Abstract
Background:
This study aims to investigate the effects of Bacillus coagulans T4 and Lactobacillus paracasei TD3 probiotics on skeletal muscle inflammation and oxidative stress in C57BL/6J mice fed a high-fat diet (HFD).Methods:
Probiotics B. coagulans T4, and L. paracasei TD3 were administered to male C57BL/6J mice fed with HFD. The gene expression of macrophage infiltration markers, inflammatory cytokines, and oxidative stress indicators in the muscle tissue was investigated.Results:
Treatment with B. coagulans T4 and L. paracasei TD3 reduced macrophage infiltration, accompanied by a decrease in the expression of monocyte chemoattractant protein-1 (MCP-1) and an increase in the expression of interleukin (IL)-10. On the other hand, L. paracasei TD3 decreased malondialdehyde (MDA) while B. coagulans T4 decreased carbonyl and increased catalase activity.Conclusions:
Treatment with probiotics B. coagulans T4 and L. paracasei TD3 partially ameliorated obesity-induced skeletal muscle inflammation in HFD-fed mice.Keywords
High-Fat Diet Skeletal Muscle Inflammation Oxidative Stress Probiotic
1. Background
The increase in the prevalence of obesity over the past 20 years has been alarming worldwide (1). Obesity, as a complex and major metabolic disease, is caused by adipose tissue expansion due to an imbalance between energy intake and expenditure. The excess fat is stored in adipocytes, resulting in hypertrophy and hyperplasia, which has been associated with type II diabetes mellitus, fatty liver disease, insulin resistance, and chronic inflammation (2-4). In obesity, hypertrophied adipocytes overproduce various inflammatory cytokines, disrupting the cross-talk between the adipose tissue and important organs involved in metabolic processes, such as the liver and skeletal muscle (5, 6). Skeletal muscles, as one of the main metabolic tissues, have a prominent role in regulating glucose and lipid metabolism (7). Inflammatory mediators affect muscles’ sensitivity to insulin (8), among which tumor necrosis factor α (TNF-α), as well as other pro-inflammatory cytokines produced by macrophages within the adipose tissue, seems to play a key role in insulin resistance pathogenesis in obese individuals (9, 10). Obesity and a high-fat diet (HFD) can change macrophages’ phenotype from M2 (anti-inflammatory) to M1 (pro-inflammatory) (11). M1 macrophages highly express inducible nitric oxide synthase (iNOS) and pro-inflammatory cytokines (e.g., TNF-α, IL-1, IL-6), while M2 macrophages mainly express arginase1 (ARG1) and/or anti-inflammatory cytokines (e.g., IL-10) (12).
On the other hand, it has been shown that in addition to inflammation, HFD leads to oxidative stress by inducing the production of reactive oxygen species (ROS) and reducing the expression of antioxidant factors such as superoxide dismutase (SOD). The production of ROS and inflammatory factors leads to insulin resistance and diabetes (13). Recently, the role of gut microbiota has been suggested in regulating caloric intake and energy expenditure, thereby contributing to obesity and its metabolic complications (14). There is evidence that genetically obese animal models and patients with obesity have fewer Bacteroidetes and more Firmicutes in comparison with controls, boosting the energy yield from the host’s diet (14-16). Gut dysbiosis impairs the gut barrier and enhances the circulatory levels of gut-derived endotoxins like lipopolysaccharides (LPS). Lipopolysaccharides can penetrate into various organs, activating the immune system after being recognized by toll-like receptor (TLR)-4, nurturing low-grade inflammation in the gut and other organs (17, 18). Gut dysbiosis and chronic low-grade inflammation are pertinent to obesity and insulin resistance (17, 19-21).
Recent investigations have proposed that the manipulation of gut microbiota and the use of probiotics (microorganisms with beneficial biological functions) can be novel approaches to treating obesity and its related complication (20, 22). Probiotics are living microorganisms that, when administered in adequate amounts, have shown beneficial physiologic or therapeutic effects on the host (23). Probiotics have been documented to promote anti-inflammatory effects and modulate hyperphagia, weight gain, fat mass loss, and glucose tolerance (24-27).
Bifidobacterium and Lactobacillus are well-studied probiotics with beneficial effects on the health status and the ability to survive in various delivery formats during gastric transit (28, 29). Bacillus species have received attention in research as their spores enable them to survive in severe processing and storage conditions, as well as in the harsh environment of the gastrointestinal tract (GIT) (30).
Among Bacillus species, Bacillus coagulans was first isolated from spoiled canned milk by Hammer in 1915, attracting increasing attention since then (31). Bacillus coagulans is a spore-forming, gram-positive, lactic-producing, catalase-positive, and facultative anaerobic bacterium with a GRAS (generally recognized as safe) status affirmed by the US Food and Drug Administration (FDA). Animal and preclinical studies on B. coagulans have been focused on its beneficial effects on diverse diseases and conditions, including irritable bowel syndrome, colitis, rheumatoid arthritis, and obesity (31, 32). The results of one study showed that drinking apple vinegar in combination with B. coagulans improved serum lipid profile and insulin resistance and prevented hepatic steatosis induced by HFD in mice (32). Lactobacillus (Lactic acid bacteria (LAB)), a main component of human GIT microbial flora, is usually used as probiotics, either alone or in combination with other agents, for fermentation in the food and dairy industries. Lactobacillus species are non-spore-forming, gram-positive, anaerobic bacteria that have been noted to promote immunostimulatory effects, maintain the intestinal microbial balance, prevent GI infections, and nurture hypocholesterolemic effects (33-36).
Different Lactobacillus species have been investigated for their potential beneficial effects on obesity. For instance, L. reuteri has been associated with weight gain, while L. casei and L. paracasei were associated with weight loss (33). Moreover, L. plantarum was reported to correct plasma lipid profiles and body weight and attenuate systemic inflammation in rats with HFD-induced obesity (37).
There is evidence that the ingestion of L. paracasei in the long term reduces HFD-induced obesity by decreasing body weight and abdominal fat in rats (38). Another investigation on school-aged children documented that L. paracasei had a protective role against obesity induced by an unhealthy diet (33). In another study, data have shown that the consumption of L. paracasei prebiotics and synbiotics for 12 weeks reduced abdominal dysbiosis and inflammation in insulin-resistant obese male Wistar rats (39).
2. Objectives
Although there has been a rapid growth in studies on the effects of probiotics on metabolic abnormalities, the role of B. coagulans and L. paracasei on muscle inflammation and oxidative stress in HFD-induced obesity has not been investigated. In the present study, we hypothesized that B. coagulans and L. paracasei could attenuate skeletal muscle inflammation in mice models of HFD-induced obesity. For this purpose, we fed B.coagulans T4 (IBRC-N10791) and L. paracasei TDC3 (IBRC-M 10791) to C57BL/6J mice models of HFD-induced obesity and assessed their impacts on the levels of pro/anti-inflammatory cytokines and oxidative stress markers in the skeletal muscle tissue.
