITM3 in eliminating viral infections, the results of this study did not show a clear association with COVID-19 severity. Monitoring inflammatory and coagulation markers remains crucial for managing COVID-19 patients. Further investigations with larger sample sizes as well as the investigation of aggregates with other sensitive methods may provide more insights into the mechanisms involved.
Acknowledgements
References
-
1.
Yesudhas D, Srivastava A, Gromiha MM. COVID-19 outbreak: history, mechanism, transmission, structural studies and therapeutics. Infect. 2021;49(2):199-213. [PubMed ID: 32886331]. [PubMed Central ID: PMC7472674]. https://doi.org/10.1007/s15010-020-01516-2.
-
2.
Huang C, Wang Y, Li X, Ren L, Zhao J, Hu Y, et al. Clinical features of patients infected with 2019 novel coronavirus in Wuhan, China. Lancet. 2020;395(10223):497-506. [PubMed ID: 31986264]. [PubMed Central ID: PMC7159299]. https://doi.org/10.1016/S0140-6736(20)30183-5.
-
3.
Chan JF, Yuan S, Kok KH, To KK, Chu H, Yang J, et al. A familial cluster of pneumonia associated with the 2019 novel coronavirus indicating person-to-person transmission: a study of a family cluster. Lancet. 2020;395(10223):514-23. [PubMed ID: 31986261]. [PubMed Central ID: PMC7159286]. https://doi.org/10.1016/S0140-6736(20)30154-9.
-
4.
Dharra R, Kumar Sharma A, Datta S. Emerging aspects of cytokine storm in COVID-19: The role of proinflammatory cytokines and therapeutic prospects. Cytokine. 2023;169:156287. [PubMed ID: 37402337]. [PubMed Central ID: PMC10291296]. https://doi.org/10.1016/j.cyto.2023.156287.
-
5.
Sciaudone A, Corkrey H, Humphries F, Koupenova M. Platelets and SARS-CoV-2 During COVID-19: Immunity, Thrombosis, and Beyond. Circ Res. 2023;132(10):1272-89. [PubMed ID: 37167360]. [PubMed Central ID: PMC10171305]. https://doi.org/10.1161/CIRCRESAHA.122.321930.
-
6.
Mohammed MA. Fighting cytokine storm and immunomodulatory deficiency: By using natural products therapy up to now. Front Pharmacol. 2023;14:1111329. [PubMed ID: 37124230]. [PubMed Central ID: PMC10134036]. https://doi.org/10.3389/fphar.2023.1111329.
-
7.
Jevtic SD, Nazy I. The COVID Complex: A Review of Platelet Activation and Immune Complexes in COVID-19. Front Immunol. 2022;13:807934. [PubMed ID: 35371058]. [PubMed Central ID: PMC8965558]. https://doi.org/10.3389/fimmu.2022.807934.
-
8.
Zaid Y, Puhm F, Allaeys I, Naya A, Oudghiri M, Khalki L, et al. Platelets Can Associate with SARS-Cov-2 RNA and Are Hyperactivated in COVID-19. Circ Res. 2020;127(11):1404-18. [PubMed ID: 32938299]. [PubMed Central ID: PMC7641188]. https://doi.org/10.1161/CIRCRESAHA.120.317703.
-
9.
Klenk C, Erber J, Fresacher D, Rohrl S, Lengl M, Heim D, et al. Platelet aggregates detected using quantitative phase imaging associate with COVID-19 severity. Commun Med (Lond). 2023;3(1):161. [PubMed ID: 37935793]. [PubMed Central ID: PMC10630365]. https://doi.org/10.1038/s43856-023-00395-6.
-
10.
Badrnya S, Schrottmaier WC, Kral JB, Yaiw KC, Volf I, Schabbauer G, et al. Platelets mediate oxidized low-density lipoprotein-induced monocyte extravasation and foam cell formation. Arterioscler Thromb Vasc Biol. 2014;34(3):571-80. [PubMed ID: 24371083]. https://doi.org/10.1161/ATVBAHA.113.302919.
-
11.
Diacovo TG, Roth SJ, Buccola JM, Bainton DF, Springer TA. Neutrophil rolling, arrest, and transmigration across activated, surface-adherent platelets via sequential action of P-selectin and the beta 2-integrin CD11b/CD18. Blood. 1996;88(1):146-57. [PubMed ID: 8704169].
