Abstract
Context:
Functional neurological disorder (FND) is common in neurology outpatient clinics, affecting approximately 6% of neurology patients. Functional neurological disorder is characterized by motor and sensory symptoms that cannot be explained by organic or structural neurological issues. These symptoms often mimic those of recognized neurological conditions, making diagnosis and treatment complex.Evidence Acquisition:
This study presents a comprehensive voxel-wise meta-analysis of structural brain changes in FND to explore potential quantitative measurements that could serve as biomarkers for different FND types. In this investigation, we systematically queried prominent academic databases using specific keywords: "FND", "somatization disorder", and "voxel-based morphometry (VBM) ". Additionally, we employed the BrainMap database to identify VBM data pertaining to FND. Our meta-analysis, performed using ES-SDM software, examined grey matter (GM) changes in FND patients. We also assessed the robustness of the results via Jackknife analysis and investigated unexplained variability using heterogeneity analysis.Results:
Our meta-analysis revealed both positive and negative GM volumetric changes in different brain areas across various FND types. The robustness of the results was confirmed, and the assessment of bias and publication bias yielded no significant concerns.Conclusions:
These findings offer valuable insights into the neuroanatomical basis of diverse functional disorders, providing potential biomarkers. Moreover, the identified regions are associated with pain modulation, emotional processing, and cognitive functions, contributing to a better understanding of FND pathophysiology.Keywords
Functional Neurological Disorder Voxel-Based Morphometry Structural Brain Changes Biomarkers
1. Context
Functional neurological disorder (FND) is one of the most common diagnoses in neurology outpatient clinics, constituting roughly 6% of neurology contacts and having community incidence rates of 4012 per 100,000 per year (1). Functional neurological disorder exhibits a higher prevalence in women, with a peak incidence between the ages of 35 - 50, but it is not rare in men or throughout their lifespan (1). The FND is clinically characterized by motor or sensory symptoms, including limb weakness, dystonia, tremor, numbness, pain, or seizures. According to the DSM-5 diagnostic criteria for FND, a diagnosis requires the presence of one or more symptoms indicating an alteration in voluntary or sensory function. Clinical assessments must also reveal incompatibility between these symptoms and recognized neurological or medical conditions (2). Compared to other neurological disorders, FND is associated with similar levels of disability and impaired physical and mental quality of life (3). The prognosis for FND is typically challenging, with up to 40% of patients reporting outcomes that are either similar to or worse than their initial condition during a recent review with a 7-year follow-up (4). Traditionally, the diagnosis of FND relied on the exclusion of organic causes for the symptoms. However, recent evidence has provided new insights into FND's pathophysiology, facilitating a more comprehensive understanding and the identification of potential biomarkers (5). In this study, a voxel-wise meta-analysis was performed to investigate structural brain changes in FNDs. Our aim was to determine whether specific quantitative measurements could serve as potential biomarkers distinguishing between various types of FNDs.
2. Evidence Acquisition
We performed a comprehensive search of several online databases—Web of Science, PubMed, Cochrane, and Scopus—using a combination of keywords: ["functional neurological disorder" OR "FND" OR "somatization disorder" OR "SD"] and ["morphometry" OR "voxel-based" OR "VBM"] (Figure 1). Additionally, we searched the BrainMap database for relevant voxel-based morphometry (VBM) data related to FND.
PRISMA 2020 flowchart for recent systematic reviews involving searches in databases and registers only (6); * if feasible, consider reporting the number of records identified from each individual database or register searched, rather than presenting a cumulative total across all databases or registers; ** if automation tools were employed in the screening process, specify the number of records excluded by human reviewers and the number excluded by the automation tools. To find more information, please visit the following: http://www.prisma-statement.org/.