3. Methods
3.1. Animals and Diet
Animal study protocols were carried out and reported according to animal husbandry standards issued by the Care and Use of Laboratory Animals Committee of Shahid Beheshti University of Medical Sciences, Tehran, Iran (IR.SBMU.RETECH.REC.1399.235) and ARRIVE guidelines for the reporting of animal experiments. Experiments were performed on 6 - 8-week-old male C57BL/6J mice purchased from the Pasteur Institute of Iran and housed individually in cages under the temperature of 23 ± 1°C, 55% humidity, and a 12-hr light/dark cycle. After an initial acclimation period, the mice were weighed and randomly divided into either the standard chow diet (SCD) [10 kcal% fat, n = 8] or HFD [60 kcal% fat, n = 32]. The mice were fed with these regimes for ten weeks.
Afterward, mice in the HFD group were categorized into four groups (eight mice per group): HFD control group, HFD + L. paracasei TD3 (IBRC-M 10791) (LP, 1 × 10 9 colony-forming units, CFU/ day), HFD + B. coagulans T4 (IBRC-N10791) (BC, 1 × 10 9 CFU/ day), and HFD + B. coagulans T4 + L. paracasei TD3 (BC and LP, 5 × 10 8 CFU/ day). These mice received the probiotics for another eight weeks. Lyophilized powders of L. paracasei TD3 and B. coagulans T4 were purchased from Tak Biogene Company (Iran). Water, diet consumption, and weight of animals in each group were recorded weekly.
The composition of SCD and HFD was prepared based on a research diet formulation (D12492i). The pork fat used in the study was replaced by a minced sheep tail. At the end of the experiment, all mice were sacrificed, and their tissue and blood samples were used to measure fasting blood glucose (FBG) (n = 5 per group), serum triglyceride (TG) (n = 5 per group), serum insulin level (n = 5 per group), mRNA expression level (n = 5 per group) and oxidative stress markers (n = 4-6 per group).
Blood samples were collected by a cardiac puncture; then, sera were separated and kept at -80°C for further analysis. Quadriceps muscle tissues were immediately isolated, washed with cold phosphate-buffered saline (PBS), snap-frozen in liquid nitrogen, and held at -80°C for molecular analyses.
3.2. Measuring Biochemical Parameters
After blood sampling and separating sera, biochemical parameters, such as FBG and TG, were measured using enzymatic methods and kits provided by Pars Azmoon Co. (Iran). Also, insulin was measured using an ELISA kit (MBS762025, MY BioSource, CA, USA) according to the manufacturer’s procedures, and insulin resistance was determined by the homeostasis model assessment of insulin resistance (HOMA-IR) using the following formula: Fasting glucose (mmol/l) × fasting insulin (μIU/ml)/22.5 (40).
3.3. Measuring Oxidative Stress Markers in the Quadriceps Muscle
The thiobarbituric acid (TBA) method was used to measure malondialdehyde (MDA), a marker of lipid peroxidation, in the muscle tissue. The reaction of TBA and MDA occurs in the presence of ferric chloride and butylated hydroxytoluene, and its optical absorption is then read at 532 nm (41).
The total antioxidant capacity (TAC) of serum was evaluated based on the conversion of ferric tripyridyltriazine complex (Fe3+-TPTZ) to ferrous tripyridyltriazine (Fe2+-TPTZ) at a low pH. Then optical absorption was read at the wavelength of 593 nm. The level of protein carbonyl was measured by the 2, 4 dinitrophenylhydrazine method described earlier (42). Total thiol level in the skeletal muscle tissue was measured using 2, 2′′-dinitro- 5, 5′ dithiodibenzoic acids (DTNB) (Sigma-Aldrich) reagent, which reacts with thiol groups and forms a yellow complex with a maximum absorbance at 412 nm. We used commercial kits (SOD activity Navand Salamat, Iran) and (Catalase activity Navand Salamat, Iran) to measure manganese superoxide dismutase (MnSOD) and catalase (CAT) activity in the skeletal muscle tissue. It should be noted that the values of the above-mentioned markers were normalized based on the protein concentration of the tissues measured by the Bradford assay.
3.4. Quantitative PCR
Real-time quantitative PCR was performed to determine the expression of key genes responsible for macrophage infiltration into muscles [F4/80] and macrophage polarization [CD11c, CD 206, iNOS, and ARG1], as well as the genes encoding pro/anti-inflammatory cytokines [IL-1β, IL-6, IL-10, TNF-α, MCP-1], TLR2, and TLR4 in the muscle tissue. Total RNA was isolated from snap-frozen samples using a GeneAll Hybrid-R RNA purification kit (GeneAll, Seoul, Korea). First, the purity and integrity of the total RNA extracted were determined using a NanoDrop device by determining the A260/280 and A260/230 ratios. Then cDNA synthesis was performed using the RevertAid First Strand cDNA Synthesis Kit (Thermo Fisher Scientific). Quantitative reverse transcription-polymerase chain reaction (qRT-PCR) was performed using SYBR Green RealQ Plus 2 × Master Mix Green (Ampliqon) in a Real-time PCR System (Applied Biosystems, CA). The primers used have been listed in Table 1. Materials used to assess gene expression included 20 μL of PCR master mix, ten μL of SYBR Green, 1 μL of each of forward and reverse primers, 1 μL cDNA, and 7 μL of nuclease-free water. The PCR reaction was conducted in 35 cycles, and β-actin served as the internal control gene. The relative expressions of the target genes were determined by the 2-ΔΔCT formula.