-
12.
Weyrich AS, Elstad MR, McEver RP, McIntyre TM, Moore KL, Morrissey JH, et al. Activated platelets signal chemokine synthesis by human monocytes. J Clin Invest. 1996;97(6):1525-34. [PubMed ID: 8617886]. [PubMed Central ID: PMC507213]. https://doi.org/10.1172/JCI118575.
-
13.
Christersson C, Johnell M, Siegbahn A. Tissue factor and IL8 production by P-selectin-dependent platelet-monocyte aggregates in whole blood involves phosphorylation of Lyn and is inhibited by IL10. J Thromb Haemost. 2008;6(6):986-94. [PubMed ID: 18363812]. https://doi.org/10.1111/j.1538-7836.2008.02956.x.
-
14.
McDonald B, Urrutia R, Yipp BG, Jenne CN, Kubes P. Intravascular neutrophil extracellular traps capture bacteria from the bloodstream during sepsis. Cell Host Microbe. 2012;12(3):324-33. [PubMed ID: 22980329]. https://doi.org/10.1016/j.chom.2012.06.011.
-
15.
Zarbock A, Singbartl K, Ley K. Complete reversal of acid-induced acute lung injury by blocking of platelet-neutrophil aggregation. J Clin Invest. 2006;116(12):3211-9. [PubMed ID: 17143330]. [PubMed Central ID: PMC1679711]. https://doi.org/10.1172/JCI29499.
-
16.
Bunescu A, Seideman P, Lenkei R, Levin K, Egberg N. Enhanced Fcgamma receptor I, alphaMbeta2 integrin receptor expression by monocytes and neutrophils in rheumatoid arthritis: interaction with platelets. J Rheumatol. 2004;31(12):2347-55. [PubMed ID: 15570633].
-
17.
Irving PM, Macey MG, Feakins RM, Knowles CH, Frye JN, Liyanage SH, et al. Platelet-leucocyte aggregates form in the mesenteric vasculature in patients with ulcerative colitis. Eur J Gastroenterol Hepatol. 2008;20(4):283-9. [PubMed ID: 18334871]. https://doi.org/10.1097/MEG.0b013e3282f246c2.
-
18.
Pamuk GE, Vural O, Turgut B, Demir M, Umit H, Tezel A. Increased circulating platelet-neutrophil, platelet-monocyte complexes, and platelet activation in patients with ulcerative colitis: a comparative study. Am J Hematol. 2006;81(10):753-9. [PubMed ID: 16941615]. https://doi.org/10.1002/ajh.20655.
-
19.
Kuligowski MP, Kitching AR, Hickey MJ. Leukocyte recruitment to the inflamed glomerulus: a critical role for platelet-derived P-selectin in the absence of rolling. J Immunol. 2006;176(11):6991-9. [PubMed ID: 16709860]. https://doi.org/10.4049/jimmunol.176.11.6991.
-
20.
Haselmayer P, Grosse-Hovest L, von Landenberg P, Schild H, Radsak MP. TREM-1 ligand expression on platelets enhances neutrophil activation. Blood. 2007;110(3):1029-35. [PubMed ID: 17452516]. https://doi.org/10.1182/blood-2007-01-069195.
-
21.
Kurose I, Anderson DC, Miyasaka M, Tamatani T, Paulson JC, Todd RF, et al. Molecular determinants of reperfusion-induced leukocyte adhesion and vascular protein leakage. Circ Res. 1994;74(2):336-43. [PubMed ID: 7507416]. https://doi.org/10.1161/01.res.74.2.336.
-
22.
Lievens D, Zernecke A, Seijkens T, Soehnlein O, Beckers L, Munnix IC, et al. Platelet CD40L mediates thrombotic and inflammatory processes in atherosclerosis. Blood. 2010;116(20):4317-27. [PubMed ID: 20705757]. [PubMed Central ID: PMC2993630]. https://doi.org/10.1182/blood-2010-01-261206.
-
23.
Michelson AD, Barnard MR, Krueger LA, Valeri CR, Furman MI. Circulating monocyte-platelet aggregates are a more sensitive marker of in vivo platelet activation than platelet surface P-selectin: studies in baboons, human coronary intervention, and human acute myocardial infarction. Circulation. 2001;104(13):1533-7. [PubMed ID: 11571248]. https://doi.org/10.1161/hc3801.095588.
