Abstract
Background:
Alzheimer's disease symptoms indicate that increased Aβ leads to central nervous system dysfunction. Exercise reduces Aβ deposition through the AMPK signaling pathway. Additionally, resveratrol (RSV) has neuroprotective effects related to cognitive decline.Objectives:
This study investigated the effects of aerobic training and RSV on the AMPK/PGC-1α/SIRT1 pathway in the hippocampus of Alzheimer's rats.Methods:
Thirty-five male Wistar rats were divided into five groups: Normal (NO), Alzheimer's (AD), Alzheimer's-training (ADT), Alzheimer's-resveratrol (ADRSV), and Alzheimer's-training-resveratrol (ADTRSV). The supplement groups received 20 mg of RSV (per kg of body weight) orally during the intervention period. The aerobic exercise program, which included running on a treadmill at speeds of 6 - 18 meters per minute, was performed 5 days a week for eight weeks.Results:
Alzheimer's induction caused a significant decrease in the expression of AMPK/PGC-1α/SIRT1 (P = 0.0001). Exercise and RSV significantly increased the expression of AMPK/PGC-1α/SIRT1 in AD rats (P < 0.05). Additionally, significant increases in the expression of AMPK, PGC-1α, and SIRT1 were observed in the ADTRSV group compared to the ADT group (P = 0.034, P = 0.020, and P = 0.038, respectively) and ADRSV (P = 0.026, P = 0.021, and P = 0.021).Conclusions:
AD induction was associated with decreased AMPK/PGC-1α/SIRT1 expression. Aerobic exercise and RSV consumption can reverse this decrease. Given the crucial role of this signaling pathway in hippocampal function, alterations in these indicators following physical activity and RSV use may partially mitigate Alzheimer's disease complications.Keywords
1. Background
The improvement in living standards is associated with increased age, and as the elderly population grows, the phenomenon of population aging occurs (1). By 2030, over half a billion people will be at risk of nervous system degradation due to aging, with 65% of this population affected by Alzheimer's disease (AD) (2). Pathological changes in the brains of Alzheimer's patients include brain atrophy characterized by senile plaques (SP) or β-amyloid plaques, neurofibrillary tangles (NFT), and neuron loss (3). The pathogenesis of Alzheimer's disease reveals that an imbalance between Aβ production and clearance causes neurodegeneration and dementia, leading to pathological processes such as tau protein hyperphosphorylation, inflammatory responses, and neuronal death (3). Although the mechanism of Aβ toxicity to neurons remains unclear, Aβ is known to induce SP deposition. Additionally, Aβ oligomers increase apoptosis or stimulate glial cells to overexpress nitric oxide synthase (iNOS), and elevated NO levels contribute to neuronal death (4). Since Aβ deposition plays a crucial role in brain disorders and contributes to AD, reducing Aβ deposition is a key therapeutic goal for AD (5). Exercise influences AMPK and reduces Aβ deposition (6). Physical activity is often considered a cost-effective and beneficial intervention for many diseases, and its effects are attributed to the activation of AMPK (7). PGC-1α and SIRT1 are downstream effectors of AMPK and are important targets for reducing Aβ. One study demonstrated that after 35 sessions of moderate-intensity treadmill exercise, learning and memory impairments in AD mice were improved, associated with a reduction in Aβ deposition. It is hypothesized that exercise increases the expression of PGC-1α and PPARγ due to AMPK activation, thereby reducing Aβ42 production (8). In addition to exercise, certain plants can directly and indirectly affect the nervous system. Resveratrol (RSV: 3,5,4′-trihydroxy-trans-stilbene) is a naturally occurring polyphenol found in grape skins, red wine, rhubarb, and other plants. Resveratrol has neuroprotective effects related to cognitive decline (9). For instance, Moussa et al. (10) demonstrated that 52 weeks of RSV treatment prevented the progression of mild to moderate AD (10). Another study indicated that 26 weeks of RSV treatment in overweight elderly individuals led to improved memory performance, retention, and hippocampal function (11). These studies highlight RSV as a promising treatment for neurodegenerative diseases. The primary mechanism of RSV's action involves the activation of sirtuin 1 (SIRT1). Sirtuins regulate essential intracellular processes such as energy consumption, metabolic patterns, control of reactive oxygen species (ROS) levels, DNA protection, and cellular aging (12). The combined therapeutic effects of exercise and RSV, both of which can protect the nervous system, are not well documented. A limited study has reported that aerobic exercise combined with RSV is associated with reduced Aβ oligomer toxicity, suppressed neuronal autophagy, and reduced apoptosis in AD mice (13). However, Allard et al. found that RSV treatment alone did not significantly affect APOE expression in the brain tissue of AD mice but was effective when combined with exercise (14).
