Abstract
Background:
Chemotherapy remains a primary approach to cancer treatment, widely applied in bladder cancer (BC). However, the various side effects and resistance associated with chemotherapeutic drugs pose significant challenges in BC therapy, prompting interest in natural compounds like luteolin. Studies focus on its effects on key biological processes involved in BC, including metastasis, apoptosis, and autophagy.Objectives:
This study investigated the regulation of mRNA expression of genes associated with apoptosis (BCL2, P53), autophagy (ULK1, ATG12), and metastasis (MMP2, MMP9) in malignant BC cells treated with luteolin.Methods:
This was an in vitro experimental study. EJ138 BC cells were treated with various concentrations of luteolin, and its impact on cell viability, proliferation, and gene expression was assessed.Results:
MTT assay results confirmed that luteolin reduced the proliferation rate of BC cells. Flow cytometry indicated increased cell death in EJ138 BC cells following luteolin treatment. RT-PCR findings demonstrated that luteolin upregulated P53, ULK1, and ATG12 expression while downregulating BCL2 mRNA expression. However, luteolin treatment in EJ138 cells did not significantly alter MMP2 and MMP9 expression levels.Conclusions:
These findings indicate that luteolin exerts cytotoxic effects on EJ138 BC cells by dysregulating mRNA expression of genes involved in apoptosis and autophagy. Therefore, luteolin shows potential as an effective anti-cancer agent for BC therapy.Keywords
1. Background
Bladder cancer (BC) is the thirteenth leading cause of cancer-related deaths globally, with a 5-year survival rate of 77% (1). In 2018, approximately 550,000 people were diagnosed with BC, accounting for around 3% of all new cancer cases. Greece and Lebanon have the highest incidence rates of BC among men and women (2). Tobacco smoking is the primary risk factor for BC, contributing to roughly 50% of cases. Other significant risk factors include alcohol, cannabis, and opium use, low fiber and grain intake, caffeine, chronic inflammation, arsenic, and nitrates (3). Currently, surgical resection, chemotherapy, radiotherapy, and immunotherapy are the primary treatment methods for BC, playing a crucial role in improving the 5-year survival rate and potential cure of BC patients (4). Despite advancements, various biological pathways and genes involved in key processes such as autophagy, apoptosis, and metastasis continue to influence BC cell proliferation, migration, and poor prognosis in BC patients (5).
Autophagy is a vital cellular process that maintains cellular integrity by recycling metabolic elements under stress conditions and is significantly involved in cancer cell development (6). Recent studies have shown that the dysregulation of autophagy-related genes, such as ATG12 and ULK1, plays a crucial role in the progression of various cancers, including gastric cancer, acute myeloid leukemia, renal cell carcinoma, and BC (7-9). P53 and BCL2 are essential in controlling the proliferation of malignant cancer cells by regulating apoptosis. Studies have indicated that up-regulating P53 and down-regulating BCL2 can suppress the growth of different cancer cells by inducing apoptosis (10). Conversely, down-regulating MMP2 and MMP9 has been shown to reduce metastasis and migration in retinoblastoma, bladder, oral, and ovarian cancer cells (11). Targeting these genes with novel agents could provide new treatment approaches for BC by regulating autophagy, apoptosis, and metastasis.
There is increasing interest in exploring herbal pharmacological agents capable of modulating multiple genes across various tumor types. Among these plant-based agents, luteolin—a flavonoid commonly found in various vegetables, plants, and fruits—has gained attention. Recent research has demonstrated that luteolin plays a significant role in several biological processes, including managing allergy, inflammation, diabetes, and neuroprotection (12). Additionally, these studies have revealed that luteolin exhibits anti-cancer effects by modifying molecular targets, regulating signaling pathways, and altering cellular processes such as apoptosis, cell cycle progression, metastasis, and autophagy, which collectively reduce invasion, migration, and proliferation in cancer cells (13). Luteolin has been shown to increase the expression of pro-apoptotic genes, such as P53 and Bax, while down-regulating anti-apoptotic genes like BCL2 and Bcl-xL (14). Luteolin’s ability to regulate MMP expression has made it a promising compound for preventing or slowing down cancer cell migration from the primary tumor site to distant tissues (15). Additionally, luteolin’s capacity to modulate autophagy is particularly relevant in cancer therapy and inflammation, where autophagy dysfunction plays a crucial role (16).