-
24.
Ahn KC, Jun AJ, Pawar P, Jadhav S, Napier S, McCarty OJ, et al. Preferential binding of platelets to monocytes over neutrophils under flow. Biochem Biophys Res Commun. 2005;329(1):345-55. [PubMed ID: 15721313]. https://doi.org/10.1016/j.bbrc.2005.01.146.
-
25.
Li N, Goodall AH, Hjemdahl P. A sensitive flow cytometric assay for circulating platelet-leucocyte aggregates. Br J Haematol. 1997;99(4):808-16. [PubMed ID: 9432026]. https://doi.org/10.1046/j.1365-2141.1997.4993305.x.
-
26.
Nagasawa A, Matsuno K, Tamura S, Hayasaka K, Shimizu C, Moriyama T. The basis examination of leukocyte-platelet aggregates with CD45 gating as a novel platelet activation marker. Int J Lab Hematol. 2013;35(5):534-41. [PubMed ID: 23356866]. https://doi.org/10.1111/ijlh.12051.
-
27.
Hottz ED, Medeiros-de-Moraes IM, Vieira-de-Abreu A, de Assis EF, Vals-de-Souza R, Castro-Faria-Neto HC, et al. Platelet activation and apoptosis modulate monocyte inflammatory responses in dengue. J Immunol. 2014;193(4):1864-72. [PubMed ID: 25015827]. [PubMed Central ID: PMC4137323]. https://doi.org/10.4049/jimmunol.1400091.
-
28.
Hottz ED, Bozza FA, Bozza PT. Platelets in Immune Response to Virus and Immunopathology of Viral Infections. Front Med (Lausanne). 2018;5:121. [PubMed ID: 29761104]. [PubMed Central ID: PMC5936789]. https://doi.org/10.3389/fmed.2018.00121.
-
29.
Sung PS, Huang TF, Hsieh SL. Extracellular vesicles from CLEC2-activated platelets enhance dengue virus-induced lethality via CLEC5A/TLR2. Nat Commun. 2019;10(1):2402. [PubMed ID: 31160588]. [PubMed Central ID: PMC6546763]. https://doi.org/10.1038/s41467-019-10360-4.
-
30.
Hottz ED, Quirino-Teixeira AC, Valls-de-Souza R, Zimmerman GA, Bozza FA, Bozza PT. Platelet function in HIV plus dengue coinfection associates with reduced inflammation and milder dengue illness. Sci Rep. 2019;9(1):7096. [PubMed ID: 31068600]. [PubMed Central ID: PMC6506591]. https://doi.org/10.1038/s41598-019-43275-7.
-
31.
Mesquita EC, Hottz ED, Amancio RT, Carneiro AB, Palhinha L, Coelho LE, et al. Persistent platelet activation and apoptosis in virologically suppressed HIV-infected individuals. Sci Rep. 2018;8(1):14999. [PubMed ID: 30301959]. [PubMed Central ID: PMC6178345]. https://doi.org/10.1038/s41598-018-33403-0.
-
32.
Koupenova M, Corkrey HA, Vitseva O, Manni G, Pang CJ, Clancy L, et al. The role of platelets in mediating a response to human influenza infection. Nat Commun. 2019;10(1):1780. [PubMed ID: 30992428]. [PubMed Central ID: PMC6467905]. https://doi.org/10.1038/s41467-019-09607-x.
-
33.
Hottz ED, Lopes JF, Freitas C, Valls-de-Souza R, Oliveira MF, Bozza MT, et al. Platelets mediate increased endothelium permeability in dengue through NLRP3-inflammasome activation. Blood. 2013;122(20):3405-14. [PubMed ID: 24009231]. [PubMed Central ID: PMC3829114]. https://doi.org/10.1182/blood-2013-05-504449.
-
34.
Quirino-Teixeira AC, Rozini SV, Barbosa-Lima G, Coelho DR, Carneiro PH, Mohana-Borges R, et al. Inflammatory signaling in dengue-infected platelets requires translation and secretion of nonstructural protein 1. Blood Adv. 2020;4(9):2018-31. [PubMed ID: 32396616]. [PubMed Central ID: PMC7218431]. https://doi.org/10.1182/bloodadvances.2019001169.
-
35.