2. Objectives
Physical activity and an active lifestyle are promising therapeutic strategies for preventing or delaying AD and other dementias. Additionally, there is growing evidence that antioxidant dietary supplements may also play a role in treating and preventing AD progression. We hypothesize that combining RSV administration with exercise training has a synergistic effect on the AMPK/PGC-1α/SIRT1 pathway in AD rats.
3. Methods
In this research, all animal experiments were conducted in accordance with animal protection policies (based on the Helsinki Convention) and the guidelines provided by the National Institute of Health for the care of laboratory animals. Thirty-five male Wistar rats, aged 8 weeks and weighing 223.17 ± 9.08 grams, were obtained from the Pasteur Institute and transferred to the animal laboratory. The ambient temperature was maintained at 22 ± 3°C, with a light-dark cycle of 12:12 hours. All animals had free access to water and rat chow. After a one-week acclimatization period to the new environmental conditions, the rats were divided into five groups, each consisting of eight rats: (1) normal (NO), (2) Alzheimer's (AD), (3) Alzheimer's-training (ADT), (4) Alzheimer's-resveratrol (ADRSV), and (5) Alzheimer's-training-resveratrol (ADTRSV).
3.1. Alzheimer's Induction
First, amyloid-β1-42 (Sigma-Aldrich) was dissolved in sterilized distilled water and incubated at 37°C for one week. The rats were anesthetized with an injection of ketamine (50 mg/kg) and xylazine (5 mg/kg) and then placed in the stereotaxic apparatus. The hair on the rats' heads was shaved, and the bregma and lambda sutures were identified using a sagittal cut. The CA1 region of the hippocampus was marked, and the skull was gently pierced. A Hamilton syringe was used to inject Aβ. Two microliters of Aβ were slowly injected into the brain over approximately one minute (15).
3.2. Exercise Protocol
The training schedule for AD rats is shown in Figure 1. Exercise on the treadmill began at 2 months of age and was divided into two stages: (1) familiarization with training (2 weeks) and (2) adaptation to training (8 weeks). During the familiarization phase, the rats underwent exercise training at speeds of 6 - 18 meters per minute for 15 - 45 minutes per session, five times a week. Following this, the rats engaged in the main exercise regimen on the treadmill for eight weeks, five days a week, at an intensity of 18 meters per minute for 45 minutes per session.
Exercise protocol
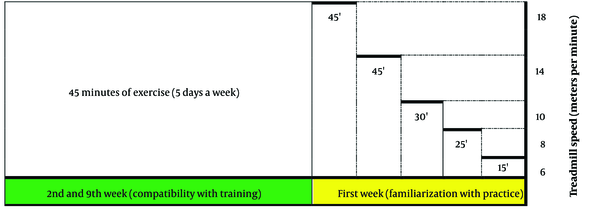
3.3. Resveratrol Consumption
Resveratrol (20 mg/kg from Sigma-Aldrich) or an equivalent volume of saline solution was administered orally by gavage to rats every morning (between 8:00 AM and 10:00 AM) for 2 months (8 weeks) (16, 17).
3.4. Laboratory Methods
After applying the independent variable, all the samples were similar and in basic conditions. Forty-eight hours after the last training session and 12 to 14 hours of fasting, the animals were anesthetized and sacrificed. Immediately after isolation and washing with saline, the hippocampal tissue was placed in tubes containing RNA, transferred to liquid nitrogen, and then stored in a refrigerator at -80°C until measurement.
3.5. Primer Design and Preparation
Table 1 shows the primer pattern. First, the primers were designed, and then total RNA was extracted from the tissues and converted into cDNA. The cDNA was then amplified by PCR and analyzed for the expression of the mentioned genes.
