Abstract
Background:
Glucocorticoid (GC) is a fundamental drug used to treat asthma. GC binds to its corresponding receptor (GR) to formulate a complex that increases the production of anti-inflammatory factors and decreases the amount of pro-inflammatory mediators, covering many cytokines. GR is a nuclear receptor superfamily protein, encoded by NR3C1 gene. Studies suggest that polymorphisms of the NR3C1 gene contribute to a decreased response to GC for the treatment of asthma, even leading to drug-resistance. Also, TGF-β1 plays a central role in airway remodeling, GC significantly inhibits the production of TGF-β1, and TGF-β1 can induce GC resistance. Thus, it is possible that the polymorphisms of the NR3C1 gene can affect the expression of TGF-β1 mRNA and tissue remodeling.Objectives:
This study evaluates the effect of polymorphisms (TthIII1, BclI, ER22/23EK, and N363S) of the NR3C1 GR gene on TGF-β1 mRNA expression in children with asthma.Methods:
The samples of this study included 52 outpatients (age range: 6 - 14 years) with asthma referred to Huai’an First People’s Hospital, Nanjing Medical University, from January 2018 to June 2019. Meanwhile, 40 healthy volunteers were included as the control group.Results:
The polymorphisms of the NR3C1 GR gene were identified using polymerase chain reaction-restriction fragment length polymorphism (PCR-RFLP), and TGF-β1 mRNA levels were measured by real-time reverse transcription (RT)-PCR. TthIII1 and TGF-β1 mRNA expression levels had significant (P = 0.011) correlations. But BclI showed no e effect on TGF-β 1 mRNA, N363S, and ER22/23EK had not been examined.Conclusions:
According to the results, there was a relationship between single nucleotide polymorphisms (SNPs) of the NR3C1 gene and TGF-β1 mRNA in asthmatic children. TthIII1 CC and CT genotype have the strongest induction effect on the expression of TGF-1. The phenomenon suggests that SNPs may be involved in the asthma pathology.Keywords
Childhood Asthma Glucocorticoid (GC) Glucocorticoid Receptor (GR) Single Nucleotide Polymorphism (SNP) TGF-β1
1. Background
Asthma is one of the most common diseases among children with a global prevalence of about 14% and an increasing incidence worldwide (1, 2). It is a disease with heterogeneity and complexity originating from an interplay of gene and environment, persisting and recurring inflammation of the respiratory tract, leading to tissue remodeling and impairing the lung function (3). The pathologic mechanisms of asthma are not completely known yet. Many polymorphisms and cytokines take part in the pathogenesis of inflammation in asthma, such as TGF-β, interleukins (IL-2, IL-4, IL-5, IL-9, IL-6, IL-10, IL-12, IL-13, and IL-17, IL-22, IL-25, IL-33, etc.) (4, 5). TGF-β has played an important role in growth, differentiation, cell migration, formation, and degradation of extracellular matrix components, chemotaxis courses, remodeling, and cell apoptosis in the bronchi. Experimental studies have shown that adenoviral mediated transgenic TGF-β1 in the rodent lung induced serious pulmonary fibrosis and caused the sedimentation of extracellular matrix (6). TGF-β is a multifunctional cytokine, which has both pro-inflammatory and anti-inflammatory effects on airway inflammation and immune response in asthma, and its fibrotic effect has an important role in airway remodeling in asthma (7, 8).
Glucocorticoid (GC) is widely used to treat many chronic conditions, such as asthma, skin diseases, Crohn’s disease, rheumatoid arthritis, and immune rejection after organ transplantation (9, 10). GC binds to its corresponding glucocorticoid receptor (GR) to form the GC-GR complex, that subsequently up-regulates the expression of anti-inflammatory proteins and cytokines (i.e., lipocortin-1, IΚB, MKP1, IL-10, IL-12, and IL-1 receptor antagonist) in the nucleus; meanwhile, it suppresses the expression of pro-inflammatory proteins in the cytoplasm (i.e., IL-2, IL-3, IL-4, IL-5, IL-6, TNF-α, IFN-γ, endothelin-1, and phospholipase A2).