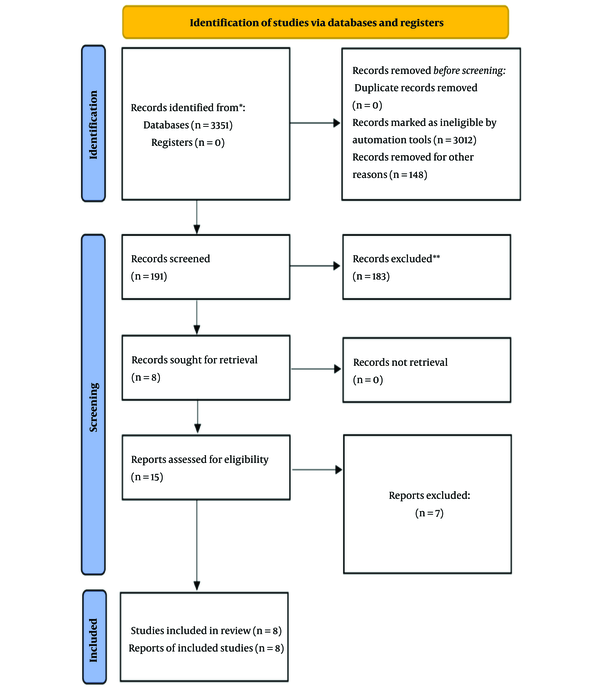
Our inclusion criteria required studies to compare grey matter (GM) changes between FND patients and control groups. Included studies had to provide coordinates for these comparisons using either Talairach or Montreal neurological institute (MNI) space. Studies were excluded if they lacked a control group or did not provide detailed coordinates.
For each included study, we extracted data on participant numbers, FND type, disease duration, any additional reported symptoms, and the coordinates of significant findings.
We used the ES-SDM software (version 6.11) for the meta-analysis. This software creates an effect-size map showing regional volumetric changes for each study using a random-effects model. It accounts for factors such as sample size, intra-study variability, and between-study heterogeneity when calculating the weight of each study. We used the ES-SDM software (version 6.11) for the meta-analysis. The primary effect size of interest was the standardized mean difference (SMD). The SMD quantifies the magnitude of differences in GM volume between FND patients and control groups, expressed in standard deviation units. This effect size facilitates comparison across studies with varying scales of measurement and sample sizes.
We set our statistical threshold at P < 0.005 and used a full width at half-maximum (FWHM) of 20 mm. These parameters were chosen based on prior research to balance sensitivity and specificity while controlling false positives.
To assess the robustness of our results, we conducted a Jackknife analysis, systematically excluding one study at a time to ensure the stability of our findings. Additionally, we performed a heterogeneity analysis using a random-effects model and Q statistics to identify and explore variability among the results from different studies (7, 8).
3. Results
3.1. Summary of the Findings
Valet et al. (9) found significant reductions in GM in the cingulate, prefrontal, and insular cortex in 14 women with pain disorders compared to 25 healthy controls. Riederer et al. (10) observed increases in GM in the right primary sensory cortex, thalamus, bilateral temporal regions, and hippocampus in 23 patients with non-dermatomal sensory deficits versus 29 controls. In a separate study, they reported reduced left anterior insula volume in 26 FND patients (11). Perez et al. (12) identified decreased GM in the right anterior hippocampus in 22 FND patients versus 27 controls. Kozlowska et al. (13) found increased GM in the left supplementary motor area, right superior temporal gyrus, and dorsomedial prefrontal cortex in 25 FND patients compared to 24 controls. Aybek et al. (14) reported increased cortical thickness in the bilateral premotor cortex in 15 motor conversion disorder patients but found no correlation with symptom severity or duration. Maurer et al. (15) observed increased GM volumes in the left striatum, fusiform gyrus, amygdala, cerebellum, and bilateral thalamus in 48 functional movement disorder patients, while the left sensorimotor cortex showed decreased volume, with no correlation to disease duration or severity.
3.2. Regional Differences in Grey Matter Volume
3.2.1. Positive Peaks
Patients with FND demonstrated an increase in regional GM in the following areas (Figures 2 and 3).
Asymmetrical funnel plots illustration: Increase regional grey matter (GM) A, cerebellar vermis lobules IV and V; B, right postcentral gyrus; C, left supplementary motor area; D, thalamus.
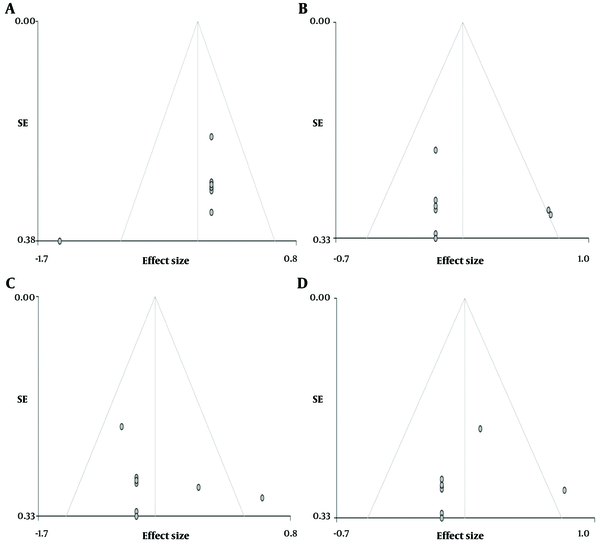
Our meta-analysis identified several brain regions with significant volumetric changes in grey matter (GM) between patients with functional neurological disorder (FND) and healthy controls. The results are categorized into regions exhibiting increased or decreased GM volume.
