Abstract
Keywords
Antibacterial Activity Antifungal Activity Aqueous and Methanolic Extracts Essential Oils Red Beet Leaves
1. Background
Despite the expansion of antibiotics regarding their numbers and effectiveness, bacterial and fungal infections are still a major issue in medicine. In addition, the rapid development of microbial resistance to the existing antibiotics has become a new challenge (1). Foodborne diseases caused by contaminated foods with pathogenic bacteria is still classified as a high-priority public health problem (2). Therefore, searching for new antimicrobial agents is an ongoing process (3). Over the last decade, the application of natural extracts obtained from domestic plants, which is prevalently used by our predecessors as a remedy, in medicines and preservation of foods and cosmetics, has surged significantly. Herbal drugs have been extensively used in this field for many centuries. Since these natural products are more available, non-toxic, biodegradable, and have fewer side-effects in comparison with common antibiotics or preservatives, they have recently been widely considered (1).
In Iranian traditional medicine, the leaves of red beet are used to treat some infections, like vaginal and skin infections. However, to the best of our knowledge, no research has yet been conducted on the antimicrobial activity of this plant.
2. Objectives
The present study is part of a comprehensive investigation in searching for alternative natural antimicrobials and preservatives, which gives way toward a new plant source for controlling pathogens and food spoilage microbes.
3. Methods
3.1. Plant Materials
The leaves of red beet Beta vulgaris, Johari variety, were purchased from a local farm in Isfahan, Iran, in October 2017. The leaves were washed twice with tap and distilled water, respectively. The leaves were dried by a cabinet dryer (Hydrotec, Iran) under 25°C and ventilation for 48 - 72 h. Subsequently, dried leaves were ground by a grinder (Achtung, Germany) using mesh no. 10 (2 mm diameter) (4).
3.2. Preparation of Extracts
The dried powder of the leaves (20 g) was soaked in 200 ml distilled water and left at room temperature for 48 h while being stirred at 150 rpm. Similarly, 20 g of the leaves were soaked in 200 ml of methanol (80% v/v) in flasks, sealed with foil, and stirred for 48 h under the same condition. The aqueous and methanolic extracts were filtered out using a filter paper (Whatman no. 1) and concentrated in a rotary vacuum evaporator at 50°C. The residues were dried at -56°C by a freeze-dryer (Christ, alpha 1 - 2 LD plus, UK). Final powders were stored at 4°C until subsequent use (5).
3.3. Essential Oils Isolation
Essential oils were extracted from the grounded leaves (50 g) by hydro-distillation method using a Clevenger-type apparatus. The extraction was carried out in 2 L distilled water for 4.5 h; up to the point that no more oil obtained (6). The essential oil was collected and stored at 4°C until subsequent use.
3.4. Test Microorganisms
Five reference bacteria, including Staphylococcus aureus, Bacillus cereus PTCC 1154, Escherichia coli PTCC 1395, Salmonella enteritidis, and Pseudomonas aeruginosa PTCC 1310, and five fungi, including Aspergillus niger PTCC 5012, Byssochlamys fulva PTCC 5062, Geotrichum candidum, Fuzarium oxysporum PTCC 5115 and Alternaria alternata PTCC 5224 were used in this study. The test microorganisms were provided by Microbial Culture Collection of Food Microbiology Laboratory, College of Agriculture, Isfahan University of Technology.
Cultures of the bacteria were grown in brain heart infusion (BHI) broth (Merck, Germany) at 37°C for 24 h aerobically and stored on BHI agar slants at 4°C. Fungi were cultivated on potato dextrose agar (PDA) (Merck, Germany) and incubated at 30°C for 48 h. The cultures were maintained on PDA slants at 4°C. Bacteria maintained on agar slants were recovered for experiments by inoculating into BHI broth and incubated at 37°C until the concentration of the microorganisms reached 108 CFU/mL. For recovering fungi, the cultures were inoculated on PDA plates and incubated at 30°C for 72 h (7, 8).