The Sequences of the Primers Used for Real-time PCR
Target Genes | Sequence (5' - 3') | Product Length (bp) |
---|---|---|
TNF-α | 142 | |
F | TGCTCTGTGAAGGGAATGGG | |
R | ACCCTGAGCCATAATCCCCT | |
IL-6 | 138 | |
F | GTTCTCTGGGAAATCGTGGA | |
R | TCCAGTTTGGTAGCATCCATC | |
IL-1β | 76 | |
F | TTCCTTGTGCAAGTGTCTGAAG | |
R | CACTGTCAAAAGGTGGCATTT | |
IL-10 | 216 | |
F | ATGCTGCCTGCTCTTACTGACTG | |
R | CCCAAGTAACCCTTAAAGTCCTGC | |
F4/80 | 165 | |
F | CTTTGGCTATGGGCTTCCAGTC | |
R | GCAAGGAGGACAGAGTTTATCGTG | |
CD11c | 86 | |
F | GCAGAGCCAGAACTTCCCAA | |
R | TGCTACCCGAGCCATCAATC | |
iNOS | 199 | |
F | TCCTACACCACACCAAAC | |
R | CTCCAATCTCTGCCTATCC | |
CD206 | 161 | |
F | CCTCTGGTGAACGGAATGAT | |
R | CTTCCTTTGGTCAGCTTTGG | |
ARG1 | 148 | |
F | TTGGCTTGCTTCGGAACTC | |
R | GGAGGAGAAGGCGTTTGC | |
TLR2 | 70 | |
F | GCATCCGAATTGCATCACCG | |
R | CCTCTGAGATTTGACGCTTTGT | |
TLR4 | 119 | |
F | TCCCTGCATAGAGGTAGTTCC | |
R | TCAAGGGGTTGAAGCTCAGA | |
MCP1 | 105 | |
F | ACTGCATCTGCCCTAAGGTCTTCA | |
R | AGAAGTGCTTGAGGTGGTTGTGGA | |
β-actin | 171 | |
F | CATCCGTAAAGACCTCTATGCCAAC | |
R | ATGGAGCCACCGATCCACA |
3.5. Statistical Analysis
Statistical analysis was performed using Prism software (GraphPad Prism 9). Data were analyzed by one-way analysis of variance (ANOVA) followed by Tukey’s multiple comparison tests at the α = 0.05 level. Data were presented as Mean ± SD, and P values < 0.05 were considered statistically significant.
4. Results
4.1. The Effects of Bacillus coagulans T4 and Lactobacillus paracasei TD3 on Obesity-induced Alterations in Biochemical Parameters
First, obesity-related characteristics were assessed in the mice receiving different diets. The HFD-fed group showed a significant increase in weight compared to the SCD group (P < 0.0001). Data revealed that L. paracasei and/or B. coagulans T4, both alone and in combination, decreased weight in the HFD group (Table 2).
Also, HFD-fed mice showed higher levels of FBG, serum insulin, and serum TG in comparison with the SCD group (P = 0.0001), indicating that L. paracasei TD3 and B. coagulans T4, either alone or in combination with each other, could reduce the levels of these metabolic parameters in mice models of obesity (Table 2).
The Characteristics of Mice in Study Groups a
Variables | Groups | ||||
---|---|---|---|---|---|
SCD | HFD | HFD + B.coagulans T4 | HFD + L. paracasei TD3 | HFD + B. coagulans T4 + L. paracasei TD3 | |
FBS (mg/dL) | 110.25 ± 10.55 | 269.5 ± 28.43 b | 184.37 ± 26.84 c | 188.37 ± 28.16 c | 156.75 ± 32.21 c |
Insulin (mg/dL) | 0.9240 ± 0.22 | 3.152 ± 0.45 b | 1.656 ± 0.37 d | 1.678 ± 0.35 d | 1.254 ± 0.59 c |
Weight (gr) | 27.49 ± 1.26 | 40.96 ± 1.70 b | 32.15 ± 1.70 c | 30.57 ± 0.90 c | 29.72 ± 1.69 c |
TG (mg/dL) | 54.5 ± 7.37 | 139.62 ± 14.06 b | 57.37 ± 12.44 c | 63.87 ± 4.5 c | 57.75 ± 7.40 c |
4.2. The Effects of Bacillus coagulans T4 and Lactobacillus paracasei TD3 on HFD-induced Skeletal Muscle Inflammation
To determine whether B. coagulans T4 and L. paracasei TD3 affect HFD-induced skeletal muscle inflammation or not, the mRNA expressions of F4/80 (a marker for macrophage infiltration), iNOS and CD11c (a marker of M1 macrophages), as well as CD206 and ARG1 (M2 macrophages’ markers), were investigated. Our observations showed that F4/80 and CD11c expression levels in the HFD group were increased in the skeletal muscle tissue as compared with the SCD group. Furthermore, F4/80 gene expression was decreased in all three experimental groups of mice receiving probiotics (L. paracasei TD3, B. coagulans T4, and B. coagulans T4+ L. paracasei TD3) compared to the HFD control group (Figure 1A). It was noticed that B. coagulans T4 and L. paracasei TD3, alone but not in combination, reduced CD11c gene expression compared to the HFD group (Figure 1B). The polarization of macrophages from the M1 to M2 phenotype has been shown to modulate inflammation control in skeletal muscles. Our findings showed that the expression of iNOS was upregulated in the HFD group compared to the SCD group, even though the difference was marginally significant (P = 0.051, Figure 1C). Furthermore, CD206 expression level was higher in the HFD compared to the SCD group (P = 0.0037), while its expression decreased in mice receiving B. coagulans T4 alone and B. coagulans T4 + L. paracasei TD3 compared to the HFD control group (P < 0.05, Figure 1D). Our results also showed no noticeable change in ARG1 expression in probiotic-treated groups compared to the HFD group (Figure 1E).
The effects of Bacillus coagulans and Lactobacillus paracasei on the marker of macrophage polarization and infiltration of the skeletal muscle: F4/80 (A), CD11c (B), iNOS (C), CD206 (D), and ARG1 (E). The bars represent mean ± SD values (5 mice per group). *P < 0.05, **P < 0.01, ***P < 0.001, and ****P < 0.0001. SCD: Standard chow diet; HFD: High-fat diet; HFD + B. coagulans T4: HFD + Bacillus coagulans T4; HFD + L. paracasei TD3: HFD+Lactobacillus paracasei TD3; HFD + B. coagulans T4+L. paracasei TD3: HFD + Bacillus coagulans T4 + Lactobacillus paracasei TD3; iNOS: Inducible nitric oxide synthetase; ARG1: Arginase1.