2. Objectives
Luteolin, a plant-based compound, shows potential in the prevention and treatment of various cancers. In this study, we investigate the anti-cancer effects of luteolin on EJ138 BC malignant cells by examining its impact on autophagic, apoptotic, and metastatic gene modulation, specifically targeting ATG12, ULK1, P53, BCL2, MMP2, and MMP9.
3. Methods
3.1. Study Design
This laboratory-based in vitro study involved exposing EJ138 BC cells to various concentrations of luteolin to assess its effects on cell viability, proliferation, and gene expression.
3.2. Chemicals and Reagents
Luteolin and MTT were obtained from Sigma Aldrich. Luteolin was dissolved in RPMI and stored in a refrigerator at 4°C.
3.3. Cell Culture
The EJ138 BC cell line was obtained from the National Cell Bank of Iran. This cell line was cultured in RPMI-1640 (Gibco) medium supplemented with 10% heat-inactivated FBS (Gibco), 50 μg/mL streptomycin (Sigma Aldrich), and 50 units/mL penicillin (Sigma Aldrich). The EJ138 BC cells were then maintained in an incubator with 5% CO₂ at 37°C.
3.4. Cell Viability Assay
The viability of EJ138 BC cells was assessed using the MTT colorimetric assay. In brief, 1×104 BC cells were seeded into each well of 96-well plates. After a 24-hour incubation, the BC cells were treated with various concentrations of luteolin (10 - 50 µM) for 24 and 48 hours. Subsequently, EJ138 BC cells were washed with PBS, and 20 µL of MTT solution was added to each well. The MTT solution was removed after 4 hours and replaced with 150 µL of DMSO (Scharlau Chemie). Finally, the 570 nm UV absorbance of EJ138 BC cells was measured using an ELISA plate reader.
3.5. Flow Cytometric Analysis for Apoptosis Characterization
After the EJ138 BC cells were seeded in a six-well plate (5 × 105 cells/well), 17.92 µM luteolin (corresponding to its IC50 value for the EJ138 BC cell line at 48 hours) was applied to treat these cancerous cells. Following 48 hours of treatment, the EJ138 cells were stained with FITC-conjugated annexin V and propidium iodide (PI) for 15 minutes. In the final step, apoptotic EJ138 cells were detected using a flow cytometer (17).
3.6. RNA Extraction and Real-time PCR
In the first step, total RNA was extracted from EJ138 BC cells using trizol, following the manufacturer’s instructions (Invitrogen, USA). Three micrograms of total RNA were reverse transcribed using the SMOBIO Kit (RP1300, Taiwan) according to the manufacturer's protocol. Real-time PCR was then performed with 2X qPCRBIO SYGreen Mix Lo-ROX (PCRBiosystems, England). The RT-PCR Detection System (Ruche Light Cycler 96, Germany) was used to analyze gene expression. Oligo primer analysis software (version 7.60) was employed for primer design. GAPDH was used as a housekeeping gene to serve as an internal control for normalization. Relative mRNA expression levels were determined using the ΔCT method (18).
3.7. Statistical Analysis
In this study, SPSS V.22 was used to analyze the data. The statistical significance of the differences was assessed using Student’s t-test. A P-value of < 0.05 was considered statistically significant.
4. Results
4.1. Luteolin Induces the Cellular Death in EJ138 Bladder Cancer Malignant Cells
The examination of BC cell viability using the MTT assay indicated that the IC50 values of luteolin's effect on the EJ138 BC cell line were 29.44 μM and 17.92 μM for 24 h and 48 h, respectively (Figure 1). As shown in Figure 1, cell viability decreased as the luteolin dose increased from 10 μM to 50 μM. These findings illustrate that the growth rate of EJ138 BC cells was inhibited by luteolin in a dose- and time-dependent manner.
Luteolin treatment raises significant cell death in bladder cancer (BC) cells. Cell viability analysis of EJ138 bladder cancerous cells, utilizing a time- and dose-dependent examination by MTT, was performed for 24 h and 48 h. EJ138 BC cells were treated with 10 - 50 µM luteolin.