GR is a nuclear receptor superfamily protein, encoded by NR3C1 gene. Studies suggest that mutations and polymorphisms of the NR3C1 gene contribute to a decreased response to GC for the treatment of asthma, leading to drug-resistance (11, 12). Four restriction fragment length polymorphisms (RFLP) (TthIII1, BclI, ER22/23EK, and N363S) may be involved in the pathogenesis of this phenomenon, given their role in the pathology of other diseases, such as metabolic syndrome, autoimmune diseases, and cardiovascular disease (13): TthIII1 coupled with ER22/23EK, is implicated in resistance to GC (11, 14); BclI couples with other two single nucleotide polymorphisms (SNPs, intron 33389 and intron 33388) to increase sensitivity to GC (15, 16); ER22/23EK deceased GC sensitivity (11, 17); the N363S influences the phosphorylation of GR that introduces the structural changes of GR and functional changes of AF1; it is also characterized by increased gene encoding for protein syntheses in response of cells to GC action (17, 18). Over the years, SNPs with altered response to GC therapy have been reported (18-20). TGF-β1 induces the proliferation and chemoattraction of fibroblasts, which results in airway remodeling. It also induces fibroblast to differentiate into myofibroblasts, promoting the production of ECM proteins, fibronectin, and collagen, which finally contributes to the contraction of the ECM (21). GC significantly inhibits the production of TGF-β1 by altering the expression of TGF-β1 mRNA (22, 23), and TGF-β1 induces GC resistance (24). These results imply that SNPs may be associated with TGF-β1, which may illuminate the potential pathological mechanism of children with asthma.
Therefore, we hypothesize that these polymorphisms contribute to the heterogeneity of treatment response given their role in mediating TGF-β1 and GC signaling.
2. Methods
2.1. Subjects
The samples of this study included 52 outpatients (age range: 6 - 14 years) with asthma referred to Huai’an First People’s Hospital, Nanjing Medical University from January 2018 to June 2019. Meanwhile, 40 age-matched healthy volunteers were included as the control group. All the recruited children conformed to the diagnostic guidelines of childhood asthma developed by the national cooperative group on Child Prevention and Treatment in 2016 (25) and The Global Initiative for Asthma (GINA) (2). There were 32 mild, 17 moderate, and three severe cases of asthmatic children who had been diagnosed for 2 - 3 years. The patients had not received any allergen specific immunotherapy, and they had three or more asthma attacks per year; onset was exacerbated at night or early morning. Of the 52 children, 32 were males, and 20 were females. All enrolled children were screened for allergens (serological allergen detection), including 10 non-sensitized, 16 mono-sensitized, and 26 multi-sensitized patients; the total serum of IgE of the allergic patients was above 0.2 IU/L. Meanwhile, 40 healthy children (25 males vs. 15 females) with an age range of 6 - 14 years were recruited and underwent a physical examination. The subjects were included if their immediate relatives across three generations did not have a history of asthma and other lung diseases, such as chronic obstructive pulmonary disease (COPD) and pulmonary fibrosis. There was no history of diabetes, nephritic syndrome, systemic lupus erythematosus (SLE), and psoriasis. Additionally, there was no history of long time use of GC and ephedrine treatment. The study was approved by the ethics committee of Huai’an First People’s Hospital, Nanjing Medical University (Ethical Code: YX-P-2020-002-01). All children or their guardians signed an informed consent.
2.2. Specimen Collection and DNA Extraction
Following the ethical considerations, 200 UL EDTA anticoagulant peripheral fasting blood was collected from both experimental and control groups. The QIAmp DNA Blood Mini Kit (QIAGEN, Germany) was used to extract DNA following manufacturer’s instructions (spin protocol). DNA samples were stored at -20°C.