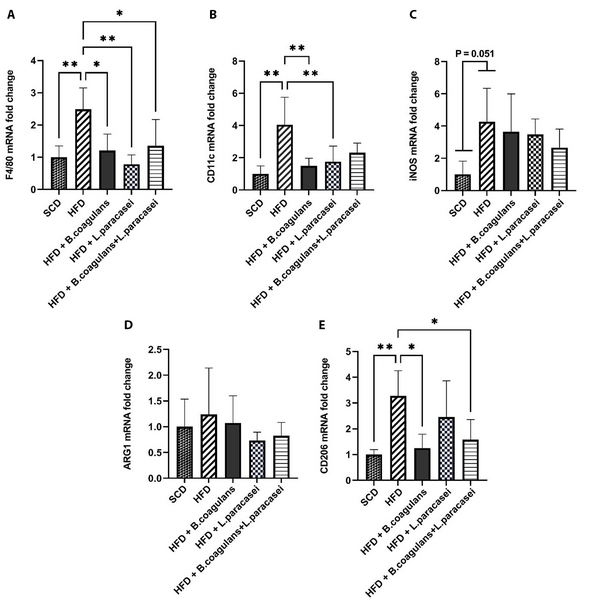
To determine the exact molecular mechanism behind the anti-inflammatory effects of Bacillus coagulans T4 and L. paracasei TD3, pro- and anti-inflammatory cytokines’ gene expressions were evaluated in the skeletal muscle tissue. The gene expression of TNF-α was increased in the HFD group compared to the SCD group (P < 0.05), while its expression was reduced in mice fed with either L. paracasei TD3 or B. coagulans T4 alone, as well as in the animals treated with the combination of both organisms, compared to the HFD control group (Figure 2A). There were no significant differences between the groups regarding the mRNA levels of IL-1β and IL-6 (Figure 2B and C). Also, evaluating IL-10 gene expression as an anti-inflammatory cytokine revealed its downregulation in the HFD group compared to the SCD group. In addition, IL-10 mRNA level showed a significant increase in mice treated with either L. paracasei TD3 or B.coagulans T4 alone compared to the HFD group (Figure 2E).
Our results showed that the expression of MCP-1, a crucial factor in the regulation of macrophage infiltration and recruitment, decreased significantly in the skeletal muscle tissues of the mice treated with either B. coagulans T4 or L. paracasei TD3 alone compared to the HFD group (Figure 2D).
Moreover, neither HFD nor supplementation with the aforementioned probiotics could significantly change the transcript levels of the TLR2 and TLR4 genes in the skeletal muscle (Figure 2F and Figure 2G).
The effects of Bacillus coagulans and Lactobacillus paracasei on the gene expression of inflammatory cytokines and chemokines: TNF-α (A), IL-1β (B), IL-6 (C), MCP-1 (D), IL-10 (E), TLR-2 (F), and TLR-4 (G). Each bar represents the mean ± SD value from five mice per group. *P < 0.05, **P < 0.01, ***P < 0.001, and ****P < 0.0001. SCD: Standard chow diet; HFD: High-fat diet; HFD + B. coagulans T4: HFD +Bacillus coagulans T4; HFD + L. paracasei TD3: HFD + Lactobacillus paracasei TD3; HFD+B. coagulans T4+L. paracasei TD3: HFD + Bacillus coagulans T4 + Lactobacillus paracasei TD3; TNF-α: Tumor necrosis factor alpha; IL-1β: Interleukin-1 beta; IL-6: Interleukin-6; MCP-1: Monocyte chemoattractant protein-1; IL-10: Interleukin-10; TLR2: Toll-like receptor 2; TLR4: Toll-like receptor 4.
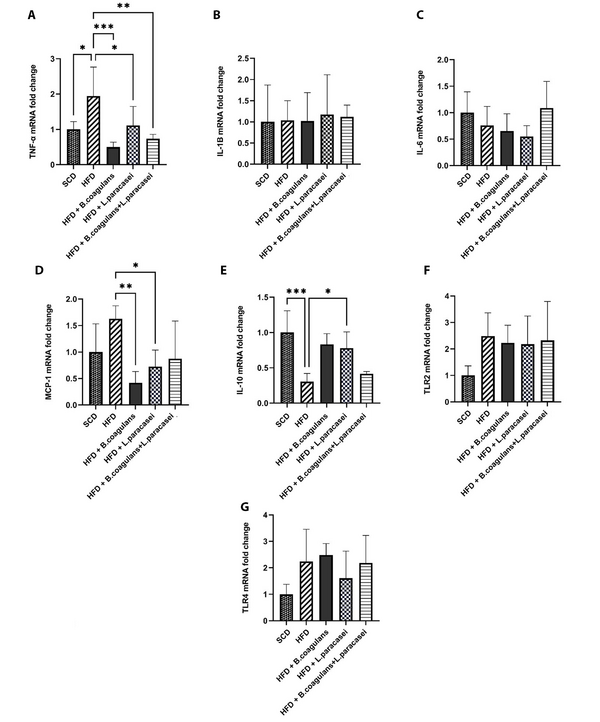
4.3. The Effects of Bacillus coagulans T4 and Lactobacillus paracasei TD3 on Oxidative Stress Markers in the Skeletal Muscles of HFD-fed Mice
The levels of TAC, MDA, thiol, carbonyl, and the activity of SOD and catalase (CAT) were assessed in the quadriceps muscles of experimental mice to evaluate changes in oxidative stress markers. The HFD group showed significantly lower TAC levels compared to the SCD group (Figure 3A), and MDA and carbonyl levels were increased in the HFD group compared to the SCD group; however, MDA and carbonyl levels were lower in other probiotic-treated groups compared to the HFD group. Nevertheless, the difference was significant for MDA only in mice treated with L. paracasei TD3 and for carbonyl only in mice fed with B. coagulans T4 (Figure 3C and D). Catalase activity was higher in the B. coagulans T4-treated group than in the HFD control group (Figure 3E), and SOD activity showed a significant decline in the HFD group compared to the SCD group (P = 0.033) but a significant elevation in all three probiotic-treated groups (Figure 3F). Regarding total thiol levels, the results showed no significant differences between the study groups (Figure 3B).
The effects of Bacillus coagulans and Lactobacillus paracasei on oxidative stress markers: TAC (A), total thiol (B), MDA (C), carbonyl (D), catalase activity (E), and SOD activity (F). Each bar represents the mean ± SD value from four to six mice per group. *P < 0.05, **P < 0.01, ***P < 0.001, and ****P < 0.0001. SCD: Standard chow diet; HFD: High-fat diet; HFD + B. coagulans T4: HFD + Bacillus coagulans T4; HFD + L. paracasei TD3: HFD + Lactobacillus paracasei TD3; HFD+B. coagulans T4+L. paracasei TD3: HFD + Bacillus coagulans T4+Lactobacillus paracasei TD3; TAC: Total antioxidant capacity; MDA: Malondialdehyde; SOD: Superoxide dismutase.