Primer Pattern
Genes | Forward Primers | Reverse Primers |
---|---|---|
AMPK | 5’- ACTATCAAAGACATACGAGAGCA -3’ | 5’- CTTGAGGGTCACCACTGTATAA -3’ |
PGC-1α | 5’- CAGAAGCAGAAAGCAATTGAAGA -3’ | 5’- GTTTCATTCGACCTGCGTAAAG -3’ |
SIRT1 | 5’- TCCTGTGGGATACCTGACTT-3’ | 5’- AAAGGAACCATGACACTGAATGA -3’ |
GAPDH | 5′-AGAAGGCTGGAGAAGATGAGG-3′ | 5′-TTGGTGCCTCGTGTCTTCTGT-3′ |
3.6. Data Analysis Procedure
After confirming the normal distribution of the data using the Shapiro-Wilk test and the homogeneity of variances using Levene's test, one-way analysis of variance and Tukey's post hoc test were used for statistical analysis. Calculations were performed using SPSS version 26 statistical software, and a significance level of P ≤ 0.05 was considered.
4. Results
The average weight of the groups is presented in Table 2.
Average Weight of Groups in Different Groups a
Group | NO (n = 7) | AD (n = 7) | ADT (n = 7) | ADRSV (n = 7) | ADTRSV (n = 7) |
---|---|---|---|---|---|
Weight (g) | 219.71 ± 10.61 | 225.57 ± 11.28 | 224.71 ± 9.41 | 222 ± 6.42 | 223.86 ± 8.49 |
Data analysis revealed a significant difference in the amount of AMPK expression in hippocampal tissue between different groups (P = 0.0001, F = 15.140) (Figure 2). Post hoc test results indicated a significant decrease in AMPK expression in the AD (P = 0.0001), ADT (P = 0.006), and ADRSV (P = 0.004) groups compared to the NO group. Additionally, a significant increase in AMPK expression was observed in the ADT (P = 0.036), ADRSV (P = 0.047), and ADTRSV (P = 0.0001) groups compared to AD, and in the ADTRSV group compared to the ADT (P = 0.034) and ADRSV (P = 0.026) groups (Figure 2).
Hippocampal AMPK expression in different groups by one-way analysis of variance (P < 0.05). A, difference from NO; B, difference from AD; C, difference from ADTRSV. Abbreviations: NO, normal; AD, Alzheimer's; ADT, Alzheimer's-training; ADRSV, Alzheimer's-resveratrol; ADTRSV Alzheimer's-training-resveratrol.
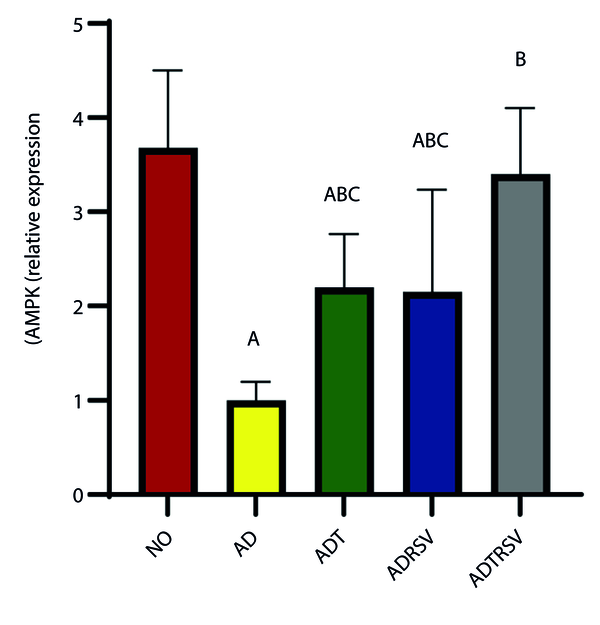
Data analysis also revealed a significant difference in PGC-1α expression in hippocampal tissue between the groups (P = 0.0001, F = 15.940) (Figure 3). Post hoc test results showed a significant decrease in PGC-1α expression in the AD (P = 0.0001), ADT (P = 0.004), and ADRSV (P = 0.004) groups compared to NO. Furthermore, a significant increase in PGC-1α expression was observed in the ADT (P = 0.039), ADRSV (P = 0.037), and ADTRSV (P = 0.0001) groups compared to AD, and in the ADTRSV group compared to the ADT (P = 0.020) and ADRSV (P = 0.021) groups (Figure 3).