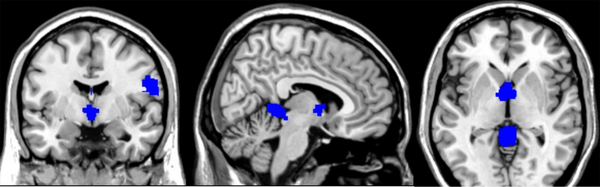
- Cerebellar vermis lobules IV and V (SMD-Z = 1.653, P < 0.0001, number of voxels = 485, MNI coordinates: 0, -46, -2).
- Right postcentral gyrus (SMD-Z = 1.486, P < 0.0005, number of voxels = 464, MNI coordinates: 60, 0, 18).
- Left supplementary motor area (SMD-Z = 1.134, P < 0.004, number of voxels = 14, MNI coordinates: 0, -16, 56).
- Thalamus (SMD-Z = 1.552, P < 0.0003, number of voxels = 452, MNI coordinates: -2, 0, 6).
3.2.2. Negative Peaks
The voxel-wise meta-analysis revealed a significant reduction in the right inferior longitudinal fasciculus (SMD-Z = -1.488, P < 0.001, number of voxels = 86, MNI coordinates: 42, -12, -12) in patients diagnosed with FND compared to healthy control participants (Figure 4).
Illustration of asymmetrical funnel plot: Decrease in right inferior longitudinal Fasciculus in functional neurological disorder (FND) patients vs. healthy controls
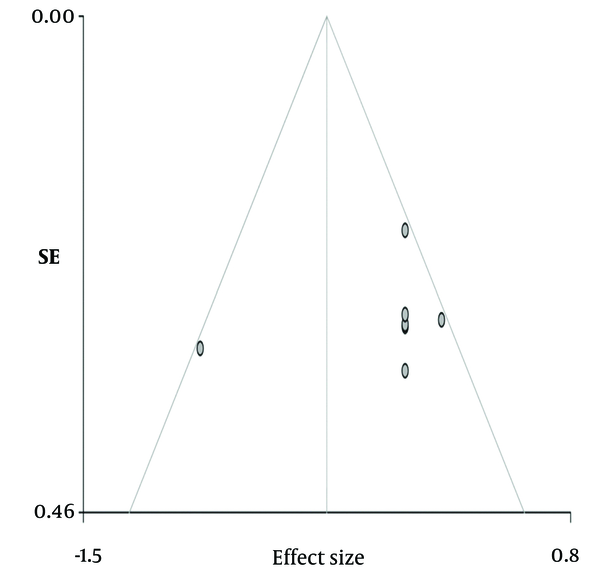
3.3. Heterogeneity and Risk of Bias
Although the funnel plots, when visually inspected, may give the impression of publication bias, Egger's test results showed no significant publication bias for each specific peak location (Figure 5).
Assessment of bias risk using the cochrane risk-of-bias tool: A, Bias-risk per study; B, bias-risk per domain summary
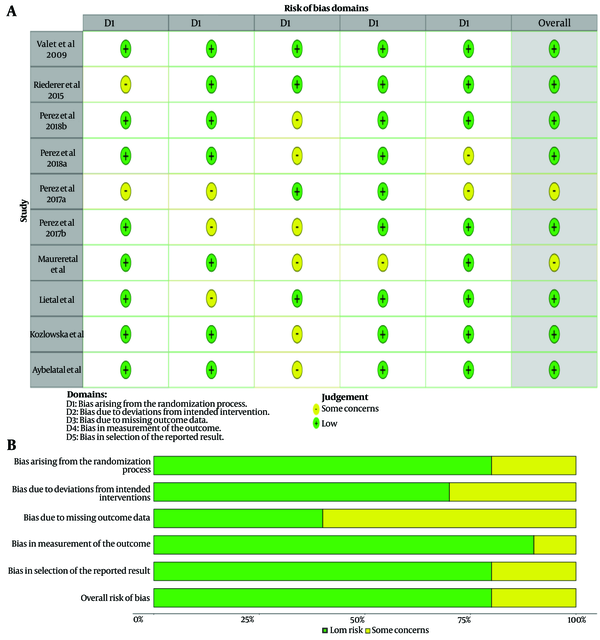
3.4. Jackknife Analysis
The Jackknife analysis confirmed the reliability of the results, as they were replicable in 8 out of 8 studies.