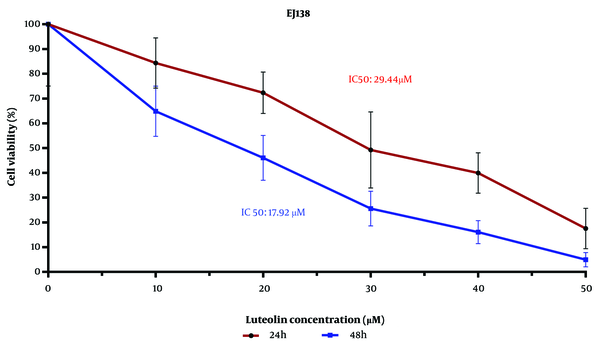
4.2. Luteolin Enhances the Apoptosis Rate in EJ138 Bladder Cancer Malignant Cells
To investigate whether luteolin mediates its cell death effects through apoptosis, EJ138 BC malignant cells were treated with 17.92 µM luteolin and analyzed by flow cytometry. The results of this investigation showed that treatment with luteolin increased apoptosis in the cancerous cells compared to the control group. As illustrated in Figure 2, luteolin enhanced the apoptotic rate in BC cells by 52%.
Flow cytometry was conducted to analyze the impacts of luteolin on the apoptosis of EJ138 bladder cancer (BC) cells after 48 h treatment with luteolin. Q1: Necrotic cells, Q2 and Q3: Apoptotic cells, Q4: Live cells.
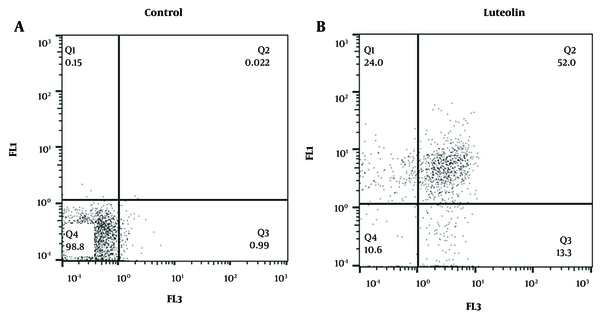
4.3. Effectiveness of Luteolin in mRNA Expression Levels of Autophagic, Apoptotic, and Metastatic Genes
In this study, we used real-time PCR to assess the roles of luteolin in regulating the mRNA expression levels of autophagic, apoptotic, and metastatic genes in EJ138 BC cells. Our findings showed that luteolin upregulated the mRNA expression of the P53, ULK1, and ATG12 genes (Figure 3B, C, D). We also confirmed that luteolin plays a crucial role in inducing apoptosis in EJ138 BC cells by downregulating BCL2 mRNA expression (Figure 3A). In contrast, treatment of BC cells with luteolin did not show a significant difference in the expression levels of MMP2 and MMP9 compared to untreated cells (Figure 3E, F).
The relative expression of BCL2 (A), P53 (B), ULK1 (C), ATG12 (D), MMP2 (E), and MMP9 (F) was examined by RT-PCR at 48 h and normalized to GAPDH mRNA level post-treatment of EJ138 bladder cancer (BC) cells with luteolin. (* P-value < 0.05, ** P-value < 0.01, and *** P-value < 0.001).
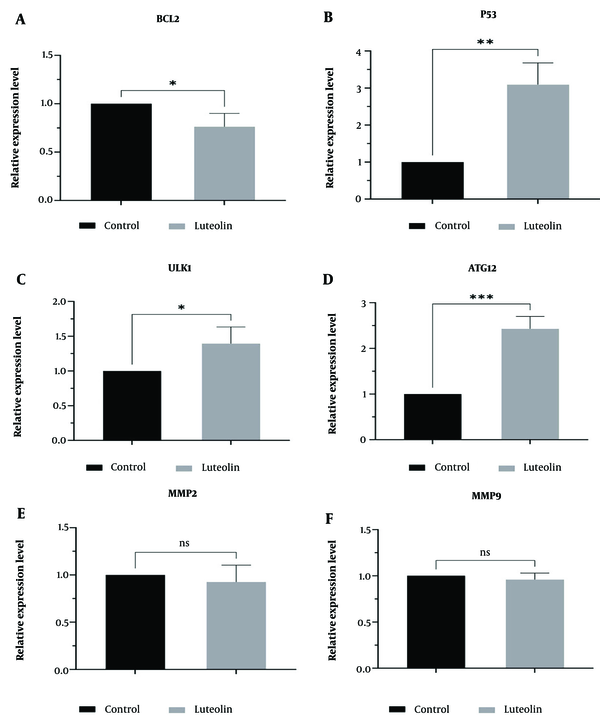
5. Discussion
Over recent years, significant advancements have been made in treating patients with various malignant cancers due to the development of anti-cancer agents (19). However, many cancer patients continue to suffer due to recurrence, diverse side effects of chemotherapeutic agents, and chemo-resistance (20). For these reasons, numerous studies have been conducted to explore new plant-based anti-cancer agents, such as flavonoid compounds, with fewer adverse effects on cancer patients (21, 22). Although various efforts have been undertaken to investigate the anti-cancer effects of luteolin against cancers, the relationship of metastasis, apoptosis, and autophagy with luteolin has not yet been explored. Therefore, investigating the association between luteolin and biological processes may be an essential step in overcoming various malignant cancerous cells, especially BC (20).