Hippocampal PGC-1α expression in different groups by one-way analysis of variance (P < 0.05). A, difference from NO; B, difference from AD; C, difference from ADTRSV. Abbreviations: NO, normal; AD, Alzheimer's; ADT, Alzheimer's-training; ADRSV, Alzheimer's-resveratrol; ADTRSV Alzheimer's-training-resveratrol.
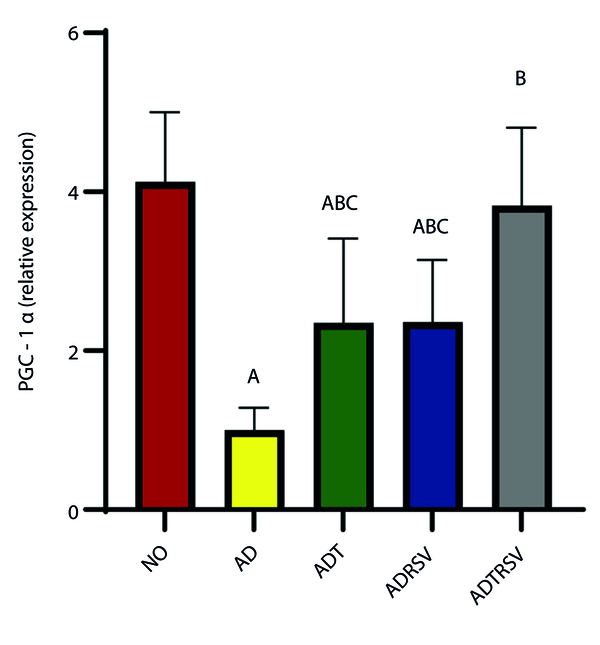
Finally, data analysis showed a significant difference in SIRT1 expression in hippocampal tissue between the groups (P = 0.0001, F = 14.877) (Figure 4). Follow-up test results revealed a significant decrease in SIRT1 expression in the AD (P = 0.0001), ADT (P = 0.026), and ADRSV (P = 0.014) groups compared to NO. A significant increase in SIRT1 expression was observed in the ADT (P = 0.015), ADRSV (P = 0.027), and ADTRSV (P = 0.0001) groups compared to AD, and in the ADTRSV group compared to the ADT (P = 0.038) and ADRSV (P = 0.021) groups (Figure 4).
Hippocampal SIRT1 expression in different groups by one-way analysis of variance (P < 0.05). A, difference from NO; B, difference from AD; C, difference from ADTRSV. Abbreviations: NO, normal; AD, Alzheimer's; ADT, Alzheimer's-training; ADRSV, Alzheimer's-resveratrol; ADTRSV Alzheimer's-training-resveratrol.
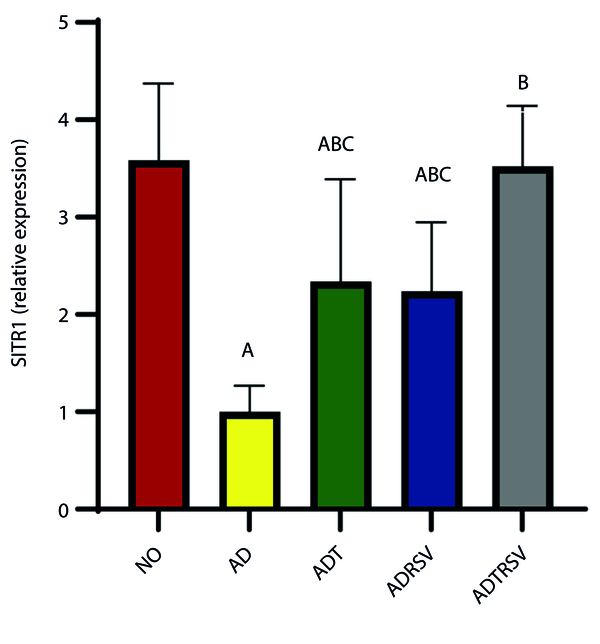
5. Discussion
The results of the present study showed that the induction of AD caused a significant decrease in the expression of AMPK/PGC-1α/SIRT1. The decreased activity of the AMPK/PGC-1α/SIRT1 signaling pathway is closely related to AD pathogenesis. AMPK can activate SIRT1 by increasing levels of nicotinamide adenine dinucleotide (NAD+) in cells, which leads to the activation of PGC-1α. Research has shown that Aβ25-35 inhibits mitochondrial biogenesis in hippocampal neurons by attenuating the AMPK/PGC-1α/SIRT1 signaling pathway (18). In line with the results of the present study, Panes et al. demonstrated in animal models of AD that the expression of SIRT1/PGC-1α decreased, indicating defective mitochondrial biogenesis in AD (19). Jia et al. also observed that AD induction is associated with decreased expression of AMPK, SIRT1, and PGC-1α in the rat hippocampus (20). Studies indicate mitochondrial dysfunction in AD. Mitochondrial dysfunction in the brain tissue of AD patients leads to decreased glucose uptake, reduced activity of enzymes related to the mitochondrial tricarboxylic acid cycle and oxidative phosphorylation, and increased ROS, which causes apoptosis. Increased Aβ accumulation in AD can decrease the activity of AMPK and CaMKKβ while increasing the activity of the ubiquitin-proteasome system. These changes are associated with the degradation of PGC-1α and ultimately impair mitochondrial DNA function in neurons, leading to mitochondrial-dependent cell death (21). Therefore, improving mitochondrial homeostasis to restore normal mitochondrial function is beneficial for preventing or treating AD. Regular exercise is one way to improve mitochondrial function in the AD brain.