3.5. Grey Matter Changes in Functional Movement Disorders
3.5.1. Positive Peaks
Increased GM in cerebellar vermis lobules IV/V (MNI: 0, -42, 0).
3.5.2. Negative Peaks
Reduced GM was observed in the left cingulate/paracingulate gyri (MNI: 0, 0, 40).
3.6. Grey Matter Changes in Functional Neurological Disorder with Sensory Symptoms
3.6.1. Positive Peaks
Increased GM was observed in the left supplementary motor area (MNI: 0, -10, 58).
3.6.2. Negative Peaks
Decreased GM was observed in the left insula (MNI: -42, 14, -2).
3.7. Grey Matter Changes in Functional Neurological Disorder with Chronic Pain
3.7.1. Positive Peaks
Increased GM was observed in the right anterior thalamic area (MNI: 10, 4, 0), right middle frontal gyrus (MNI: 32, 0, 56), and left cerebellum (MNI: -6, -48, 4).
3.7.2. Negative Peaks
No significant reductions.
4. Conclusions
Our study represents the inaugural voxel-wise meta-analysis investigating volumetric changes in FNDs. Unfortunately, the data available so far are relatively limited. However, the present study has shown important findings. Functional neurological disorders appear to impact specific brain regions, including the cerebellar hemispheres and cerebellar vermis, the right postcentral gyrus, the left supplementary motor area, the thalamus, the right longitudinal fasciculus, the median cingulate gyrus, the insula of Reil, and the inferior and middle frontal gyri.
Patients with FND, particularly those presenting with predominant sensory symptoms, exhibited statistically significant findings, including a reduction in GM volume in the left insula and an augmentation in GM volume in the left supplementary motor cortex.
The insula and cingulate cortex are regions involved in emotional processing and are also thought to be connected with stress-mediated neuroplasticity (11, 16, 17). The insula functions with other brain areas, including the ventrolateral prefrontal cortex and the anterior cingulate cortex (18, 19). It plays a pivotal role in both cognitive and emotional domains, serving as a receiver and interpreter of emotions within the context of cognitive and sensory-motor information (20).
The posterior insula processes input from the spinothalamic tract and contributes to the initial response to nociceptive (painful) stimuli (21). The prefrontal cortex and insular cortex are actively engaged in pain processing and can further amplify and exacerbate the pain experience in the context of mood and emotional state (22, 23). Perez et al. (24) demonstrated a connection between the volume of the left insular cortex and the increase in symptom severity, particularly in the context of childhood abuse, among women with FND. Neurons in the supplementary motor area (SMA) respond to tactile, auditory, and visual stimuli when these stimuli are used as signals to initiate a movement or a series of movements. The SMA exhibits modulated activity and alterations in functional connectivity with the parahippocampal area during emotional contexts (25). Moreover, it appears to generate an efferent signal that enhances somatosensory processes, regardless of the number of sensory inputs reaching the somatosensory areas (25, 26). The SMA also plays a crucial role in timing sensory and motor tasks (27).
Overall analysis and the comparison between FMD patients and controls showed significantly increased GM volume in the lobules IV and V of the cerebellar vermis and a decrease in the cingulate gyrus volume. The cerebellum, especially the posterior lobe of the cerebellar hemispheres and the midline vermis, are implicated in modulating cognitive and affective functions. These regions are related to aversive responses and negative emotional processing (28).
Previous studies have also shown structural and functional abnormalities in the cerebellum in FND patients (29, 30). Perez et al. (12) also reported reduced left anterior cingulate cortex thickness in patients with FMD and high somatoform dissociation scores compared to controls.