2.3. Genotyping of TthIII1, BclI, ER22/23EK, N363S: PCR-RFLP Method
The genotyping of TthIII1, BclI, ER22/23EK, and N363S was carried out using primers sequence as previously described (14, 26). The forward primer for TthIII1 was 5’-TCC AGG AGT GGG ACA TAA AGC T-3’, and the reverse primer was 5’-CTT AGA AGC AGA GGT GGA AAT GAA G-3’. The forward primer for BcII was 5'-TGC TGC CTT ATT TGT AAA TTC GT-3', and the reverse primer was 5'-AAG CTT AAC AAT TTT GGC CAT C-3'. The forward primer for ER22/23EK was 5'-GAT TCG GAG TTA ACT AAA AG-3', and the reverse primer was 5'-ATC CCA GGT CAT TTC CCA TC-3'. The forward primer for N363S was 5'-AGT ACC TCT GGA GGA CAG AT-3' and the reverse primer was 5'-GTC CAT TCT TAA GAA ACA GG-3'. Polymerase chain reaction (PCR) was conducted according to the manufacturer’s instructions (Applied Biosystems, USA). The products were digested at 37°C for 4 hours with 1 UL restriction enzyme (Thermo Fisher Scientific, USA). The TthIII1 restriction enzyme, (PsyI) cutting site was 5’…G A C N↓ N N G T C…3’, 3’…C T G N N↑N C A G…5’. The BclI restriction enzyme (BclI) (Thermo Fisher scientific, USA) cutting site was 5’…T↓ G A T C A …3’,3’…A C T A G ↑T…5’. The ER22/23EK restriction enzyme (MnlI) cutting site was 5’…C C T C (N)7 ↓…3’,3’…G G A G (N)6↑ …5’. The N363S restriction enzyme (TasI) cutting site was 5’…↓ A A T T …3’, 3’…T T A A↑T…5’. The RFLP (restriction fragment length polymorphisms) products were isolated using 3% agarose gel electrophoresis, stained with ethidium bromide and observed by UV light. Hydrolyzed fragments of TthIII1 DNA were CT (96bp, 53bp, 43bp), TT (96bp), and CC (53bp, 43bp); DNA fragments of BclI were GC (335bp, 221bp, 117b), CC (221bp, 117bp), and GG (335bp); DNA fragments of ER22/23EK were GG (221bp, 117bp); and DNA fragments of N363S were AA (135bp, 95bp).
2.4. TGF-β1 mRNA Expression
The peripheral mononuclear cells were extracted by Hank’s method from 2 mL EDTA anticoagulant blood. The total RNA was extracted by TRIzol Reagent (Ambion, NY, USA), then 1000 ng RNA was converted to cDNA by reverse transcription using AccuScript PfuUltraII RT-PCR kit (Agilent Technologies, USA). Primers of TGF-β1 of real-time PCR were 5’-GGT ACC TGA ACC CGT GTT GCT-3’ and 5’-TGT TGC TGT ATT TCT GGT ACA GCT C-3’, and the primers of the loading control GAPDH were 5'-AGC CAC ATC GCT CAG ACA-3’ and 5’-GCC CAA TAC GAC CAA ATC C-3’. The amplification of cDNA was done by qRT-PCR kit (Stratagene, USA), using normal two steps: primer annealing temperature was 61°C and annealing time was 40 cycles of 20 seconds. PCR reaction used Agilent Technologies Stratagene Mx3000P. The RT-PCR amplification of the TGF-β1 and GAPDH gene for every sample, GAPDH as internal reference, the CT (threshold cycle) values were analyzed using Mx-Pro software. ΔCT values calculated using the formula: ΔCT = CT, GENE - CT, GAPDH.
2.5. Statistical Analysis
SPSS 26.0 software and GraphPad Prism version 8 were used for statistical analysis. The genotype distribution frequency and allele frequency of each point in each group were calculated and confirmed to be in line with the Hardy-Weinberg equilibrium (P > 0.05). The comparison of alleles and genotypes between groups was performed by analysis of variance (ANOVA), and P < 0.05 indicated a statistically significant difference. Correlation coefficients were analyzed by Spearman’s rank test.
3. Results
Table 1 indicates the frequencies of genotypes and particular alleles of four SNPs. We found no statistically significant differences in TthIII1 and Bcl1 between the asthmatic and control groups (P > 0.05); N363S and ER22/23EK polymorphisms were not detected between the two groups; also, the genotypes of N363S and ER22/23EK were AA and GG, respectively.