3.5. Antibacterial Activity Evaluation
3.5.1. Disc-Diffusion Assay
In order to prepare paper discs, a 100-mg plant extract was added to 1 mL dimethyl sulfoxide (DMSO) (Merck, Germany) and sterilized by a 0.45 µm cellulose acetate filter (Millipore, USA). Then, 50 µL of 100 mg/mL plant extract (equivalent to 5 mg dosage) and 50 µL DMSO were applied to 6 mm diameter paper discs (Puya-Teb, Iran). Next, 100 μL of the prepared inoculum (equal to 108 CFU/mL) was pipetted on to the Mueller-Hinton agar (MHA) plates. A sterile L-shaped glass rod was used to completely spread the inoculum on the plates by streaking over the surface with rotation. Then, the inoculated discs were placed on the top of the MHA plates. Ampicillin and chloramphenicol discs were used (Puya-Teb, Iran) as positive controls for determining the susceptibility of tested bacteria. Moreover, a disc impregnated with pure DMSO (solvent) was used as a negative control. The plates were incubated at 37°C for 48 h and the formation of inhibition zone (IZ) around the discs was considered as an antibacterial activity of the extracts (3).
3.5.2. Measurement of Minimum Inhibitory Concentration
Measurement of minimum inhibitory concentration (MIC) of the samples was investigated through the broth dilution method. A 100-µL bacterial suspension at a concentration of l05 CFU/mL was added to each 1.5-mL Eppendorf tubes containing 200 µL sterile BHI. Then, the test compounds (methanolic or water extractions or essential oil of red beat leaves) were added to give the ultimate concentrations of 50, 100, 250, and 500 µg/mL. The tubes were then incubated at 37°C for 24 h. A 100-µL sample of each tube was taken and cultivated on MHA plates. Plates were incubated at 37°C for 24 h. The least concentration, at which no growth occurred on the plates was considered as MIC (9). Antibiotics and a solvent were used as positive and negative controls, respectively. All experiments were carried out in triplicate.
3.6. Antifungal Activity Investigation
For studying the antifungal activity of the aqueous and methanolic extracts and essential oils of red beet leaves, contact phase (CP) method was used. PDA medium was autoclaved and cooled in a water bath to 40°C. Extracts and essential oils were thoroughly mixed with a sterile molten medium at 40°C in the flasks to obtain the final concentration of 5% (v/v). The PDA media containing essential oils and extracts were poured into sterile 8 cm Petri dishes (22 mL/plate). Agar discs (5 mm diameter) from the edge of fungi cultures were placed at the center of each plate and incubated at 30°C for 72 h. The mean radial mycelial growth of the fungi was determined by measuring the diameter of the colony in two directions. The growth was compared with the control plate, in which the same fungi were grown on an untreated PDA medium incubated under the same condition. Three replications were done for each treatment. The mean growth values were measured and converted to the inhibition percentage of mycelial growth (MGI) in relation to the control treatment using Equation 1:
Where, dc and dt are mycelial growth diameter in control and treated plates, respectively (10). In this experiment, ethylene diamine tetra-acetate (EDTA) was used as a positive control.
3.7. Data Analysis
Experiments were carried out in triplicate, and all data were averaged. Statistical analysis was carried out through a completely randomized design by SPSS software. Significant differences between the means were determined by Duncan’s multiple range test at P ≤ 0.05.
4. Results
4.1. Antibacterial Activity
4.1.1. Disc Diffusion Method
In this study, to investigate the ability of methanolic and aqueous extracts of red beet leaves and their essential oils to prohibit the growth of tested bacteria, the disc diffusion assay was employed, which is a common test for determining the antimicrobial activity of various materials. In this test, the antimicrobial effect of compounds was determined by measuring the diameter of an inhibition zone around the disks, where microbial growth was inhibited (Table 1).
Antibacterial Activity of the Extracts of Red Beet Leaves Against Tested Bacterial Species by Disc Diffusion Method (5 mg/disc)
Bacteria | Treatment | ||||
---|---|---|---|---|---|
Methanolic Extract | Water Extract | Essential Oils | Negative Control | Positive Control | |
Escherichia coli | + | - | - | - | + |
B. cereus | + | - | + | - | + |
P. aeruginosa | - | - | - | - | + |
S. enteritidis | + | - | + | - | + |
St. aureus | + | - | + | - | + |
As shown in Table 1, the methanolic extract of red beet leaves had an inhibitory effect on the growth of some bacteria, including E. coli, B. cereus, S. enteritidis, and St. aureus. The aqueous extract of red beet leaves showed no antimicrobial activity against all tested bacterial species. Essential oils of red beet leaves had an inhibitory effect against three bacterial species, including B. cereus, S. enteritidis, and St. aureus.
It is noteworthy that P. aeruginosa was resistant to all extracts and essential oils. E. coli showed sensitivity only to the methanolic extract.
Thus, the MIC was determined by the broth dilution method only for bacteria, which showed susceptibility in the disk diffusion method.