Battinelli EM. COVID-19 concerns aggregate around platelets. Blood. 2020;136(11):1221-3. [PubMed ID: 32957116]. [PubMed Central ID: PMC7483434]. https://doi.org/10.1182/blood.2020007805.
-
36.
Ajanel A, Middleton EA. Alterations in the megakaryocyte transcriptome impacts platelet function in sepsis and COVID-19 infection. Thromb Res. 2023;231:247-54. [PubMed ID: 37258336]. [PubMed Central ID: PMC10198739]. https://doi.org/10.1016/j.thromres.2023.05.015.
-
37.
Yount JS, Moltedo B, Yang YY, Charron G, Moran TM, Lopez CB, et al. Palmitoylome profiling reveals S-palmitoylation-dependent antiviral activity of IFITM3. Nat Chem Biol. 2010;6(8):610-4. [PubMed ID: 20601941]. [PubMed Central ID: PMC2928251]. https://doi.org/10.1038/nchembio.405.
-
38.
Weidner JM, Jiang D, Pan XB, Chang J, Block TM, Guo JT. Interferon-induced cell membrane proteins, IFITM3 and tetherin, inhibit vesicular stomatitis virus infection via distinct mechanisms. J Virol. 2010;84(24):12646-57. [PubMed ID: 20943977]. [PubMed Central ID: PMC3004348]. https://doi.org/10.1128/JVI.01328-10.
-
39.
Huang IC, Bailey CC, Weyer JL, Radoshitzky SR, Becker MM, Chiang JJ, et al. Distinct patterns of IFITM-mediated restriction of filoviruses, SARS coronavirus, and influenza A virus. PLoS Pathog. 2011;7(1). e1001258. [PubMed ID: 21253575]. [PubMed Central ID: PMC3017121]. https://doi.org/10.1371/journal.ppat.1001258.
-
40.
Schoggins JW, Wilson SJ, Panis M, Murphy MY, Jones CT, Bieniasz P, et al. A diverse range of gene products are effectors of the type I interferon antiviral response. Nature. 2011;472(7344):481-5. [PubMed ID: 21478870]. [PubMed Central ID: PMC3409588]. https://doi.org/10.1038/nature09907.
-
41.
Schoggins JW, MacDuff DA, Imanaka N, Gainey MD, Shrestha B, Eitson JL, et al. Pan-viral specificity of IFN-induced genes reveals new roles for cGAS in innate immunity. Nature. 2014;505(7485):691-5. [PubMed ID: 24284630]. [PubMed Central ID: PMC4077721]. https://doi.org/10.1038/nature12862.
-
42.
Anafu AA, Bowen CH, Chin CR, Brass AL, Holm GH. Interferon-inducible transmembrane protein 3 (IFITM3) restricts reovirus cell entry. J Biol Chem. 2013;288(24):17261-71. [PubMed ID: 23649619]. [PubMed Central ID: PMC3682530]. https://doi.org/10.1074/jbc.M112.438515.
-
43.
Lu J, Pan Q, Rong L, He W, Liu SL, Liang C. The IFITM proteins inhibit HIV-1 infection. J Virol. 2011;85(5):2126-37. [PubMed ID: 21177806]. [PubMed Central ID: PMC3067758]. https://doi.org/10.1128/JVI.01531-10.
-
44.
Mudhasani R, Tran JP, Retterer C, Radoshitzky SR, Kota KP, Altamura LA, et al. IFITM-2 and IFITM-3 but not IFITM-1 restrict Rift Valley fever virus. J Virol. 2013;87(15):8451-64. [PubMed ID: 23720721]. [PubMed Central ID: PMC3719792]. https://doi.org/10.1128/JVI.03382-12.
-
45.
Wilkins C, Woodward J, Lau DT, Barnes A, Joyce M, McFarlane N, et al. IFITM1 is a tight junction protein that inhibits hepatitis C virus entry. Hepatol. 2013;57(2):461-9. [PubMed ID: 22996292]. [PubMed Central ID: PMC3566288]. https://doi.org/10.1002/hep.26066.
-
46.
Diamond MS, Farzan M. The broad-spectrum antiviral functions of IFIT and IFITM proteins. Nat Rev Immunol. 2013;13(1):46-57. [PubMed ID: 23237964]. [PubMed Central ID: PMC3773942]. https://doi.org/10.1038/nri3344.
-
47.