The Frequencies of Four SNPs (TthIII1, BclI, N363S, and ER22/23EK) of the NR3C1 Gene in Asthmatic Children and Control Group a
NR3C1 SNP | Asthma Group | Control Group | P |
---|---|---|---|
TthIII1 | 0.959 | ||
Genotype | |||
TT | 1 (1.92) | 1 (2.50) | |
CT | 7 (13.46) | 6 (15) | |
CC | 44 (84.62) | 33 (82.5) | |
Allele | |||
C | 95 (91.35) | 72 (90) | |
T | 9 (8.65) | 8 (10) | |
BclI | 0.964 | ||
Genotype | |||
CC | 2 (3.85) | 2 (5.0) | |
CG | 16 (30.77) | 12 (30) | |
GG | 34 (65.38) | 26 (65.0) | |
Allele | |||
C | 20 (19.23) | 16 (20) | |
G | 84 (80.77) | 64 (80) | |
ER22/23EK | |||
Genotype | |||
GG | 52 (100) | 40 (100) | |
Allele | |||
A | 0 (0) | 0 (0) | |
G | 104 (100) | 80 (100) | |
N363S | |||
Genotype | |||
AA | 52 (100) | 40 (100) | |
Allele | |||
A | 104 (100) | 80 (100) | |
G | 0 (0) | 0 (0) |
The expression levels of TGF-β1 mRNA are summarized in Table 2. Correlation analysis between the two SNPs and the expression levels of TGF-β1 mRNA were calculated by Spearman’s rank test. TthIII1 and TGF-β1 mRNA were significantly correlated [R2 = -0.25, P = 0.016, beta coefficient = -0.296, 95% CI -0.0147-0.073] (Figure 1). There was no statistically significant correlation between BclI and TGF-β1 mRNA [R2 = 0.063, P = 0.548; beta coefficient = 0.063, 95%CI = 0.036-0.019]. BclI and the level of TGF-β1 mRNA relationships are shown in Figure 2. ER22/23EK and N363S polymorphisms were not found between the groups.
The Expression Level of TGF-β1 mRNA in Asthmatic and Control Groups
Parameter | Control Group | Asthmatic Group | Control + Asthmatic Group |
---|---|---|---|
Mean ± SD dCT | -9.748 ± 3.268 | -7.459 ± 2.928 | -7.580 ± 4.319 |
Max. dCT | -4.3 | 9.1 | 9.1 |
Min. dCT | -18.3 | -14.2 | -18.3 |
CT and TT, CC and TT was statistically significant, CC and CT was not statistically significant
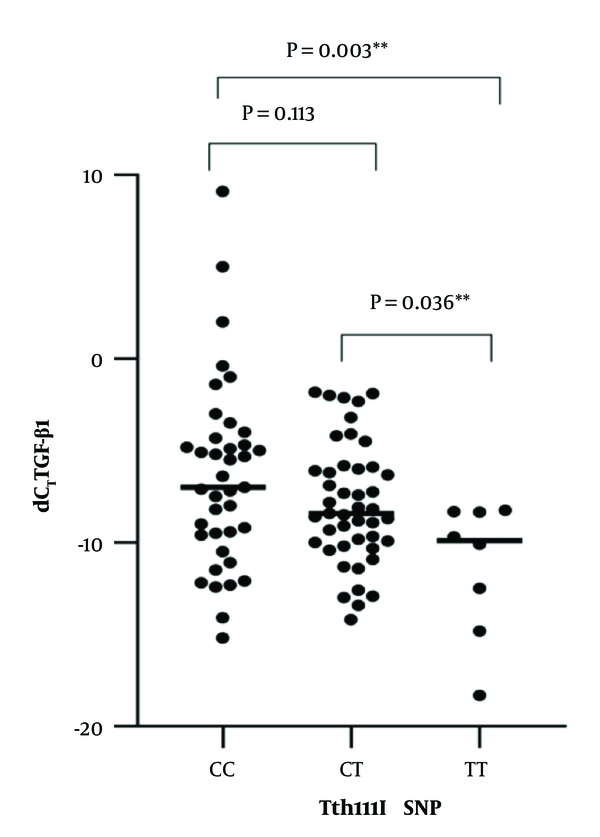
CC and GG, CC and GC, GC and GG was not statistically significant (*P < 0.05 indicated a statistically significant difference; **P < 0.01 showed a high difference.)