4.1.2. Minimum Inhibitory Concentration
The results of the minimum inhibitory concentration (MIC) test of the samples are shown in Table 2. Since the disk diffusion method is a qualitative test and many factors influence its accuracy, the MIC test was used to measure the antibacterial activity of the samples. In this test, after adding different concentrations of the antimicrobial substances to the tubes containing test microorganisms, the least concentration that inhibited the growth of microbes in media was considered as MIC. According to Table 2, a higher amount of MIC represents the lower antimicrobial activity of the compound or higher bacterial resistance.
Minimum Inhibitory Concentrations of the Methanolic Extract and Essential Oils of Red Beet Leaves (µg/mL)a, b
Treatment | Bacteria | |||
---|---|---|---|---|
Escherichia coli | B. cereus | S. enteritidis | St. aureus | |
Methanolic extract | 500A | 250B, C | > 500A | 100C |
Essential oils | ND | 50C | 250B, C | 100C |
Antibiotics (positive control)c | 5D | 10D | 2D | 5D |
Except for P. aeruginosa, which was completely resistant against the methanolic extract in the disk diffusion test, MIC of this extract was evaluated against other bacteria, and the results are demonstrated in Table 2. E. coli and S. enteritidis showed the highest resistance to methanolic extract, whereas St. aureus was the most sensitive bacteria as it showed the lowest MIC.
Moreover, MIC of essential oils was examined against all test bacteria except for P. aeruginosa and E. coli, which both were completely resistant in the disk diffusion test (Table 2). Essential oils had higher antimicrobial potential against St. aureus and B. cereus compared with S. enteritidis.
MIC of the antibiotics, including Chloramphenicol and Ampicillin, as positive controls, are also presented in Table 2. Considering MIC values for methanolic extract and essential oils, the antibacterial effect of red beet leaves extracts was not as strong as antibiotics.
4.2. Antifungal Activity
Figure 1 presents the average data obtained by measuring the antifungal activity of essential oils, as well as the aqueous and methanolic extracts of red beet leaves against various fungi. The percentage of inhibition is shown for all strains tested. The results showed that antifungal properties depended on the type of fungi and also the method of extraction i.e., aqueous or methanolic extract or essential oils. It was observed that the most and the least sensitive fungi were Fusarium and Alternaria, respectively.
EDTA, as a strong fungicide, had the highest percentage of inhibition. There were significant differences between the inhibition percentage of EDTA and the extracts (P < 0.05). Antifungal activity of different treatments against tested fungi was as follows: methanolic extract > essential oils > aqueous extract. Growth inhibition percentage of the extracts was calculated in the range of 8% - 69%. According to the results, the highest inhibitory effect was found for the effect of water extract on Alternaria (percentage of inhibitions = 69%), and the lowest was the effect of water extract on Fusarium (percentage of inhibitions = 8%).
Antifungal activity of different extracts of red beet leaves by the contact phase method (each column is the average of three data).
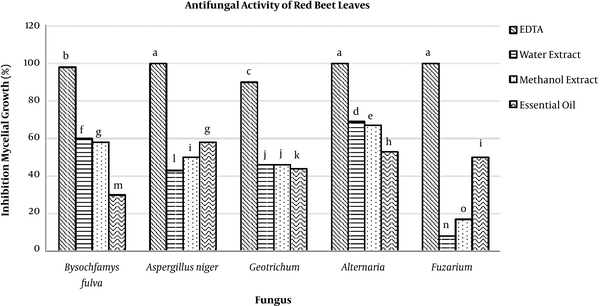
5. Discussion
The obtained results demonstrated that the aqueous extract of red beet leaves had no significant antimicrobial effect against none of the test bacteria. However, the methanolic extract and essential oils included some compounds with antibacterial activity. These results are in accordance with previous studies reporting methanol as a better solvent for the extraction of antimicrobial substances from plants compared with other solvents, such as water, ethanol, or hexane (5, 11). A research team has recently used various solvents, such as water, methanol, ethyl acetate, and petroleum ether, for the extraction of antimicrobial compounds from remedial plants of India. They observed that methanolic extracts of almost all plants showed higher inhibitory activity against microbial indicators than aqueous extracts. However, they finally introduced ethyl acetate as the best solvent for the extraction of antimicrobials (12). Our findings are in agreement with results reported by Casagrande et al. (13), who reported the aqueous extract of Baccharis dracunculifolia with no antimicrobial activity against B. cereus, E. coli, P. aeruginosa, and S. enteritidis with slight activity against St. aureus.