Savidis G, Perreira JM, Portmann JM, Meraner P, Guo Z, Green S, et al. The IFITMs Inhibit Zika Virus Replication. Cell Rep. 2016;15(11):2323-30. [PubMed ID: 27268505]. https://doi.org/10.1016/j.celrep.2016.05.074.
-
48.
Monel B, Compton AA, Bruel T, Amraoui S, Burlaud-Gaillard J, Roy N, et al. Zika virus induces massive cytoplasmic vacuolization and paraptosis-like death in infected cells. EMBO J. 2017;36(12):1653-68. [PubMed ID: 28473450]. [PubMed Central ID: PMC5470047]. https://doi.org/10.15252/embj.201695597.
-
49.
Bailey CC, Zhong G, Huang IC, Farzan M. IFITM-Family Proteins: The Cell's First Line of Antiviral Defense. Annu Rev Virol. 2014;1:261-83. [PubMed ID: 25599080]. [PubMed Central ID: PMC4295558]. https://doi.org/10.1146/annurev-virology-031413-085537.
-
50.
Kim YC, Jeong MJ, Jeong BH. Strong association of regulatory single nucleotide polymorphisms (SNPs) of the IFITM3 gene with influenza H1N1 2009 pandemic virus infection. Cell Mol Immunol. 2020;17(6):662-4. [PubMed ID: 31685927]. [PubMed Central ID: PMC7264285]. https://doi.org/10.1038/s41423-019-0322-1.
-
51.
Hachim MY, Al Heialy S, Hachim IY, Halwani R, Senok AC, Maghazachi AA, et al. Interferon-Induced Transmembrane Protein (IFITM3) Is Upregulated Explicitly in SARS-CoV-2 Infected Lung Epithelial Cells. Front Immunol. 2020;11:1372. [PubMed ID: 32595654]. [PubMed Central ID: PMC7301886]. https://doi.org/10.3389/fimmu.2020.01372.
-
52.
Shi G, Kenney AD, Kudryashova E, Zani A, Zhang L, Lai KK, et al. Opposing activities of IFITM proteins in SARS-CoV-2 infection. EMBO J. 2021;40(3). e106501. [PubMed ID: 33270927]. [PubMed Central ID: PMC7744865]. https://doi.org/10.15252/embj.2020106501.
-
53.
Wrensch F, Winkler M, Pohlmann S. IFITM proteins inhibit entry driven by the MERS-coronavirus spike protein: evidence for cholesterol-independent mechanisms. Viruses. 2014;6(9):3683-98. [PubMed ID: 25256397]. [PubMed Central ID: PMC4189045]. https://doi.org/10.3390/v6093683.
-
54.
Winstone H, Lista MJ, Reid AC, Bouton C, Pickering S, Galao RP, et al. The Polybasic Cleavage Site in SARS-CoV-2 Spike Modulates Viral Sensitivity to Type I Interferon and IFITM2. J Virol. 2021;95(9). [PubMed ID: 33563656]. [PubMed Central ID: PMC8104117]. https://doi.org/10.1128/JVI.02422-20.
-
55.
Prelli Bozzo C, Nchioua R, Volcic M, Koepke L, Kruger J, Schutz D, et al. IFITM proteins promote SARS-CoV-2 infection and are targets for virus inhibition in vitro. Nat Commun. 2021;12(1):4584. [PubMed ID: 34321474]. [PubMed Central ID: PMC8319209]. https://doi.org/10.1038/s41467-021-24817-y.
-
56.
Xu F, Wang G, Zhao F, Huang Y, Fan Z, Mei S, et al. IFITM3 Inhibits SARS-CoV-2 Infection and Is Associated with COVID-19 Susceptibility. Viruses. 2022;14(11). [PubMed ID: 36423162]. [PubMed Central ID: PMC9692367]. https://doi.org/10.3390/v14112553.
-
57.
Zhao X, Sehgal M, Hou Z, Cheng J, Shu S, Wu S, et al. Identification of Residues Controlling Restriction versus Enhancing Activities of IFITM Proteins on Entry of Human Coronaviruses. J Virol. 2018;92(6). [PubMed ID: 29263263]. [PubMed Central ID: PMC5827390]. https://doi.org/10.1128/JVI.01535-17.
-
58.
Rolling CC, Barrett TJ, Berger JS. Platelet-monocyte aggregates: molecular mediators of thromboinflammation. Front Cardiovasc Med. 2023;10:960398. [PubMed ID: 37255704]. [PubMed Central ID: PMC10225702]. https://doi.org/10.3389/fcvm.2023.960398.