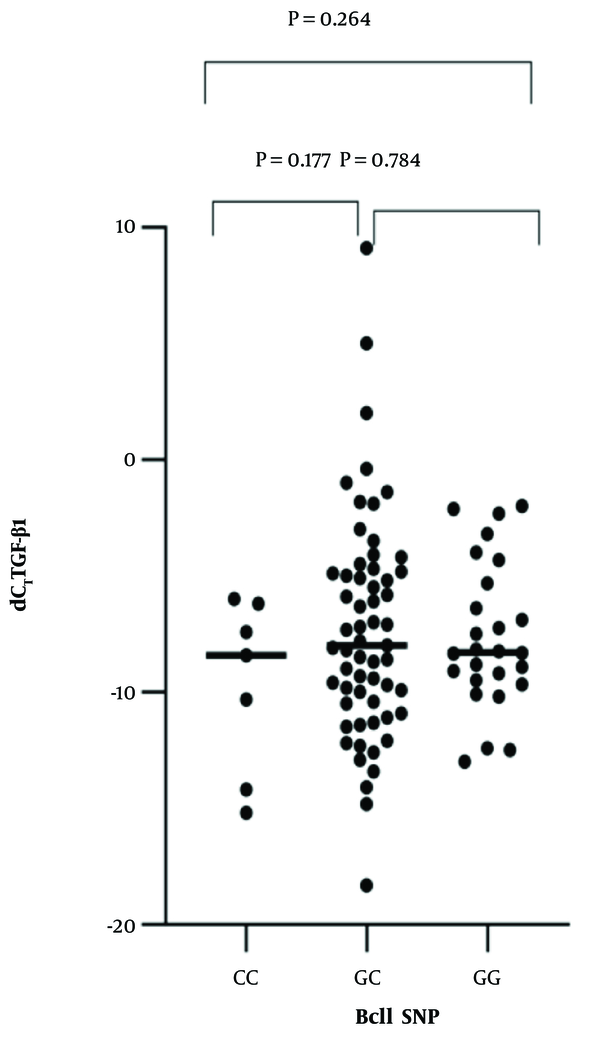
4. Discussion
Asthma causes airway hyperresponsiveness, recurrent wheezing, cough, and dyspnea. GC is a vital drug for the treatment of asthma. However, some children with asthma have reduced sensitivity or resistance to it. Studies found that GC resistance is related to the mutation of NR3C1, that changes the amino acid content of the receptor structure and determine the biological action or polymorphism (20, 27-29). Some studies found that glucocorticoid resistant patients have an increased cells expressing IL-2 and IL-4 mRNA compared with numbers seen in steroid-sensitive asthmatic patients in the bronchoalveolar lavage fluid. IL-2 and IL-4 activate P38MAPK kinase, JNK, and ERK, GR phosphorylates at Ser226, which reduces affinity with GC, resulting in reduced sensitivity or resistance to GC (30, 31).
Patients with resistance to GC are associated with epidermal growth factor (EGF). EGF has low expression of phosphorylated tyrosine of these patients, which leading to increasing inflammation and subsequent resistance (32). In asthmatic patients treated with GC, down-regulation of bispecific MAPK phosphatase expression (DUSP1) and loss of MAPK activity affect GC sensitivity (33).
In this study, we found that TthIII1 SNPs are significantly correlated with the expression level of TGF-β1. We hypothesize that TthIII1 SNPs increase the expression level of TGF-β1 and aggravate the inflammation of asthma. TthIII1 CC and CT genotype have the strongest induction effect on the expression of TGF-1. TthIII1 SNPs can significantly increase the expression of TGF-β1 in patients with GC treatment (24); therefore, TGF-β1 can induce and intensify airway remodeling and GC resistance. According to the present study, TthIII1 CC are the most important risk factors for tissue remodeling and GC resistance.
N363 facilitates the action of the GC-GR complex, which improves GC sensitivity (34, 35). It has also been reported that codon N363S AG or GG is related to the enhancement of the anti-inflammatory effect of GC treatment and reduces the risk of out-of-control asthma (28, 29, 34). However, we did not observe this issue in our study, because we did not detect the polymorphism of N363S or racial difference.
Our study found that TthIII1 can induce an increase in the expression level of TGF-β1 mRNA. This causes a progressive asthma course and airway obstruction, leading to the reduction of lung function and poor clinical prognosis. It is thought that TGF-β1 plays a crucial part in the epithelial-mesenchymal transformation (EMT) mechanism, which results in an increase in the number of subepithelial mesenchymal cells, therefore adding to the number of contractile cell and airway hyperreactivity (36). In the course of EMT, epithelial cells lose their classic connections and polarity between cells and acquire a more mesenchymal phenotype. A variety of cytokines, such as interleukins, reinforce MT in respiratory epithelial cells in a TGF-β1 dependent manner, which promotes airway remodeling in patients with asthma. EMT contributes to increasing airway smooth muscle cells in the lung process and elevated TGF-β signaling, this mechanism which has been confirmed by many experiments of animal models of asthma (36).
4.1. Conclusions
The results of this study showed the significant effect of TthIII1 SNPs of NR3C1 polymorphism on increased inflammation in asthmatic children, inducing the expression level of TGF-β1 mRNA. CC and CT of Tth111I are important high-risk factors enhancing the expression level of TGF-β1; therefore, they can result in EMT, contributing to undesirable bronchial remodeling and promotion of airway hyperresponsiveness. The ways to reduce the expression of TGF-β1 and mitigate the degree of airway remodeling have certain implications in the future diagnosis and treatment of bronchial airway asthma. However, the mechanism of bronchial airway remodeling and the interconnection of other growth factors and airway remodeling needs to be further studied. Clinical early intervention in the process of airway remodeling can avoid irreversible airway restriction or inhibit its further development to improve the quality of life of children with asthma.