In this present study, we demonstrated that luteolin therapy significantly increased the number of apoptotic BC malignant cells compared with the untreated group. Similar to our study, Wu et al. revealed that luteolin markedly increased the apoptotic rate of triple-negative breast cancer (TNBC) cells. They showed that luteolin decreased the expression of SGT1 and AKT3 genes while increasing BNIP3 mRNA levels, which led to reduced proliferation, invasion, and migration of TNBC (23). In another study, Zhang et al. demonstrated that luteolin promoted cell death and inhibited colony formation in lung cancer cells by downregulating cyclin-D1, P-LIMK, and P-cofilin mRNA expression levels. They also confirmed that luteolin exerted its anti-cancer effects by suppressing P-cofilin, P-LIMK, and Ki-67 expression in lung cancer in vivo (24). Luteolin also promotes apoptosis in SMMC-7721 hepatocellular carcinoma cells by upregulating caspase-8 and downregulating BCL2 protein and mRNA levels (25). In 2020, Lida et al. illustrated that luteolin reduced the viability of T24 BC cells and induced G2/M cell-cycle arrest by increasing p21 and TRX1 and inhibiting p-S6 expression, which plays a crucial role in regulating the mTOR signaling pathway. Their in vivo findings showed that luteolin downregulated p-S6 and the Ki67-Labeling Index in rats, leading to a reduction in BC size (26). Furthermore, combination therapy of luteolin and TRAIL inhibited the growth of T24 BC cells by XIAP suppression and Bax upregulation (27).
Given the extensive apoptotic, autophagic, and anti-metastatic effects of luteolin in various cancer cells, few studies have examined the mechanisms involved (9). Therefore, in this study, we assessed changes in mRNA expression levels of genes that play critical roles in apoptosis, autophagy, and metastasis. The findings of this study illustrated that luteolin exerted its anti-proliferative effects by downregulating BCL2 and upregulating P53. We also showed that luteolin plays an essential role in the autophagy process of BC cells by upregulating ULK1 and ATG12. However, our results did not show significant changes in the expression levels of metastatic genes, including MMP2 and MMP9, in EJ138 BC cells. For this reason, to understand the exact mechanism of luteolin in BC cell metastasis, we suggest using a western blot assay in future studies.
In 2023, Yajie et al. reported that luteolin enhanced cell death rates and reduced cell proliferation by downregulating BCL2 and P-AKT and upregulating cytochrome C, Bax, and caspase-3 in MKN-45 gastric cancer cells (28). Chang et al. found that luteolin decreased cell growth and sensitized ovarian cancer cells to cisplatin by increasing P53 mRNA expression and decreasing VRK1 expression (29). Contrary to our study, Liu et al. reported that luteolin and ellagic acid reduced ovarian cancer cell metastasis by inhibiting MMP2 and MMP9 protein expression (30). Additionally, luteolin inhibited proliferation, invasion, and metastasis in A375 melanoma cells by downregulating MMP2 and MMP9 and upregulating TIMP-1 and TIMP-2 expression levels (31). Wang et al. indicated that luteolin has critical functions in reducing lung cancer cell metastasis by upregulating miR-106a and downregulating its target genes, including MMP2 and TWIST1 (32).