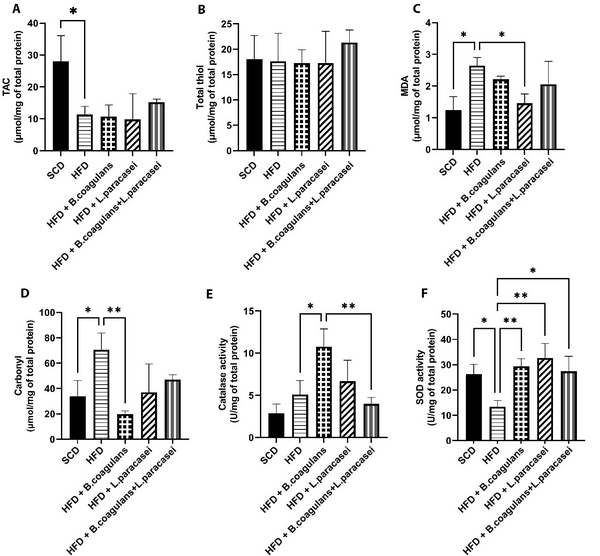
5. Discussion
In an attempt to understand the possible ameliorative effects of B. coagulans T4 and L. paracasei TD3 on plasma oxidative stress markers and skeletal muscle inflammation, we here investigated the effects of supplementing these probiotics to C57BL/6J mice models of HFD-induced obesity. The results showed that B. coagulans T4 and L. paracasei TD3, alone and in combination with each other, significantly decreased body weight and ameliorated obesity-related oxidative and inflammatory parameters, such as serum TG, FBG, and insulin levels.
The accumulation of macrophages in the skeletal muscle leads to local and systemic inflammation following obesity, and skeletal muscle inflammation plays an important role in systemic metabolic dysfunction. In line with previous studies (43, 44), we found a significant increase in F4/80 mRNA level along with a decrease in the gene expression of CD11c in the HFD group compared to the SCD group, suggesting that HFD-induced obesity augmented macrophage infiltration, which was reversed following the supplementation of B. coagulans T4 and L. paracasei TD3, suggesting that these probiotics attenuated inflammation in the skeletal muscle tissue.
Probiotics have different effects on macrophage phenotypes. For instance, some probiotics can activate M1 macrophages, facilitating the elimination of intracellular pathogens, but others may exert an anti-inflammatory effect (45). In a study, a probiotic cocktail of L delbruckei sp. bulgaricus DWT1 and Streptococcus thermophilus DWT4 shifted the balance of macrophages from M2 to M1 phenotype in-vitro (46). In another investigation, L. pentosus var. plantarum C29 induces M2 macrophage in mouse hippocampal macrophages from male C57BL/6J mice (47).
Obesity and HFD augmented the expression of the TNF-α pro-inflammatory gene and reduced the expression of anti-inflammatory (IL-10) genes. In the current study, this expression pattern was not seen in other investigated genes, such as IL-1β, IL-6, MCP-1, TLR2, and TLR4, following supplementation with the HFD. Although the exact reason for this observation could not be explained in the present study, it could be speculated that treatment for 18 weeks with the HFD was not sufficient for inducing all inflammatory markers in the adipose tissue. It is noteworthy that adipose tissue inflammation is generally triggered before the development of inflammation in other tissues (48).
Regarding the impact of probiotics supplementation on inflammatory genes’ expression, our data demonstrated that B. coagulans T4 and L. paracasei TD3 alone decreased TNF-α and MCP-1 expression and increased IL-10 expression. Also, combined treatment with these probiotics only reduced the expression of TNF-α. Supporting the present data, the combined oral administration of L. mucosae AN1 and L. fermentum SNR1 decreased the plasma levels of IL-6 and TNF-α and increased IL-10 levels in the paw tissues of the rat models of acute and chronic inflammation. The discrepancy observed between our research, and the aforementioned study may be related to the fact that the expression of inflammatory genes in the recent study was measured in the local inflammation induction area (49).
In another study, the effects of Bacillus strains on the expression levels of TNFα, IFNγ, MCP-1, IL-12, and IL-1β were evaluated in the liver and skeletal muscle of C57BL/6J male mice, where the mice were fed with HFD for two weeks and then received probiotics for 13 weeks. The results revealed that in the liver, all cytokines decreased, but this decline was marginally significant only for IL-1β. Furthermore, the expression of MCP-1 significantly declined in the skeletal muscles of Bacillus-treated mice, while the decrease in the levels of TNFα, IL-1β, and IFNγ was statistically nonsignificant (50). In another study, mice were fed with HFD for three weeks, which increased the level of IL-10 protein in the skeletal muscle in the HFD group compared to the SCD group (43). It is worth mentioning that the time of initiating the treatment after HFD supplementation varies in different studies and might be the reason for inconsistencies.
It is well-established that obesity induces oxidative stress and, in turn, oxidative stress, in a vicious cycle, worsens inflammation, contributing to the development of obesity-related metabolic abnormalities (51). Here, B. coagulans T4, and L. paracasei TD3 probiotics could partly augment the antioxidant defense system and reduce oxidative stress markers, partly explaining the reduction in skeletal muscle inflammation in obese rats following oral supplementation with B. coagulans T4 and L. paracasei TD3.
It should be noted that we found no statistical difference in metabolic parameters, oxidative stress markers, and transcript levels of inflammatory genes between mice treated with the combination of B.coagulans T4 and L. paracasei TD3 and the animals receiving each probiotic alone. Hence, it can be speculated that the combined administration of L. paracasei TD3 and B. coagulans T4 had no favorable effects in comparison with when each probiotic was administered alone. There is still a great level of uncertainty about whether or not a mixture of several probiotics is more effective than a single probiotic strain. Although our results in some aspects are in line with others’ findings (49, 52, 53), discrepancies may be related to the type of probiotic strains, duration of treatment, and the dosage of the probiotics used. More studies are needed to establish the role of these parameters.
5.1. Conclusions
It seems that B. coagulans T4 and L. paracasei TD3 probiotics, either alone or in combination, could partly prevent obesity-induced inflammation by suppressing macrophage infiltration, shifting macrophages’ phenotype from M1 (pro-inflammatory) to M2 (anti-inflammatory), decreasing the production of pro-inflammatory cytokines, and enhancing the antioxidant defense system (Figure 4). These results suggest that B. coagulans T4 and L. paracasei TD3 may be highly beneficial for overcoming obesity-related adverse effects.
Schematic representation of the effect of Bacillus coagulans T4 and Lactobacillus paracasei TD3 on the skeletal muscle’s oxidative stress and inflammation in high-fat diet-fed C57BL/6J mice. Both alone and in combination, B. coagulans T4 and L. paracasei TD3 probiotics could partly prevent obesity-induced inflammation by suppressing macrophage infiltration, macrophage phenotype switching from M1 to M2, decreasing the production of pro-inflammatory cytokines, and enhancing the antioxidant defense system. The upward arrow indicates an increase, the downward arrow indicates a decrease, and the horizontal arrow indicates no change in comparison with the HFD-induced model. HFD: High-fat diet, FBG: Fasting blood glucose, TG: Triglyceride, TNF-α: Tumor necrosis factor-α, IL-1β: Interleukin 1β, IL-6: Interleukin 6, MCP-1: Monocyte chemoattractant protein-1, IL-10: Interleukin 10, TLR-2: Toll-like receptor-2, TLR-4: Toll-like receptor-4, ARG1: Arginase-1, iNOS: Inducible nitric oxide synthase, TAC: Total antioxidant capacity, MDA: Malondialdehyde, Mn-SOD: Manganese superoxide dismutase, CAT: Catalase.