References
-
1.
Zar HJ, Ferkol TW. The global burden of respiratory disease-impact on child health. Pediatr Pulmonol. 2014;49(5):430-4. [PubMed ID: 24610581]. https://doi.org/10.1002/ppul.23030.
-
2.
Global Initiative for Asthma. Global Strategy for Asthma Management and Prevention. Fontana, CA, USA: Global Initiative for Asthma; 2021.
-
3.
Fuchs O, Bahmer T, Rabe KF, von Mutius E. Asthma transition from childhood into adulthood. Lancet Respir Med. 2017;5(3):224-34. [PubMed ID: 27666650]. https://doi.org/10.1016/S2213-2600(16)30187-4.
-
4.
Ito T, Hirose K, Nakajima H. Bidirectional roles of IL-22 in the pathogenesis of allergic airway inflammation. Allergol Int. 2019;68(1):4-8. [PubMed ID: 30424940]. https://doi.org/10.1016/j.alit.2018.10.002.
-
5.
Gibeon D, Menzies-Gow AN. Targeting interleukins to treat severe asthma. Expert Rev Respir Med. 2012;6(4):423-39. [PubMed ID: 22971067]. https://doi.org/10.1586/ers.12.38.
-
6.
McMillan SJ, Xanthou G, Lloyd CM. Manipulation of allergen-induced airway remodeling by treatment with anti-TGF-beta antibody: effect on the Smad signaling pathway. J Immunol. 2005;174(9):5774-80. [PubMed ID: 15843580]. https://doi.org/10.4049/jimmunol.174.9.5774.
-
7.
Rider CC, Mulloy B. Heparin, Heparan Sulphate and the TGF-beta Cytokine Superfamily. Molecules. 2017;22(5). [PubMed ID: 28468283]. [PubMed Central ID: PMC6154108]. https://doi.org/10.3390/molecules22050713.
-
8.
Saito A, Horie M, Nagase T. TGF-beta Signaling in Lung Health and Disease. Int J Mol Sci. 2018;19(8). [PubMed ID: 30127261]. [PubMed Central ID: PMC6121238]. https://doi.org/10.3390/ijms19082460.
-
9.
Buttgereit F, Burmester GR, Lipworth BJ. Optimised glucocorticoid therapy: the sharpening of an old spear. Lancet. 2005;365(9461):801-3. [PubMed ID: 15733723]. https://doi.org/10.1016/S0140-6736(05)17989-6.
-
10.
Liu D, Ahmet A, Ward L, Krishnamoorthy P, Mandelcorn ED, Leigh R, et al. A practical guide to the monitoring and management of the complications of systemic corticosteroid therapy. Allergy Asthma Clin Immunol. 2013;9(1):30. [PubMed ID: 23947590]. [PubMed Central ID: PMC3765115]. https://doi.org/10.1186/1710-1492-9-30.
-
11.
Nicolaides NC, Galata Z, Kino T, Chrousos GP, Charmandari E. The human glucocorticoid receptor: molecular basis of biologic function. Steroids. 2010;75(1):1-12. [PubMed ID: 19818358]. [PubMed Central ID: PMC2813911]. https://doi.org/10.1016/j.steroids.2009.09.002.
-
12.
Vandewalle J, Luypaert A, De Bosscher K, Libert C. Therapeutic Mechanisms of Glucocorticoids. Trends Endocrinol Metab. 2018;29(1):42-54. [PubMed ID: 29162310]. https://doi.org/10.1016/j.tem.2017.10.010.
-
13.
Manenschijn L, van den Akker EL, Lamberts SW, van Rossum EF. Clinical features associated with glucocorticoid receptor polymorphisms. An overview. Ann N Y Acad Sci. 2009;1179:179-98. [PubMed ID: 19906240]. https://doi.org/10.1111/j.1749-6632.2009.05013.x.
-
14.
Tissing WJ, Meijerink JP, den Boer ML, Brinkhof B, van Rossum EF, van Wering ER, et al. Genetic variations in the glucocorticoid receptor gene are not related to glucocorticoid resistance in childhood acute lymphoblastic leukemia. Clin Cancer Res. 2005;11(16):6050-6. [PubMed ID: 16115950]. https://doi.org/10.1158/1078-0432.CCR-04-2097.