Although in vivo evidence suggests that luteolin has potential therapeutic effects, there is a significant gap in clinical trials investigating its efficacy and safety in human BC patients. More comprehensive studies, including long-term animal models and clinical trials, are necessary to determine luteolin’s therapeutic potential and safety in the treatment of human BC.
5.1. Conclusions
Altogether, the outcomes of our investigation illustrate that luteolin induces cell death in a dose- and time-dependent manner and inhibits the proliferation of BC cells. Furthermore, our findings demonstrated that luteolin has the potential to be considered as an adjuvant or complementary treatment alongside chemotherapy due to its multi-targeted effects on cancer cells in treating BC patients. Specifically, luteolin upregulates the mRNA expression of P53, ULK1, and ATG12 and downregulates BCL2 expression, genes that play crucial roles in apoptosis and autophagy processes. Therefore, the results of our experimental study provide a scientific basis suggesting that luteolin could offer a promising strategy in BC therapy through its substantial impact on regulating various biological mechanisms. Nonetheless, further investigations are warranted to clarify the exact mechanism of luteolin and explore BC therapy strategies.
References
-
1.
Zhang Y, Rumgay H, Li M, Yu H, Pan H, Ni J. The global landscape of bladder cancer incidence and mortality in 2020 and projections to 2040. J Glob Health. 2023;13:4109. [PubMed ID: 37712386]. [PubMed Central ID: PMC10502766]. https://doi.org/10.7189/jogh.13.04109.
-
2.
Saginala K, Barsouk A, Aluru JS, Rawla P, Padala SA, Barsouk A. Epidemiology of Bladder Cancer. Med Sci (Basel). 2020;8(1). [PubMed ID: 32183076]. [PubMed Central ID: PMC7151633]. https://doi.org/10.3390/medsci8010015.
-
3.
Jubber I, Ong S, Bukavina L, Black PC, Comperat E, Kamat AM, et al. Epidemiology of Bladder Cancer in 2023: A Systematic Review of Risk Factors. Eur Urol. 2023;84(2):176-90. [PubMed ID: 37198015]. https://doi.org/10.1016/j.eururo.2023.03.029.
-
4.
De George KC, Holt HR, Hodges SC. Bladder Cancer: Diagnosis and Treatment. Am Fam Physician. 2017;96(8):507-14. [PubMed ID: 29094888].
-
5.
Lin YC, Lin JF, Wen SI, Yang SC, Tsai TF, Chen HE, et al. Inhibition of High Basal Level of Autophagy Induces Apoptosis in Human Bladder Cancer Cells. J Urol. 2016;195(4 Pt 1):1126-35. [PubMed ID: 26519656]. https://doi.org/10.1016/j.juro.2015.10.128.
-
6.
Song B, Bian Q, Zhang YJ, Shao CH, Li G, Liu AA, et al. Downregulation of ASPP2 in pancreatic cancer cells contributes to increased resistance to gemcitabine through autophagy activation. Mol Cancer. 2015;14:177. [PubMed ID: 26438046]. [PubMed Central ID: PMC4594892]. https://doi.org/10.1186/s12943-015-0447-5.
-
7.
Li F, Guo H, Yang Y, Feng M, Liu B, Ren X, et al. Autophagy modulation in bladder cancer development and treatment (Review). Oncol Rep. 2019;42(5):1647-55. [PubMed ID: 31436298]. [PubMed Central ID: PMC6775810]. https://doi.org/10.3892/or.2019.7286.
-
8.
Liu L, Yan L, Liao N, Wu WQ, Shi JL. A Review of ULK1-Mediated Autophagy in Drug Resistance of Cancer. Cancers (Basel). 2020;12(2). [PubMed ID: 32033142]. [PubMed Central ID: PMC7073181]. https://doi.org/10.3390/cancers12020352.
-
9.
Cao QH, Liu F, Yang ZL, Fu XH, Yang ZH, Liu Q, et al. Prognostic value of autophagy related proteins ULK1, Beclin 1, ATG3, ATG5, ATG7, ATG9, ATG10, ATG12, LC3B and p62/SQSTM1 in gastric cancer. Am J Transl Res. 2016;8(9):3831-47. [PubMed ID: 27725863]. [PubMed Central ID: PMC5040681].
-
10.