The results of the present study showed that exercise training increased the expression of AMPK/PGC-1α/SIRT1 in the hippocampus of AD rats. Recently, Azarian et al. showed that AD induction caused a significant decrease in the expression of the PGC-1α gene in the hippocampal tissue of rats, and that after eight weeks of endurance training, the expression of PGC-1α increased (22). In aged rats, swimming exercise was shown to improve the AMPK/PGC-1α/SIRT1 pathway in hippocampal tissue, thereby suppressing apoptosis and inflammation in the brain (23). Exercise mediates various mitochondrial autophagy pathways through AMPK. One such pathway is AMPK/PGC-1α/SIRT1, where AMPK and SIRT1 are closely related in energy regulation, metabolism, and aging and can enhance each other's activities (24). Nicotinamide adenine dinucleotide increases SIRT1 activity by activating AMPK, leading to the deacetylation of PGC-1α and subsequently activating the AMPK/PGC-1α/SIRT1 signaling pathway. Therefore, exercise improves mitochondrial biogenesis and removes damaged mitochondria through autophagy. Additionally, exercise increases BDNF and its receptor, tyrosine kinase B, activates PKA through the MAPK-2/ERK1 pathway, and ultimately activates cAMP (25). Calcium release following muscle contraction also activates calmodulin, calcineurin, and calmodulin kinase, thereby increasing the activity of SIRT1, PGC-1α, and PPAR (26).
Among the results of the present study, the expression of AMPK, PGC-1α, and SIRT1 increased after RSV consumption in AD rats. Some studies have shown that RSV significantly improves disorders caused by AD through the modulation of various underlying mechanisms and signaling pathways and can slow the onset and progression of AD (27). It has also been demonstrated that pretreatment with RSV improves the antioxidant defense system and increases SIRT1 in AD patients (28). In another study, RSV was shown to improve mitochondrial function by modulating the activation and expression of PGC-1α, which is responsible for mitochondrial biogenesis (29). Mitochondrial biogenesis appears to be induced by SIRT1 with the involvement of AMPK. In SIRT1 knockout mice, daily intake of 25 and 215 mg of RSV had no significant effect on the increase of AMPK, mtDNA content, mitochondrial respiration, or PGC-1α (30). This result suggests that the protective effect of RSV is mediated by its impact on SIRT1. Resveratrol inhibited NF-κB signaling in neurons and protected these cells against microglia-dependent Aβ toxicity. RSV has also been reported to increase mitochondrial mass and function, as well as PGC-1α levels, citrate synthase, and cytochrome c oxidase IV (COXIV) activity, by activating AMPK (31). An in vitro study indicates an ameliorative effect of RSV against neurodegeneration in the brain through the regulation of mitochondrial dynamics and action on AMPK-related pathways (32). Ma et al. conducted a study administering RSV at a dose of 25 mg/kg in Wistar diabetic AD model rats, showing that RSV could increase the level of SIRT1 (33).