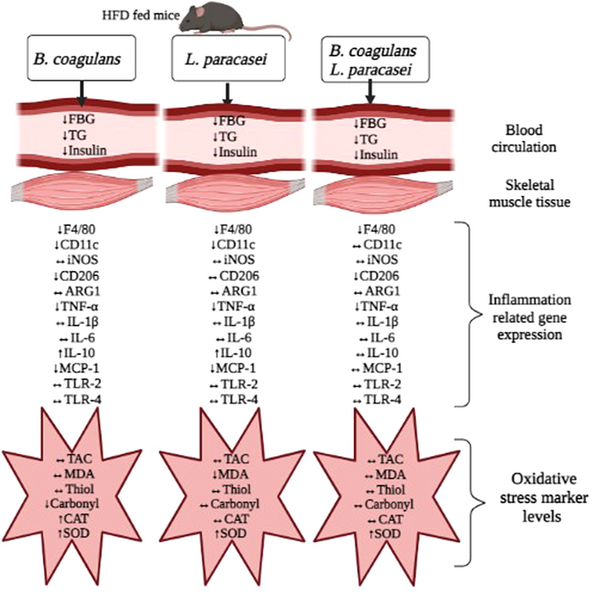
Acknowledgements
References
-
1.
Eckardt K, Taube A, Eckel J. Obesity-associated insulin resistance in skeletal muscle: role of lipid accumulation and physical inactivity. Rev Endocr Metab Disord. 2011;12(3):163-72. [PubMed ID: 21336841]. https://doi.org/10.1007/s11154-011-9168-2.
-
2.
Fabbrini E, Sullivan S, Klein S. Obesity and nonalcoholic fatty liver disease: biochemical, metabolic, and clinical implications. Hepatology. 2010;51(2):679-89. [PubMed ID: 20041406]. [PubMed Central ID: PMC3575093]. https://doi.org/10.1002/hep.23280.
-
3.
Fox CS, Massaro JM, Hoffmann U, Pou KM, Maurovich-Horvat P, Liu CY, et al. Abdominal visceral and subcutaneous adipose tissue compartments: association with metabolic risk factors in the Framingham Heart Study. Circulation. 2007;116(1):39-48. [PubMed ID: 17576866]. https://doi.org/10.1161/CIRCULATIONAHA.106.675355.
-
4.
Wu H, Ballantyne CM. Skeletal muscle inflammation and insulin resistance in obesity. J Clin Invest. 2017;127(1):43-54. [PubMed ID: 28045398]. [PubMed Central ID: PMC5199705]. https://doi.org/10.1172/JCI88880.
-
5.
Chawla A, Nguyen KD, Goh YP. Macrophage-mediated inflammation in metabolic disease. Nat Rev Immunol. 2011;11(11):738-49. [PubMed ID: 21984069]. [PubMed Central ID: PMC3383854]. https://doi.org/10.1038/nri3071.
-
6.
Harwood HJ. The adipocyte as an endocrine organ in the regulation of metabolic homeostasis. Neuropharmacology. 2012;63(1):57-75. [PubMed ID: 22200617]. https://doi.org/10.1016/j.neuropharm.2011.12.010.
-
7.
Akhmedov D, Berdeaux R. The effects of obesity on skeletal muscle regeneration. Front Physiol. 2013;4:371. [PubMed ID: 24381559]. [PubMed Central ID: PMC3865699]. https://doi.org/10.3389/fphys.2013.00371.
-
8.
Kewalramani G, Bilan PJ, Klip A. Muscle insulin resistance: Assault by lipids, cytokines and local macrophages. Curr Opin Clin Nutr Metab Care. 2010;13(4):382-90. [PubMed ID: 20495453]. https://doi.org/10.1097/MCO.0b013e32833aabd9.
-
9.
Weisberg SP, McCann D, Desai M, Rosenbaum M, Leibel RL, Ferrante AJ. Obesity is associated with macrophage accumulation in adipose tissue. J Clin Invest. 2003;112(12):1796-808. [PubMed ID: 14679176]. [PubMed Central ID: PMC296995]. https://doi.org/10.1172/JCI19246.
-
10.
Xu H, Barnes GT, Yang Q, Tan G, Yang D, Chou CJ, et al. Chronic inflammation in fat plays a crucial role in the development of obesity-related insulin resistance. J Clin Invest. 2003;112(12):1821-30. [PubMed ID: 14679177]. [PubMed Central ID: PMC296998]. https://doi.org/10.1172/JCI19451.
-
11.
Chen L, Chen R, Wang H, Liang F. Mechanisms Linking Inflammation to Insulin Resistance. Int J Endocrinol. 2015;2015:508409. [PubMed ID: 26136779]. [PubMed Central ID: PMC4468292]. https://doi.org/10.1155/2015/508409.
-
12.
Pillon NJ, Bilan PJ, Fink LN, Klip A. Cross-talk between skeletal muscle and immune cells: muscle-derived mediators and metabolic implications. Am J Physiol Endocrinol Metab. 2013;304(5):E453-65. [PubMed ID: 23277185]. https://doi.org/10.1152/ajpendo.00553.2012.
-
13.
Gregersen S, Samocha-Bonet D, Heilbronn LK, Campbell LV. Inflammatory and oxidative stress responses to high-carbohydrate and high-fat meals in healthy humans. J Nutr Metab. 2012;2012:238056. [PubMed ID: 22474579]. [PubMed Central ID: PMC3306970]. https://doi.org/10.1155/2012/238056.
-
14.
Frazier TH, DiBaise JK, McClain CJ. Gut microbiota, intestinal permeability, obesity-induced inflammation, and liver injury. JPEN J Parenter Enteral Nutr. 2011;35(5 Suppl):14S-20S. [PubMed ID: 21807932]. https://doi.org/10.1177/0148607111413772.
-
15.
Ley RE, Backhed F, Turnbaugh P, Lozupone CA, Knight RD, Gordon JI. Obesity alters gut microbial ecology. Proc Natl Acad Sci U S A. 2005;102(31):11070-5. [PubMed ID: 16033867]. [PubMed Central ID: PMC1176910]. https://doi.org/10.1073/pnas.0504978102.
-
16.
Ley RE, Turnbaugh PJ, Klein S, Gordon JI. Microbial ecology: Human gut microbes associated with obesity. Nature. 2006;444(7122):1022-3. [PubMed ID: 17183309]. https://doi.org/10.1038/4441022a.