Rahman N, Khan H, Zia A, Khan A, Fakhri S, Aschner M, et al. Bcl-2 Modulation in p53 Signaling Pathway by Flavonoids: A Potential Strategy towards the Treatment of Cancer. Int J Mol Sci. 2021;22(21). [PubMed ID: 34768743]. [PubMed Central ID: PMC8582810]. https://doi.org/10.3390/ijms222111315.
-
11.
Jiang H, Li H. Prognostic values of tumoral MMP2 and MMP9 overexpression in breast cancer: a systematic review and meta-analysis. BMC Cancer. 2021;21(1):149. [PubMed ID: 33568081]. [PubMed Central ID: PMC7877076]. https://doi.org/10.1186/s12885-021-07860-2.
-
12.
Cetinkaya M, Baran Y. Therapeutic Potential of Luteolin on Cancer. Vaccines (Basel). 2023;11(3). [PubMed ID: 36992138]. [PubMed Central ID: PMC10057337]. https://doi.org/10.3390/vaccines11030554.
-
13.
Ganai SA, Sheikh FA, Baba ZA, Mir MA, Mantoo MA, Yatoo MA. Anticancer activity of the plant flavonoid luteolin against preclinical models of various cancers and insights on different signalling mechanisms modulated. Phytother Res. 2021;35(7):3509-32. [PubMed ID: 33580629]. https://doi.org/10.1002/ptr.7044.
-
14.
Chang H, Li C, Huo K, Wang Q, Lu L, Zhang Q, et al. Luteolin Prevents H2O2-Induced Apoptosis in H9C2 Cells through Modulating Akt-P53/Mdm2 Signaling Pathway. Biomed Res Int. 2016;2016:5125836. [PubMed ID: 27525270]. [PubMed Central ID: PMC4976196]. https://doi.org/10.1155/2016/5125836.
-
15.
Cook MT. Mechanism of metastasis suppression by luteolin in breast cancer. Breast Cancer (Dove Med Press). 2018;10:89-100. [PubMed ID: 29928143]. [PubMed Central ID: PMC6003288]. https://doi.org/10.2147/BCTT.S144202.
-
16.
Ashrafizadeh M, Ahmadi Z, Farkhondeh T, Samarghandian S. Autophagy regulation using luteolin: new insight into its anti-tumor activity. Cancer Cell Int. 2020;20(1):537. [PubMed ID: 33292250]. [PubMed Central ID: PMC7641824]. https://doi.org/10.1186/s12935-020-01634-9.
-
17.
Panahizadeh R, Vatankhah MA, Jeddi F, Arabzadeh AA, Nejati-Koshki K, Najafzadeh N. Cytotoxicity of curcumin against CD44±prostate cancer cells: Roles of miR-383 and miR-708. Avicenna J Phytomed. 2022;13(4). https://doi.org/10.21203/rs.3.rs-1281066/v1.
-
18.
Vatankhah MA, Panahizadeh R, Nejati-Koshki K, Arabzadeh M, Arabzadeh AA, Najafzadeh N. Curcumin Upregulates miR-148a to Increase the Chemosensitivity of CD44-Positive Prostate Cancer Stem Cells to Paclitaxel Through Targeting the MSK1/IRS1 axis. Drug Res (Stuttg). 2022;72(8):457-65. [PubMed ID: 35868335]. https://doi.org/10.1055/a-1867-4805.
-
19.
Liu Z, Delavan B, Roberts R, Tong W. Lessons Learned from Two Decades of Anticancer Drugs. Trends Pharmacol Sci. 2017;38(10):852-72. [PubMed ID: 28709554]. https://doi.org/10.1016/j.tips.2017.06.005.
-
20.
Lee HS, Park BS, Kang HM, Kim JH, Shin SH, Kim IR. Role of Luteolin-Induced Apoptosis and Autophagy in Human Glioblastoma Cell Lines. Med J (Kaunas). 2021;57(9). [PubMed ID: 34577802]. [PubMed Central ID: PMC8470580]. https://doi.org/10.3390/medicina57090879.
-
21.
Dehelean CA, Marcovici I, Soica C, Mioc M, Coricovac D, Iurciuc S, et al. Plant-Derived Anticancer Compounds as New Perspectives in Drug Discovery and Alternative Therapy. Molecul J. 2021;26(4). [PubMed ID: 33669817]. [PubMed Central ID: PMC7922180]. https://doi.org/10.3390/molecules26041109.
-
22.