Significant differences in GM volume were also observed in the primary somatosensory cortex (postcentral gyrus) and thalamus. Patients experiencing chronic pain displayed an increase in GM volume in the right anterior thalamic area, the right middle frontal gyrus, and the left cerebellar hemispheres.
The thalamus is a critical relay station in pain pathways. Nociceptive inputs are transmitted from the spinal cord to the dorsal thalamus, either directly via the spinothalamic tract or indirectly through pathways like the spinoreticular, spinomesencephalic, or medial lemniscal pathways to the thalamus (31). Both the left and right lobules VI and VIIb of the cerebellar hemispheres can exhibit overlapping activity during pain and motor processing. Functional connectivity analyses revealed significant correlations between multimodal cerebellar regions and sensorimotor regions within the cerebrum, including the supplementary motor area, anterior midcingulate cortex, and thalamus. Coombes et al. (32) proposed that the posterior cerebellum may play a crucial role in understanding pain-related adjustments in motor control. Egloff et al. (33) reported hypometabolism in the somatosensory cortex, thalamus, and lateral postcentral regions in patients with non-dermatomal somatosensory deficits. They also documented reduced activation in the contralateral primary sensory cortex, thalamus, and basal ganglia in patients with a non-dermatomal somatosensory deficit (33-35). Notably, this diminished activation was observed to be reversible following symptom recovery, coinciding with an increase in GM within the postcentral gyrus and thalamus (33-35).
Different FNDs are characterized by changes in GM volumes in different brain areas.
The GM changes found in the current meta-analysis are consistent with the outcomes of previous functional neuroimaging studies. These changes could represent the structural background to functional impairment that causes the symptoms in FNDs, but they may also indicate compensatory changes.
References
-
1.
Carson A, Lehn A. Epidemiology. Handb Clin Neurol. 2016;139:47-60. [PubMed ID: 27719864]. https://doi.org/10.1016/B978-0-12-801772-2.00005-9.
-
2.
American Psychiatric Association. Diagnostic and Statistical Manual of Mental Disorders. Washington, USA: American Psychiatric Publishing; 2013.
-
3.
Anderson KE, Gruber-Baldini AL, Vaughan CG, Reich SG, Fishman PS, Weiner WJ, et al. Impact of psychogenic movement disorders versus Parkinson's on disability, quality of life, and psychopathology. Mov Disord. 2007;22(15):2204-9. [PubMed ID: 17876850]. https://doi.org/10.1002/mds.21687.
-
4.
Gelauff J, Stone J, Edwards M, Carson A. The prognosis of functional (psychogenic) motor symptoms: a systematic review. J Neurol Neurosurg Psychiatry. 2014;85(2):220-6. [PubMed ID: 24029543]. https://doi.org/10.1136/jnnp-2013-305321.
-
5.
Thomsen BLC, Teodoro T, Edwards MJ. Biomarkers in functional movement disorders: a systematic review. J Neurol Neurosurg Psychiatry. 2020;91(12):1261-9. [PubMed ID: 33087421]. https://doi.org/10.1136/jnnp-2020-323141.
-
6.
Page MJ, McKenzie JE, Bossuyt PM, Boutron I, Hoffmann TC, Mulrow CD, et al. The PRISMA 2020 statement: an updated guideline for reporting systematic reviews. BMJ. 2021;372:n71. [PubMed ID: 33782057]. [PubMed Central ID: PMC8005924]. https://doi.org/10.1136/bmj.n71.
-
7.
Radua J, Mataix-Cols D, Phillips ML, El-Hage W, Kronhaus DM, Cardoner N, et al. A new meta-analytic method for neuroimaging studies that combines reported peak coordinates and statistical parametric maps. Eur Psychiatry. 2012;27(8):605-11. [PubMed ID: 21658917]. https://doi.org/10.1016/j.eurpsy.2011.04.001.
-
8.
Lim L, Radua J, Rubia K. Gray matter abnormalities in childhood maltreatment: a voxel-wise meta-analysis. Am J Psychiatry. 2014;171(8):854-63. [PubMed ID: 24781447]. https://doi.org/10.1176/appi.ajp.2014.13101427.
-
9.
Valet M, Gundel H, Sprenger T, Sorg C, Muhlau M, Zimmer C, et al. Patients with pain disorder show gray-matter loss in pain-processing structures: a voxel-based morphometric study. Psychosom Med. 2009;71(1):49-56. [PubMed ID: 19073757]. https://doi.org/10.1097/PSY.0b013e31818d1e02.