Another finding of the present study was the increased expression of AMPK, PGC-1α, and SIRT1 in the ADTRSV group compared to other experimental groups. As mentioned, both exercise training (21, 22) and RSV (28, 30-32) can increase the expression of AMPK, PGC-1α, and SIRT1 in various mouse models (AD, aging, and diabetes). It seems that RSV can enhance the effects of exercise on these indicators, leading to synergistic results. Liao et al. showed that exercise, RSV, and their combination increased the expression of AMPK and SIRT1, while decreasing the expression of P53 and the Bax/Bcl-2 ratio in aged rats (34). Another study indicated that the combination of exercise and RSV could cause synergistic activation of SIRT1 and PI3K/Akt signaling in the heart tissue of old rats (35).
One limitation of the present study is the lack of investigation of the AMP/ATP ratio and the NAD+/NADH ratio, which are crucial factors for AMPK activation following exercise. Additionally, since the onset of AD occurs over a long period, induction of AD using Aβ may not fully reveal the disease's symptoms.
The results of the present study indicated that AD induction decreased the expression of AMPK/PGC-1α/SIRT1, while aerobic exercise improved the expression of these indicators in the hippocampus of AD rats. RSV also positively affected this signaling pathway. However, the combined effect of exercise and RSV on this pathway was greater than the effect of each intervention alone, indicating a synergistic effect. Therefore, based on the results of the present study and previous research, it is recommended to use both exercise and RSV supplementation to improve mitochondrial function and metabolic status in AD, potentially reducing or delaying the problems caused by AD by enhancing cellular function in the central nervous system.
References
-
1.
Rudnicka E, Napierala P, Podfigurna A, Meczekalski B, Smolarczyk R, Grymowicz M. The World Health Organization (WHO) approach to healthy ageing. Maturitas. 2020;139:6-11. [PubMed ID: 32747042]. [PubMed Central ID: PMC7250103]. https://doi.org/10.1016/j.maturitas.2020.05.018.
-
2.
Hou Y, Dan X, Babbar M, Wei Y, Hasselbalch SG, Croteau DL, et al. Ageing as a risk factor for neurodegenerative disease. Nat Rev Neurol. 2019;15(10):565-81. [PubMed ID: 31501588]. https://doi.org/10.1038/s41582-019-0244-7.
-
3.
Ju Y, Tam KY. Pathological mechanisms and therapeutic strategies for Alzheimer's disease. Neural Regen Res. 2022;17(3):543-9. [PubMed ID: 34380884]. [PubMed Central ID: PMC8504384]. https://doi.org/10.4103/1673-5374.320970.
-
4.
Cioffi F, Adam RHI, Broersen K. Molecular mechanisms and genetics of oxidative stress in alzheimer's disease. J Alzheimers Dis. 2019;72(4):981-1017. [PubMed ID: 31744008]. [PubMed Central ID: PMC6971833]. https://doi.org/10.3233/JAD-190863.
-
5.
Yoon SS, Jo SA. Mechanisms of amyloid-beta peptide clearance: Potential therapeutic targets for alzheimer's disease. Biomol Ther (Seoul). 2012;20(3):245-55. [PubMed ID: 24130920]. [PubMed Central ID: PMC3794520]. https://doi.org/10.4062/biomolther.2012.20.3.245.
-
6.
Jeon SM. Regulation and function of AMPK in physiology and diseases. Exp Mol Med. 2016;48(7). e245. [PubMed ID: 27416781]. [PubMed Central ID: PMC4973318]. https://doi.org/10.1038/emm.2016.81.
-
7.
Kjobsted R, Hingst JR, Fentz J, Foretz M, Sanz MN, Pehmoller C, et al. AMPK in skeletal muscle function and metabolism. FASEB J. 2018;32(4):1741-77. [PubMed ID: 29242278]. [PubMed Central ID: PMC5945561]. https://doi.org/10.1096/fj.201700442R.