Montane X, Kowalczyk O, Reig-Vano B, Bajek A, Roszkowski K, Tomczyk R, et al. Current Perspectives of the Applications of Polyphenols and Flavonoids in Cancer Therapy. Molecul J. 2020;25(15). [PubMed ID: 32717865]. [PubMed Central ID: PMC7435624]. https://doi.org/10.3390/molecules25153342.
-
23.
Wu L, Lin Y, Gao S, Wang Y, Pan H, Wang Z, et al. Luteolin inhibits triple-negative breast cancer by inducing apoptosis and autophagy through SGK1-FOXO3a-BNIP3 signaling. Front Pharmacol. 2023;14:1200843. [PubMed ID: 37346292]. [PubMed Central ID: PMC10279868]. https://doi.org/10.3389/fphar.2023.1200843.
-
24.
Zhang M, Wang R, Tian J, Song M, Zhao R, Liu K, et al. Targeting LIMK1 with luteolin inhibits the growth of lung cancer in vitro and in vivo. J Cell Mol Med. 2021;25(12):5560-71. [PubMed ID: 33982869]. [PubMed Central ID: PMC8184676]. https://doi.org/10.1111/jcmm.16568.
-
25.
Cao Z, Zhang H, Cai X, Fang W, Chai D, Wen Y, et al. Luteolin Promotes Cell Apoptosis by Inducing Autophagy in Hepatocellular Carcinoma. Cell Physiol Biochem. 2017;43(5):1803-12. [PubMed ID: 29049999]. https://doi.org/10.1159/000484066.
-
26.
Iida K, Naiki T, Naiki-Ito A, Suzuki S, Kato H, Nozaki S, et al. Luteolin suppresses bladder cancer growth via regulation of mechanistic target of rapamycin pathway. Cancer Sci. 2020;111(4):1165-79. [PubMed ID: 31994822]. [PubMed Central ID: PMC7156788]. https://doi.org/10.1111/cas.14334.
-
27.
Park HS, Choi YH. Induction of Apoptosis by Combination Treatment with Luteolin and TRAIL in T24 Human Bladder Cancer Cells. J Korea Soc Food Sci Nutr. 2013;42(9):1363-9. https://doi.org/10.3746/jkfn.2013.42.9.1363.
-
28.
Yajie D, Feng L, Zhaoyan LI, Yan X, Nida C, Guangao Z, et al. Efficacy of luteolin on the human gastric cancer cell line MKN45 and underlying mechanism. J Tradit Chin Med. 2023;43(1):34-41. [PubMed ID: 36639993]. [PubMed Central ID: PMC9924784]. https://doi.org/10.19852/j.cnki.jtcm.2023.01.005.
-
29.
Chang X, Tamauchi S, Yoshida K, Yoshihara M, Yokoi A, Shimizu Y, et al. Downregulating vaccinia-related kinase 1 by luteolin suppresses ovarian cancer cell proliferation by activating the p53 signaling pathway. Gynecol Oncol. 2023;173:31-40. [PubMed ID: 37075494]. https://doi.org/10.1016/j.ygyno.2023.04.003.
-
30.
Liu H, Zeng Z, Wang S, Li T, Mastriani E, Li QH, et al. Main components of pomegranate, ellagic acid and luteolin, inhibit metastasis of ovarian cancer by down-regulating MMP2 and MMP9. Cancer Biol Ther. 2017;18(12):990-9. [PubMed ID: 29173024]. [PubMed Central ID: PMC5718784]. https://doi.org/10.1080/15384047.2017.1394542.
-
31.
Yao X, Jiang W, Yu D, Yan Z. Luteolin inhibits proliferation and induces apoptosis of human melanoma cells in vivo and in vitro by suppressing MMP-2 and MMP-9 through the PI3K/AKT pathway. Food Funct. 2019;10(2):703-12. [PubMed ID: 30663726]. https://doi.org/10.1039/c8fo02013b.
-
32.
Wang Q, Chen M, Tang X. Luteolin Inhibits Lung Cancer Cell Migration by Negatively Regulating TWIST1 and MMP2 Through Upregulation of miR-106a-5p. Integr Cancer Ther. 2024;23:15347354241247200. [PubMed ID: 38646808]. [PubMed Central ID: PMC11034356]. https://doi.org/10.1177/15347354241247223.