-
15.
Pietras T, Panek M, Tworek D, Oszajca K, Wujcik R, Gorski P, et al. The Bcl I single nucleotide polymorphism of the human glucocorticoid receptor gene h-GR/NR3C1 promoter in patients with bronchial asthma: pilot study. Mol Biol Rep. 2011;38(6):3953-8. [PubMed ID: 21113676]. [PubMed Central ID: PMC3115143]. https://doi.org/10.1007/s11033-010-0512-5.
-
16.
Stevens A, Ray DW, Zeggini E, John S, Richards HL, Griffiths CE, et al. Glucocorticoid sensitivity is determined by a specific glucocorticoid receptor haplotype. J Clin Endocrinol Metab. 2004;89(2):892-7. [PubMed ID: 14764810]. https://doi.org/10.1210/jc.2003-031235.
-
17.
Muller LM, Kienitz T, Deutschbein T, Riester A, Hahner S, Burger-Stritt S, et al. Glucocorticoid Receptor Polymorphisms Influence Muscle Strength in Cushing's Syndrome. J Clin Endocrinol Metab. 2020;105(1). [PubMed ID: 31613324]. https://doi.org/10.1210/clinem/dgz052.
-
18.
Panek M, Pietras T, Antczak A, Gorski P, Kuna P, Szemraj J. The role of functional single nucleotide polymorphisms of the human glucocorticoid receptor gene NR3C1 in Polish patients with bronchial asthma. Mol Biol Rep. 2012;39(4):4749-57. [PubMed ID: 22015776]. [PubMed Central ID: PMC3294211]. https://doi.org/10.1007/s11033-011-1267-3.
-
19.
Thomson NC. Addressing corticosteroid insensitivity in adults with asthma. Expert Rev Respir Med. 2016;10(2):137-56. [PubMed ID: 26678267]. https://doi.org/10.1586/17476348.2016.1133304.
-
20.
Mohamed NA, Abdel-Rehim AS, Farres MN, Muhammed HS. Influence of glucocorticoid receptor gene NR3C1 646 C>G polymorphism on glucocorticoid resistance in asthmatics: a preliminary study. Cent Eur J Immunol. 2015;40(3):325-30. [PubMed ID: 26648776]. [PubMed Central ID: PMC4655382]. https://doi.org/10.5114/ceji.2015.54594.
-
21.
Tian X, Tian X, Huo R, Chang Q, Zheng G, Du Y, et al. Bacillus Calmette-Guerin alleviates airway inflammation and remodeling by preventing TGF-beta1 induced epithelial-mesenchymal transition. Hum Vaccin Immunother. 2017;13(8):1758-64. [PubMed ID: 28441064]. [PubMed Central ID: PMC5557244]. https://doi.org/10.1080/21645515.2017.1313366.
-
22.
De Iudicibus S, Franca R, Martelossi S, Ventura A, Decorti G. Molecular mechanism of glucocorticoid resistance in inflammatory bowel disease. World J Gastroenterol. 2011;17(9):1095-108. [PubMed ID: 21448414]. [PubMed Central ID: PMC3063901]. https://doi.org/10.3748/wjg.v17.i9.1095.
-
23.
Tang X, Nian H, Li X, Yang Y, Wang X, Xu L, et al. Effects of the combined extracts of Herba Epimedii and Fructus Ligustrilucidi on airway remodeling in the asthmatic rats with the treatment of budesonide. BMC Complement Altern Med. 2017;17(1):380. [PubMed ID: 28764781]. [PubMed Central ID: PMC5540498]. https://doi.org/10.1186/s12906-017-1891-0.
-
24.
Li M, Keenan CR, Lopez-Campos G, Mangum JE, Chen Q, Prodanovic D, et al. A Non-canonical Pathway with Potential for Safer Modulation of Transforming Growth Factor-beta1 in Steroid-Resistant Airway Diseases. iScience. 2019;12:232-46. [PubMed ID: 30711747]. [PubMed Central ID: PMC6360516]. https://doi.org/10.1016/j.isci.2019.01.023.
-
25.
Hong J, Bao Y, Chen A, Li C, Xiang L, Liu C, et al. Chinese guidelines for childhood asthma 2016: Major updates, recommendations and key regional data. J Asthma. 2018;55(10):1138-46. [PubMed ID: 29227721]. https://doi.org/10.1080/02770903.2017.1396474.