-
10.
Riederer F, Landmann G, Gantenbein AR, Stockinger L, Egloff N, Sprott H, et al. Nondermatomal somatosensory deficits in chronic pain are associated with cerebral grey matter changes. World J Biol Psychiatry. 2017;18(3):227-38. [PubMed ID: 26492569]. https://doi.org/10.3109/15622975.2015.1073356.
-
11.
Perez DL, Matin N, Barsky A, Costumero-Ramos V, Makaretz SJ, Young SS, et al. Cingulo-insular structural alterations associated with psychogenic symptoms, childhood abuse and PTSD in functional neurological disorders. J Neurol Neurosurg Psychiatry. 2017;88(6):491-7. [PubMed ID: 28416565]. [PubMed Central ID: PMC5497745]. https://doi.org/10.1136/jnnp-2016-314998.
-
12.
Perez DL, Williams B, Matin N, Mello J, Dickerson BC, LaFrance WC, et al. Anterior hippocampal grey matter predicts mental health outcome in functional neurological disorders: an exploratory pilot study. J Neurol Neurosurg Psychiatry. 2018;89(11):1221-4. [PubMed ID: 29326291]. [PubMed Central ID: PMC6041189]. https://doi.org/10.1136/jnnp-2017-317305.
-
13.
Kozlowska K, Griffiths KR, Foster SL, Linton J, Williams LM, Korgaonkar MS. Grey matter abnormalities in children and adolescents with functional neurological symptom disorder. Neuroimage Clin. 2017;15:306-14. [PubMed ID: 28560155]. [PubMed Central ID: PMC5440356]. https://doi.org/10.1016/j.nicl.2017.04.028.
-
14.
Aybek S, Nicholson TR, Draganski B, Daly E, Murphy DG, David AS, et al. Grey matter changes in motor conversion disorder. J Neurol Neurosurg Psychiatry. 2014;85(2):236-8. [PubMed ID: 23236016]. https://doi.org/10.1136/jnnp-2012-304158.
-
15.
Maurer CW, LaFaver K, Limachia GS, Capitan G, Ameli R, Sinclair S, et al. Gray matter differences in patients with functional movement disorders. Neurol. 2018;91(20):e1870-9. [PubMed ID: 30305450]. [PubMed Central ID: PMC6260194]. https://doi.org/10.1212/WNL.0000000000006514.
-
16.
Klumpp H, Post D, Angstadt M, Fitzgerald DA, Phan KL. Anterior cingulate cortex and insula response during indirect and direct processing of emotional faces in generalized social anxiety disorder. Biol Mood Anxiety Disord. 2013;3:7. [PubMed ID: 23547713]. [PubMed Central ID: PMC3632493]. https://doi.org/10.1186/2045-5380-3-7.
-
17.
Shy M, Fung S, Boone TB, Karmonik C, Fletcher SG, Khavari R. Functional magnetic resonance imaging during urodynamic testing identifies brain structures initiating micturition. J Urol. 2014;192(4):1149-54. [PubMed ID: 24769029]. [PubMed Central ID: PMC5485249]. https://doi.org/10.1016/j.juro.2014.04.090.
-
18.
Pattij T, Schetters D, Schoffelmeer AN. Dopaminergic modulation of impulsive decision making in the rat insular cortex. Behav Brain Res. 2014;270:118-24. [PubMed ID: 24837747]. https://doi.org/10.1016/j.bbr.2014.05.010.
-
19.
Menon V, Gallardo G, Pinsk MA, Nguyen VD, Li JR, Cai W, et al. Microstructural organization of human insula is linked to its macrofunctional circuitry and predicts cognitive control. Elife. 2020;9. [PubMed ID: 32496190]. [PubMed Central ID: PMC7308087]. https://doi.org/10.7554/eLife.53470.
-
20.
Pavuluri M, May A. I Feel, Therefore, I am: The Insula and Its Role in Human Emotion, Cognition and the Sensory-Motor System. AIMS Neuroscience. 2015;2(1):18-27. https://doi.org/10.3934/Neuroscience.2015.1.18.
-
21.
Garcia-Larrea L, Peyron R. Pain matrices and neuropathic pain matrices: a review. Pain. 2013;154 Suppl 1:S29-43. [PubMed ID: 24021862]. https://doi.org/10.1016/j.pain.2013.09.001.