-
17.
Ding S, Chi MM, Scull BP, Rigby R, Schwerbrock NM, Magness S, et al. High-fat diet: bacteria interactions promote intestinal inflammation which precedes and correlates with obesity and insulin resistance in mouse. PLoS One. 2010;5(8). e12191. [PubMed ID: 20808947]. [PubMed Central ID: PMC2922379]. https://doi.org/10.1371/journal.pone.0012191.
-
18.
Xiao S, Fei N, Pang X, Shen J, Wang L, Zhang B, et al. A gut microbiota-targeted dietary intervention for amelioration of chronic inflammation underlying metabolic syndrome. FEMS Microbiol Ecol. 2014;87(2):357-67. [PubMed ID: 24117923]. [PubMed Central ID: PMC4255291]. https://doi.org/10.1111/1574-6941.12228.
-
19.
Cani PD, Amar J, Iglesias MA, Poggi M, Knauf C, Bastelica D, et al. Metabolic endotoxemia initiates obesity and insulin resistance. Diabetes. 2007;56(7):1761-72. [PubMed ID: 17456850]. https://doi.org/10.2337/db06-1491.
-
20.
Jia W, Li H, Zhao L, Nicholson JK. Gut microbiota: A potential new territory for drug targeting. Nat Rev Drug Discov. 2008;7(2):123-9. [PubMed ID: 18239669]. https://doi.org/10.1038/nrd2505.
-
21.
Lopez-Cepero A, Palacios C. Association of the intestinal microbiota and obesity. Puerto Rico health sciences journal. 2015;34:60-4.
-
22.
Hoffman FA. Development of probiotics as biologic drugs. Clin Infect Dis. 2008;46 Suppl 2:S125-7. discussion S144-51. [PubMed ID: 18181717]. https://doi.org/10.1086/523326.
-
23.
Schrezenmeir J, de Vrese M. Probiotics, prebiotics, and synbiotics—approaching a definition. Am J Clin Nutr. 2001;73(2):361s-4s. https://doi.org/10.1093/ajcn/73.2.361s.
-
24.
Champagne CP, Gardner NJ, Roy D. Challenges in the addition of probiotic cultures to foods. Crit Rev Food Sci Nutr. 2005;45(1):61-84. [PubMed ID: 15730189]. https://doi.org/10.1080/10408690590900144.
-
25.
Stenman LK, Waget A, Garret C, Klopp P, Burcelin R, Lahtinen S. Potential probiotic Bifidobacterium animalis ssp. lactis 420 prevents weight gain and glucose intolerance in diet-induced obese mice. Benef Microbes. 2014;5(4):437-45. [PubMed ID: 25062610]. https://doi.org/10.3920/BM2014.0014.
-
26.
Yadav H, Lee JH, Lloyd J, Walter P, Rane SG. Beneficial metabolic effects of a probiotic via butyrate-induced GLP-1 hormone secretion. J Biol Chem. 2013;288(35):25088-97. [PubMed ID: 23836895]. [PubMed Central ID: PMC3757173]. https://doi.org/10.1074/jbc.M113.452516.
-
27.
Yoo SR, Kim YJ, Park DY, Jung UJ, Jeon SM, Ahn YT, et al. Probiotics L. plantarum and L. curvatus in combination alter hepatic lipid metabolism and suppress diet-induced obesity. Obesity (Silver Spring). 2013;21(12):2571-8. [PubMed ID: 23512789]. https://doi.org/10.1002/oby.20428.
-
28.
Abdou RM, Zhu L, Baker RD, Baker SS. Gut microbiota of nonalcoholic fatty liver disease. Dig Dis Sci. 2016;61(5):1268-81. [PubMed ID: 26898658]. https://doi.org/10.1007/s10620-016-4045-1.
-
29.
Liu H, Cui SW, Chen M, Li Y, Liang R, Xu F, et al. Protective approaches and mechanisms of microencapsulation to the survival of probiotic bacteria during processing, storage and gastrointestinal digestion: A review. Crit Rev Food Sci Nutr. 2019;59(17):2863-78. [PubMed ID: 28933562]. https://doi.org/10.1080/10408398.2017.1377684.
-
30.
Soares MB, Santos-Junior VA, Tavares Filho ER, Lollo PCB, Morato PN, Amaya-Farfan J, et al. The step of incorporation of Bacillus coagulans GBI-30 6086 into "requeijao cremoso" processed cheese does Not affect metabolic homeostasis of rats. Front Microbiol. 2019;10:2332. [PubMed ID: 31695686]. [PubMed Central ID: PMC6817512]. https://doi.org/10.3389/fmicb.2019.02332.
-
31.
Cao J, Yu Z, Liu W, Zhao J, Zhang H, Zhai Q, et al. Probiotic characteristics of Bacillus coagulans and associated implications for human health and diseases. J Funct Foods. 2020;64. https://doi.org/10.1016/j.jff.2019.103643.
-
32.
Urtasun R, Diaz-Gomez J, Arana M, Pajares MJ, Oneca M, Torre P, et al. A Combination of Apple Vinegar Drink with Bacillus coagulans Ameliorates High Fat Diet-Induced Body Weight Gain, Insulin Resistance and Hepatic Steatosis. Nutrients. 2020;12(9). [PubMed ID: 32825073]. [PubMed Central ID: PMC7551919]. https://doi.org/10.3390/nu12092504.
-
33.
Castaneda-Marquez AC, Diaz-Benitez CE, Bahena-Roman M, Campuzano-Benitez GE, Galvan-Portillo M, Campuzano-Rincon JC, et al. Lactobacillus paracasei as a protective factor of obesity induced by an unhealthy diet in children. Obes Res Clin Pract. 2020;14(3):271-8. [PubMed ID: 32518007]. https://doi.org/10.1016/j.orcp.2020.04.005.
-
34.
Chiang SS, Pan TM. Beneficial effects of Lactobacillus paracasei subsp. paracasei NTU 101 and its fermented products. Appl Microbiol Biotechnol. 2012;93(3):903-16. [PubMed ID: 22159887]. https://doi.org/10.1007/s00253-011-3753-x.
-
35.
De Smet I, De Boever P, Verstraete W. Cholesterol lowering in pigs through enhanced bacterial bile salt hydrolase activity. Br J Nutr. 1998;79(2):185-94. [PubMed ID: 9536863]. https://doi.org/10.1079/bjn19980030.
-
36.
Kumar R, Grover S, Batish VK. Hypocholesterolaemic effect of dietary inclusion of two putative probiotic bile salt hydrolase-producing Lactobacillus plantarum strains in Sprague-Dawley rats. Br J Nutr. 2011;105(4):561-73. [PubMed ID: 20923582]. https://doi.org/10.1017/S0007114510003740.