-
26.
Kaya Z, Caglayan S, Akkiprik M, Aral C, Ozisik G, Ozata M, et al. Impact of glucocorticoid receptor gene (NR3C1) polymorphisms in Turkish patients with metabolic syndrome. J Endocrinol Invest. 2016;39(5):557-66. [PubMed ID: 26596278]. https://doi.org/10.1007/s40618-015-0409-1.
-
27.
Panek M, Pietras T, Antczak A, Fabijan A, Przemecka M, Gorski P, et al. The N363S and I559N single nucleotide polymorphisms of the h-GR/NR3C1 gene in patients with bronchial asthma. Int J Mol Med. 2012;30(1):142-50. [PubMed ID: 22469783]. https://doi.org/10.3892/ijmm.2012.956.
-
28.
Prystupa LN, Garbuzova VY, Kmyta VV. [Bcl1 Polymorphism of Glucocorticoid Receptor Gene and Respiratory Diseases]. Lik Sprava. 2015;(1-2):43-8. Ukrainian. [PubMed ID: 26118026].
-
29.
Sarro-Ramirez A, Sanchez D, Tejeda-Padron A, Buenfil-Canto LV, Valladares-Garcia J, Pacheco-Pantoja E, et al. Characterization of Mitogen-Activated Protein Kinase Expression in Nucleus Accumbens and Hippocampus of Rats Subjected to Food Selection in the Cafeteria Diet Protocol. CNS Neurol Disord Drug Targets. 2016;15(7):866-72. [PubMed ID: 27071784]. https://doi.org/10.2174/1871527315666160413161200.
-
30.
Dejager L, Vandevyver S, Petta I, Libert C. Dominance of the strongest: inflammatory cytokines versus glucocorticoids. Cytokine Growth Factor Rev. 2014;25(1):21-33. [PubMed ID: 24412262]. https://doi.org/10.1016/j.cytogfr.2013.12.006.
-
31.
Irusen E, Matthews JG, Takahashi A, Barnes PJ, Chung KF, Adcock IM. p38 Mitogen-activated protein kinase-induced glucocorticoid receptor phosphorylation reduces its activity: role in steroid-insensitive asthma. J Allergy Clin Immunol. 2002;109(4):649-57. [PubMed ID: 11941315]. https://doi.org/10.1067/mai.2002.122465.
-
32.
Hamilton LM, Puddicombe SM, Dearman RJ, Kimber I, Sandstrom T, Wallin A, et al. Altered protein tyrosine phosphorylation in asthmatic bronchial epithelium. Eur Respir J. 2005;25(6):978-85. [PubMed ID: 15929951]. https://doi.org/10.1183/09031936.05.00098604.
-
33.
Shah S, King EM, Mostafa MM, Altonsy MO, Newton R. DUSP1 Maintains IRF1 and Leads to Increased Expression of IRF1-dependent Genes: A MECHANISM PROMOTING GLUCOCORTICOID INSENSITIVITY. J Biol Chem. 2016;291(41):21802-16. [PubMed ID: 27551049]. [PubMed Central ID: PMC5076847]. https://doi.org/10.1074/jbc.M116.728964.
-
34.
Roerink SH, Wagenmakers MA, Smit JW, van Rossum EF, Netea-Maier RT, Plantinga TS, et al. Glucocorticoid receptor polymorphisms modulate cardiometabolic risk factors in patients in long-term remission of Cushing's syndrome. Endocrine. 2016;53(1):63-70. [PubMed ID: 26873309]. [PubMed Central ID: PMC4901092]. https://doi.org/10.1007/s12020-016-0883-z.
-
35.
van den Akker EL, Russcher H, van Rossum EF, Brinkmann AO, de Jong FH, Hokken A, et al. Glucocorticoid receptor polymorphism affects transrepression but not transactivation. J Clin Endocrinol Metab. 2006;91(7):2800-3. [PubMed ID: 16684836]. https://doi.org/10.1210/jc.2005-2119.
-
36.
Johnson JR, Nishioka M, Chakir J, Risse PA, Almaghlouth I, Bazarbashi AN, et al. IL-22 contributes to TGF-beta1-mediated epithelial-mesenchymal transition in asthmatic bronchial epithelial cells. Respir Res. 2013;14:118. [PubMed ID: 24283210]. [PubMed Central ID: PMC4176096]. https://doi.org/10.1186/1465-9921-14-118.