-
22.
Apkarian AV, Bushnell MC, Treede RD, Zubieta JK. Human brain mechanisms of pain perception and regulation in health and disease. Eur J Pain. 2005;9(4):463-84. [PubMed ID: 15979027]. https://doi.org/10.1016/j.ejpain.2004.11.001.
-
23.
Tracey I, Mantyh PW. The cerebral signature for pain perception and its modulation. Neuron. 2007;55(3):377-91. [PubMed ID: 17678852]. https://doi.org/10.1016/j.neuron.2007.07.012.
-
24.
Perez DL, Williams B, Matin N, LaFrance WC, Costumero-Ramos V, Fricchione GL, et al. Corticolimbic structural alterations linked to health status and trait anxiety in functional neurological disorder. J Neurol Neurosurg Psychiatry. 2017;88(12):1052-9. [PubMed ID: 28844071]. https://doi.org/10.1136/jnnp-2017-316359.
-
25.
Lhomond O, Teasdale N, Simoneau M, Mouchnino L. Neural Consequences of Increasing Body Weight: Evidence from Somatosensory Evoked Potentials and the Frequency-Specificity of Brain Oscillations. Front Hum Neurosci. 2016;10:318. [PubMed ID: 27445758]. [PubMed Central ID: PMC4925671]. https://doi.org/10.3389/fnhum.2016.00318.
-
26.
Mazzola V, Vuilleumier P, Latorre V, Petito A, Gallese V, Popolizio T, et al. Effects of emotional contexts on cerebello-thalamo-cortical activity during action observation. PLoS One. 2013;8(9). e75912. [PubMed ID: 24086664]. [PubMed Central ID: PMC3784399]. https://doi.org/10.1371/journal.pone.0075912.
-
27.
Macar F, Vidal F, Casini L. The supplementary motor area in motor and sensory timing: evidence from slow brain potential changes. Exp Brain Res. 1999;125(3):271-80. [PubMed ID: 10229018]. https://doi.org/10.1007/s002210050683.
-
28.
Schmahmann JD, Sherman JC. The cerebellar cognitive affective syndrome. Brain. 1998;121 ( Pt 4):561-79. [PubMed ID: 9577385]. https://doi.org/10.1093/brain/121.4.561.
-
29.
Arthuis M, Micoulaud-Franchi JA, Bartolomei F, McGonigal A, Guedj E. Resting cortical PET metabolic changes in psychogenic non-epileptic seizures (PNES). J Neurol Neurosurg Psychiatry. 2015;86(10):1106-12. [PubMed ID: 25466258]. https://doi.org/10.1136/jnnp-2014-309390.
-
30.
Schrag AE, Mehta AR, Bhatia KP, Brown RJ, Frackowiak RS, Trimble MR, et al. The functional neuroimaging correlates of psychogenic versus organic dystonia. Brain. 2013;136(Pt 3):770-81. [PubMed ID: 23436503]. [PubMed Central ID: PMC3580272]. https://doi.org/10.1093/brain/awt008.
-
31.
Todd AJ. Neuronal circuitry for pain processing in the dorsal horn. Nat Rev Neurosci. 2010;11(12):823-36. [PubMed ID: 21068766]. [PubMed Central ID: PMC3277941]. https://doi.org/10.1038/nrn2947.
-
32.
Coombes SA, Misra G. Pain and motor processing in the human cerebellum. Pain. 2016;157(1):117-27. [PubMed ID: 26307859]. https://doi.org/10.1097/j.pain.0000000000000337.
-
33.
Egloff N, Gander ML, Gerber S, von Kanel R, Wiest R. [Chronic right-sided pain-associated nondermatomal somatosensory deficit following an accident]. Praxis (Bern 1994). 2010;99(13):797-801. DE. [PubMed ID: 20572002]. https://doi.org/10.1024/1661-8157/a000173.
-
34.
Ghaffar O, Staines WR, Feinstein A. Unexplained neurologic symptoms: an fMRI study of sensory conversion disorder. Neurol. 2006;67(11):2036-8. [PubMed ID: 17159115]. https://doi.org/10.1212/01.wnl.0000247275.68402.fc.
-
35.
Vuilleumier P, Schwartz S. Emotional facial expressions capture attention. Neurol. 2001;56(2):153-8. [PubMed ID: 11160948]. https://doi.org/10.1212/wnl.56.2.153.