Measuring MIC of the methanolic extract and essential oils of red beet leaves showed that Gram-negative bacteria, including P. aeruginosa, E. coli, and S. enteritidis were more resistant than Gram-positive bacteria, such as St. aureus and B. cereus. Such susceptibility differences among microorganisms against antimicrobial substances may be explained by considering their cell wall composition and/or inherited genes on plasmids (5). For instance, infections caused by P. aeruginosa are among the most difficult to deal with using conventional antibiotics (14), and it was observed in this study that P. aeruginosa was resistant to all tested extracts. Generally, Gram-positive bacteria are more susceptible to plant antibiotic substances in comparison with Gram-negative bacteria, which has confirmed by several studies. For example, investigations on antimicrobial properties of volatile oils of black pepper, clove, nutmeg, oregano, geranium, and thyme (15), essential oils of cinnamon, clove, thyme, and 19 other plants (16), essential oils of Corydothymus capitatus, Cinnamomum cassia, Origanum heracleoticum, Satureja montana, and Cinnamomum verum (2). Sgariglia et al. (17) demonstrated that all tested strains of B. cereus and St. aureus, as Gram-positive indicators, were susceptible to antibacterial components isolated from Caesalpinia paraguariensis bark. However, several Gram-negative indicators were resistant to similar plant antimicrobials.
It is worth mentioning that penicillin and some other antibiotic agents with fungal sources also represent their inhibitory action selectively, and most of them are active against Gram-positive bacteria (14). It can be explained by the fact that Gram-negative bacteria display a central resistance to a wide variety of antimicrobial agents, which is associated with the hydrophobic surface of their outer membrane that forms a barrier against toxic agents and is rich in lipopolysaccharide molecules. Small hydrophilic molecules can freely pass through the outer membrane because of the porin proteins (1, 18); However, access of many compounds to the peptidoglycan layer of the cell wall is hindered by outer lipopolysaccharide layer of Gram-negative bacteria (14). The same mechanism was also reported for bacterial resistance of Gram-negative strains to bacteriocins as the outer membrane covers the receptor of these species-specific antibacterial peptides and inhibits their bactericidal activity (19).
The mechanism of action of essential oils against Gram-positive bacteria and fungi seems to be similar. The oil components destroy the bacterial and fungal cell walls and membrane, which leads to a leakage of cytoplasm and its coagulation (1, 20). Essential oils also inhibit the synthesis of DNA, RNA, proteins, and polysaccharides in fungal and bacterial cells (21). In many cases, the antifungal activity of essential oils is caused by an interaction between these oils and some compounds, such as phenols, ethers, aldehydes, ketones, alcohols, and hydrocarbons. Also, it seems that the inhibition effect of essential oils against fungi is strongly influenced by its main ingredients, as some of these have a strong antifungal effect individually (22). Wang et al. (23) evaluated the antifungal activity of 11 plant essential oils against several toxigenic molds, including A. flavus, A. carbonarius, and P. viridicatum. They reported essential oils obtained from four plants, including Cinnamomum cassia, Litsea cubeba, Cymbopogon martini, and Thymus mongolicus with stronger activity against indicators (23). Essential oils isolated from peppermint also showed significant antifungal activity against Alt. alternaria, F. tabacinum, Penicillum spp., and A. fumigates. These antifungal activities can be due to chemicals containing heteroatoms i.e., oxygen (24).
Our results also demonstrated that EDTA had a considerable antifungal activity that can inhibit the growth of molds significantly and that is why it is considered as a positive control for antifungal activity tests. Gil et al. (25) attributed the antifungal activity of EDTA to high-molecular-weight mannoproteins (HMWM), with mycelium walls that are blocked in EDTA-treated cells. Other studies also revealed the antifungal effect of EDTA as a chelating agent, which significantly decreased the metabolic activity of Candida albicans and destabilized its biofilm leading to inhibiting fungal proliferation (26)
5.1. Conclusions
In this study, the antibacterial and antifungal properties of red beet leaves were studied. Experiments were carried out on methanolic extract, water extract, and essential oils of the leaves. To the best of our knowledge, this is the first study in which the methanolic extract and essential oils of red beet leaves were reported to contain some antimicrobial agents. The methanolic extract had an inhibitory effect on the growth of four bacterial species, including E. coli, B. cereus, S. enteritidis, and St. aureus. Moreover, essential oils showed an antibacterial effect against three bacterial species of B. cereus, S. enteritidis, and St. aureus. The water extract had no antimicrobial activity against tested bacteria. Therefore, it is suggested that methanol is a better solvent for extracting antimicrobial materials compared with water. However, essential oils and the methanolic extract of red beet leaves selectively exerted a great inhibitory activity against Gram-positive microorganisms regarding their amount of MICs in comparison with Gram-negative bacteria.