-
59.
Hottz ED, Martins-Goncalves R, Palhinha L, Azevedo-Quintanilha IG, de Campos MM, Sacramento CQ, et al. Platelet-monocyte interaction amplifies thromboinflammation through tissue factor signaling in COVID-19. Blood Adv. 2022;6(17):5085-99. [PubMed ID: 35420680]. [PubMed Central ID: PMC9015715]. https://doi.org/10.1182/bloodadvances.2021006680.
-
60.
Barrett TJ, Cornwell M, Myndzar K, Rolling CC, Xia Y, Drenkova K, et al. Platelets amplify endotheliopathy in COVID-19. Sci Adv. 2021;7(37):eabh2434. [PubMed ID: 34516880]. [PubMed Central ID: PMC8442885]. https://doi.org/10.1126/sciadv.abh2434.
-
61.
Barrett TJ, Bilaloglu S, Cornwell M, Burgess HM, Virginio VW, Drenkova K, et al. Platelets contribute to disease severity in COVID-19. J Thromb Haemost. 2021;19(12):3139-53. [PubMed ID: 34538015]. [PubMed Central ID: PMC8646651]. https://doi.org/10.1111/jth.15534.
-
62.
Hottz ED, Azevedo-Quintanilha IG, Palhinha L, Teixeira L, Barreto EA, Pao CRR, et al. Platelet activation and platelet-monocyte aggregate formation trigger tissue factor expression in patients with severe COVID-19. Blood. 2020;136(11):1330-41. [PubMed ID: 32678428]. [PubMed Central ID: PMC7483437]. https://doi.org/10.1182/blood.2020007252.
-
63.
Srihirun S, Sriwantana T, Srichatrapimuk S, Vivithanaporn P, Kirdlarp S, Sungkanuparph S, et al. Increased platelet activation and lower platelet-monocyte aggregates in COVID-19 patients with severe pneumonia. PLoS One. 2023;18(3). e0282785. [PubMed ID: 36888618]. [PubMed Central ID: PMC9994685]. https://doi.org/10.1371/journal.pone.0282785.
-
64.
Scavone M, Ghali C, Calogiuri M, Sala M, Bossi E, Mencarini T, et al. Impairment of platelet function in both mild and severe COVID-19 patients. Br J Haematol. 2023;203(4):656-67. [PubMed ID: 37615207]. https://doi.org/10.1111/bjh.19062.
-
65.
Ghahramani S, Tabrizi R, Lankarani KB, Kashani SMA, Rezaei S, Zeidi N, et al. Laboratory features of severe vs. non-severe COVID-19 patients in Asian populations: a systematic review and meta-analysis. Eur J Med Res. 2020;25(1):30. [PubMed ID: 32746929]. [PubMed Central ID: PMC7396942]. https://doi.org/10.1186/s40001-020-00432-3.
-
66.
Xiang Q, Feng Z, Diao B, Tu C, Qiao Q, Yang H, et al. SARS-CoV-2 Induces Lymphocytopenia by Promoting Inflammation and Decimates Secondary Lymphoid Organs. Front Immunol. 2021;12:661052. [PubMed ID: 33995382]. [PubMed Central ID: PMC8113960]. https://doi.org/10.3389/fimmu.2021.661052.
-
67.
Lin J, Yan H, Chen H, He C, Lin C, He H, et al. COVID-19 and coagulation dysfunction in adults: A systematic review and meta-analysis. J Med Virol. 2021;93(2):934-44. [PubMed ID: 32706426]. [PubMed Central ID: PMC7405098]. https://doi.org/10.1002/jmv.26346.
-
68.
Devang N, Sreelatha S, BV M. Assessment of inflammatory markers and their association with disease mortality in severe COVID-19 patients of tertiary care hospital in South India. Egypt J Bronchol. 2022;16(1):55.
-
69.
Poggiali E, Zaino D, Immovilli P, Rovero L, Losi G, Dacrema A, et al. Lactate dehydrogenase and C-reactive protein as predictors of respiratory failure in CoVID-19 patients. Clin Chim Acta. 2020;509:135-8. [PubMed ID: 32531257]. [PubMed Central ID: PMC7282743]. https://doi.org/10.1016/j.cca.2020.06.012.