-
37.
Ben Salah R, Trabelsi I, Hamden K, Chouayekh H, Bejar S. Lactobacillus plantarum TN8 exhibits protective effects on lipid, hepatic and renal profiles in obese rat. Anaerobe. 2013;23:55-61. [PubMed ID: 23891961]. https://doi.org/10.1016/j.anaerobe.2013.07.003.
-
38.
Tanida M, Shen J, Maeda K, Horii Y, Yamano T, Fukushima Y, et al. High-fat diet-induced obesity is attenuated by probiotic strain Lactobacillus paracasei ST11 (NCC2461) in rats. Obes Res Clin Pract. 2008;2(3):I-II. [PubMed ID: 24351773]. https://doi.org/10.1016/j.orcp.2008.04.003.
-
39.
Thiennimitr P, Yasom S, Tunapong W, Chunchai T, Wanchai K, Pongchaidecha A, et al. Lactobacillus paracasei HII01, xylooligosaccharides, and synbiotics reduce gut disturbance in obese rats. Nutrition. 2018;54:40-7. [PubMed ID: 29705500]. https://doi.org/10.1016/j.nut.2018.03.005.
-
40.
Matthews DR, Hosker JP, Rudenski AS, Naylor BA, Treacher DF, Turner RC. Homeostasis model assessment: Insulin resistance and beta-cell function from fasting plasma glucose and insulin concentrations in man. Diabetologia. 1985;28(7):412-9. [PubMed ID: 3899825]. https://doi.org/10.1007/BF00280883.
-
41.
Sadeghi H, Jahanbazi F, Sadeghi H, Omidifar N, Alipoor B, Kokhdan EP, et al. Metformin attenuates oxidative stress and liver damage after bile duct ligation in rats. Res Pharm Sci. 2019;14(2):122-9. [PubMed ID: 31620188]. [PubMed Central ID: PMC6791170]. https://doi.org/10.4103/1735-5362.253359.
-
42.
Majidi Z, Ansari M, Maghbooli Z, Ghasemi A, Ebrahimi SSS, Hossein-Nezhad A, et al. Oligopin(R) supplementation mitigates oxidative stress in postmenopausal women with osteopenia: A randomized, double-blind, placebo-controlled trial. Phytomedicine. 2021;81:153417. [PubMed ID: 33250314]. https://doi.org/10.1016/j.phymed.2020.153417.
-
43.
Hong EG, Ko HJ, Cho YR, Kim HJ, Ma Z, Yu TY, et al. Interleukin-10 prevents diet-induced insulin resistance by attenuating macrophage and cytokine response in skeletal muscle. Diabetes. 2009;58(11):2525-35. [PubMed ID: 19690064]. [PubMed Central ID: PMC2768157]. https://doi.org/10.2337/db08-1261.
-
44.
Pincu Y, Linden MA, Zou K, Baynard T, Boppart MD. The effects of high fat diet and moderate exercise on TGFbeta1 and collagen deposition in mouse skeletal muscle. Cytokine. 2015;73(1):23-9. [PubMed ID: 25689619]. https://doi.org/10.1016/j.cyto.2015.01.013.
-
45.
Wang Y, Liu H, Zhao J. Macrophage polarization induced by probiotic bacteria: A concise review. Probiotics Antimicrob Proteins. 2020;12(3):798-808. [PubMed ID: 31741313]. https://doi.org/10.1007/s12602-019-09612-y.
-
46.
Guha D, Banerjee A, Mukherjee R, Pradhan B, Peneva M, Aleksandrov G, et al. A probiotic formulation containing Lactobacillus bulgaricus DWT1 inhibits tumor growth by activating pro-inflammatory responses in macrophages. J Funct Foods. 2019;56:232-45. https://doi.org/10.1016/j.jff.2019.03.030.
-
47.
Woo JY, Gu W, Kim KA, Jang SE, Han MJ, Kim DH. Lactobacillus pentosus var. plantarum C29 ameliorates memory impairment and inflammaging in a D-galactose-induced accelerated aging mouse model. Anaerobe. 2014;27:22-6. [PubMed ID: 24657159]. https://doi.org/10.1016/j.anaerobe.2014.03.003.
-
48.
van der Heijden RA, Sheedfar F, Morrison MC, Hommelberg PP, Kor D, Kloosterhuis NJ, et al. High-fat diet induced obesity primes inflammation in adipose tissue prior to liver in C57BL/6j mice. Aging (Albany NY). 2015;7(4):256-68. [PubMed ID: 25979814]. [PubMed Central ID: PMC4429090]. https://doi.org/10.18632/aging.100738.
-
49.
Ayyanna R, Ankaiah D, Arul V. Anti-inflammatory and antioxidant properties of probiotic Bacterium Lactobacillus mucosae AN1 and Lactobacillus fermentum SNR1 in wistar albino rats. Front Microbiol. 2018;9:3063. [PubMed ID: 30619149]. [PubMed Central ID: PMC6301997]. https://doi.org/10.3389/fmicb.2018.03063.
-
50.
Kim B, Kwon J, Kim MS, Park H, Ji Y, Holzapfel W, et al. Protective effects of Bacillus probiotics against high-fat diet-induced metabolic disorders in mice. PLoS One. 2018;13(12). e0210120. [PubMed ID: 30596786]. [PubMed Central ID: PMC6312313]. https://doi.org/10.1371/journal.pone.0210120.
-
51.
Fernandez-Sanchez A, Madrigal-Santillan E, Bautista M, Esquivel-Soto J, Morales-Gonzalez A, Esquivel-Chirino C, et al. Inflammation, oxidative stress, and obesity. Int J Mol Sci. 2011;12(5):3117-32. [PubMed ID: 21686173]. [PubMed Central ID: PMC3116179]. https://doi.org/10.3390/ijms12053117.
-
52.
Wang W, Li Q, Chai W, Sun C, Zhang T, Zhao C, et al. Lactobacillus paracasei Jlus66 extenuate oxidative stress and inflammation via regulation of intestinal flora in rats with non alcoholic fatty liver disease. Food Sci Nutr. 2019;7(8):2636-46. [PubMed ID: 31428351]. [PubMed Central ID: PMC6694609]. https://doi.org/10.1002/fsn3.1118.
-
53.
Zeng Z, Yuan Q, Yu R, Zhang J, Ma H, Chen S. Ameliorative effects of probiotic Lactobacillus paracasei NL41 on insulin sensitivity, oxidative stress, and beta-cell function in a Type 2 diabetes mellitus rat model. Mol Nutr Food Res. 2019;63(22). e1900457. [PubMed ID: 31433912]. https://doi.org/10.1002/mnfr.201900457.