In addition, studying antifungal properties revealed that the water extract, methanolic extract, and essential oils had an approximately 50% antifungal effect depended on the type of extracts or fungi genus.
It can be concluded that by adding the extracts of red beet leaves to foodstuffs, it can retard microbial contamination, and consequently reduce the onset of spoilage and pathogenicity.
References
-
1.
Kalemba D, Kunicka A. Antibacterial and antifungal properties of essential oils. Current medicinal chemistry. 2003;10(10):813-29. [PubMed ID: 12678685]. https://doi.org/10.2174/0929867033457719.
-
2.
Oussalah M, Caillet S, Saucier L, Lacroix M. Inhibitory effects of selected plant essential oils on the growth of four pathogenic bacteria: E. coli O157: H7, Salmonella typhimurium, Staphylococcus aureus and Listeria monocytogenes. Food control. 2007;18(5):414-20. https://doi.org/10.2174/0929867033457719.
-
3.
Hussain Z, Mohammad P, Sadozai SK, Khan KM, Nawaz Y, Parveen S. Extraction of anti-pneumonia fractions from the leaves of sugar beets Beta vulgaris. Journal of Pharmacy Research. 2011;4(12):4783-5.
-
4.
Lin J, Opoku AR, Geheeb-Keller M, Hutchings AD, Terblanche SE, K. Jäger A, et al. Preliminary screening of some traditional zulu medicinal plants for anti-inflammatory and anti-microbial activities. Journal of Ethnopharmacology. 1999;68(1):267-74. https://doi.org/10.1016/S0378-8741(99)00130-0.
-
5.
Karaman I, Şahin F, Güllüce M, Öǧütçü H, Şengül M, Adıgüzel A. Antimicrobial activity of aqueous and methanol extracts of Juniperus oxycedrus L. Journal of ethnopharmacology. 2003;85(2):231-5. https://doi.org/10.1016/S0378-8741(03)00006-0.
-
6.
Khalil N, Ashour M, Fikry S, Singab AN, Salama O. Chemical composition and antimicrobial activity of the essential oils of selected Apiaceous fruits. Future Journal of Pharmaceutical Sciences. 2018;4(1):88-92. https://doi.org/10.1016/j.fjps.2017.10.004.
-
7.
Canadanovic-Brunet JM, Savatovic SS, Cetkovic GS, Viilic JJ, Djilas SM, Markov SAL, et al. Antioxidant and antimicrobial activities of beet root pomace extracts. Czech Journal of Food Sciences. 2011;29(6):575-85. https://doi.org/10.17221/210/2010-CJFS.
-
8.
Hsieh P, Mau J, Huang S. Antimicrobial effect of various combinations of plant extracts. Food Microbiology. 2001;18(1):35-43. https://doi.org/10.1006/fmic.2000.0376.
-
9.
Lee YL, Cesario T, Wang Y, Shanbrom E, Thrupp L. Antibacterial activity of vegetables and juices. Nutrition. 2003;19(11-12):994-6. [PubMed ID: 14624951]. https://doi.org/10.1016/j.nut.2003.08.003.
-
10.
Manssouri M, Znini M, Harrak A, Majidi L. Antifungal activity of essential oil from the fruits of Ammodaucus leucotrichus Coss & Dur, in liquid and vapour phase against postharvest phytopathogenic fungi in apples. Journal of Applied Pharmaceutical Science. 2016;6(5):131-6. https://doi.org/10.7324/JAPS.2016.60520.
-
11.
Eloff JN. Which extractant should be used for the screening and isolation of antimicrobial components from plants? Journal of ethnopharmacology. 1998;60(1):1-8. https://doi.org/10.1016/S0378-8741(97)00123-2.
-
12.
Arulmozhi P, Vijayakumar S, Kumar T. Phytochemical analysis and antimicrobial activity of some medicinal plants against selected pathogenic microorganisms. Microbial Pathogenesis. 2018;123:219-26. [PubMed ID: 30009969]. https://doi.org/10.1016/j.micpath.2018.07.009.
-
13.
Casagrande M, Zanela J, Wagner A, Busso C, Wouk J, Iurckevicz G, et al. Influence of time, temperature and solvent on the extraction of bioactive compounds of Baccharis dracunculifolia: In vitro antioxidant activity, antimicrobial potential, and phenolic compound quantification. Industrial Crops and Products. 2018;125:207-19. https://doi.org/10.1016/j.indcrop.2018.08.088.
-
14.
Kumar VP, Chauhan NS, Padh H, Rajani M. Search for antibacterial and antifungal agents from selected Indian medicinal plants. Journal of ethnopharmacology. 2006;107(2):182-8. [PubMed ID: 16678369]. https://doi.org/10.1016/j.jep.2006.03.013.
-
15.
Dorman HJD, Deans SG. Antimicrobial agents from plants: antibacterial activity of plant volatile oils. Journal of applied microbiology. 2000;88(2):308-16. [PubMed ID: 10736000]. https://doi.org/10.1046/j.1365-2672.2000.00969.x.
-
16.
Smith-Palmer A, Stewart J, Fyfe L. Antimicrobial properties of plant essential oils and essences against five important food-borne pathogens. Letters in applied microbiology. 1998;26(2):118-22. [PubMed ID: 9569693]. https://doi.org/10.1046/j.1472-765X.1998.00303.x.
-
17.
Sgariglia MA, Soberón JR, Sampietro DA, Quiroga EN, Vattuone MA. Isolation of antibacterial components from infusion of Caesalpinia paraguariensis bark. A bio-guided phytochemical study. Food Chemistry. 2011;126(2):395-404. https://doi.org/10.1016/j.foodchem.2010.10.104.
-
18.
Nikaido H. Prevention of drug access to bacterial targets: permeability barriers and active efflux. Science. 1994;264(5157):382-8. [PubMed ID: 8153625]. https://doi.org/10.1126/science.8153625.
-
19.
Zaeim D, Soleimanian-Zad S, Sheikh-Zeinoddin M. Identification and partial characterization of a bacteriocin-like inhibitory substance (BLIS) from Lb. Bulgaricus K41 isolated from indigenous yogurts. Journal of Food Science. 2014;79(1):67-73. [PubMed ID: 24279356]. https://doi.org/10.1111/1750-3841.12314.
-
20.
de Billerbeck VG, Roques CG, Bessière J, Fonvieille J, Dargent R. Effects of Cymbopogon nardus (L.) W. Watson essential oil on the growth and morphogenesis of Aspergillus niger. Canadian journal of microbiology. 2001;47(1):9-17. [PubMed ID: 15049444]. https://doi.org/10.1139/w00-117.
-
21.
Himejima M, Kubo I. Fungicidal activity of polygodial in combination with anethole and indole against Candida albicans. Journal of agricultural and food chemistry. 1993;41(10):1776-9. https://doi.org/10.1021/jf00034a048.
-
22.
Tzortzakis NG. Ethanol, vinegar and Origanum vulgare oil vapour suppress the development of anthracnose rot in tomato fruit. International journal of food microbiology. 2010;142(1):14-8. [PubMed ID: 20576303]. https://doi.org/10.1016/j.ijfoodmicro.2010.05.005.
-
23.
Wang H, Yang Z, Ying G, Yang M, Nian Y, Wei F, et al. Antifungal evaluation of plant essential oils and their major components against toxigenic fungi. Industrial Crops and Products. 2018;120:180-6. https://doi.org/10.1016/j.indcrop.2018.04.053.
-
24.
Desam NR, Al-Rajab AJ, Sharma M, Mylabathula MM, Gowkanapalli RR, Albratty M. Chemical constituents, in vitro antibacterial and antifungal activity of Mentha×Piperita L. (peppermint) essential oils. Journal of King Saud University - Science. 2017. https://doi.org/10.1016/j.jksus.2017.07.013.
-
25.
Gil ML, Casanova M, Martínez JP. Changes in the cell wall glycoprotein composition of Candida albicans associated to the inhibition of germ tube formation by EDTA. Archives of microbiology. 1994;161(6):489-94. [PubMed ID: 8048840]. https://doi.org/10.1007/BF00307769.
-
26.
Casalinuovo IA, Sorge R, Bonelli G, Di Francesco P. Evaluation of the antifungal effect of EDTA, a metal chelator agent, on Candida albicans biofilm. European review for medical and pharmacological sciences. 2017;21(6):1413-20. [PubMed ID: 28387883].