-
8.
Koo JH, Kang EB, Oh YS, Yang DS, Cho JY. Treadmill exercise decreases amyloid-beta burden possibly via activation of SIRT-1 signaling in a mouse model of Alzheimer's disease. Exp Neurol. 2017;288:142-52. [PubMed ID: 27889467]. https://doi.org/10.1016/j.expneurol.2016.11.014.
-
9.
Cicero AFG, Ruscica M, Banach M. Resveratrol and cognitive decline: A clinician perspective. Arch Med Sci. 2019;15(4):936-43. [PubMed ID: 31360188]. [PubMed Central ID: PMC6657254]. https://doi.org/10.5114/aoms.2019.85463.
-
10.
Moussa C, Hebron M, Huang X, Ahn J, Rissman RA, Aisen PS, et al. Resveratrol regulates neuro-inflammation and induces adaptive immunity in Alzheimer's disease. J Neuroinflammation. 2017;14(1):1. [PubMed ID: 28086917]. [PubMed Central ID: PMC5234138]. https://doi.org/10.1186/s12974-016-0779-0.
-
11.
Witte AV, Kerti L, Margulies DS, Floel A. Effects of resveratrol on memory performance, hippocampal functional connectivity, and glucose metabolism in healthy older adults. J Neurosci. 2014;34(23):7862-70. [PubMed ID: 24899709]. [PubMed Central ID: PMC6608268]. https://doi.org/10.1523/JNEUROSCI.0385-14.2014.
-
12.
Houtkooper RH, Pirinen E, Auwerx J. Sirtuins as regulators of metabolism and healthspan. Nat Rev Mol Cell Biol. 2012;13(4):225-38. [PubMed ID: 22395773]. [PubMed Central ID: PMC4872805]. https://doi.org/10.1038/nrm3293.
-
13.
Broderick TL, Rasool S, Li R, Zhang Y, Anderson M, Al-Nakkash L, et al. Neuroprotective effects of chronic resveratrol treatment and exercise training in the 3xTg-AD mouse model of alzheimer's disease. Int J Mol Sci. 2020;21(19). [PubMed ID: 33020412]. [PubMed Central ID: PMC7582460]. https://doi.org/10.3390/ijms21197337.
-
14.
Allard J, Graham-Hyatt S, Mulgrave V, Alsayegh A. Exercise-induced upregulation of APOE expression is attenuated by dietary supplementation with resveratrol. Physiology. 2023;38(S1):5735198. https://doi.org/10.1152/physiol.2023.38.S1.5735198.
-
15.
Eslimi Esfehani D, Oryan S, Khosravi M, Valizadegan F. Effect of fennel extract on the improvement of memory disorders in beta amyloid alzheimer model of male wistar rats. J Ilam Univ Med Sci. 2019;27(1):1-12. https://doi.org/10.29252/sjimu.27.1.1.
-
16.
Monserrat Hernandez-Hernandez E, Serrano-Garcia C, Antonio Vazquez-Roque R, Diaz A, Monroy E, Rodriguez-Moreno A, et al. Chronic administration of resveratrol prevents morphological changes in prefrontal cortex and hippocampus of aged rats. Synapse. 2016;70(5):206-17. [PubMed ID: 26789275]. https://doi.org/10.1002/syn.21888.
-
17.
Zhao HF, Li N, Wang Q, Cheng XJ, Li XM, Liu TT. Resveratrol decreases the insoluble Abeta1-42 level in hippocampus and protects the integrity of the blood-brain barrier in AD rats. Neuroscience. 2015;310:641-9. [PubMed ID: 26454022]. https://doi.org/10.1016/j.neuroscience.2015.10.006.
-
18.
Dong W, Wang F, Guo W, Zheng X, Chen Y, Zhang W, et al. Abeta25-35 suppresses mitochondrial biogenesis in primary hippocampal neurons. Cell Mol Neurobiol. 2016;36(1):83-91. [PubMed ID: 26055049]. https://doi.org/10.1007/s10571-015-0222-6.
-
19.
Panes JD, Godoy PA, Silva-Grecchi T, Celis MT, Ramirez-Molina O, Gavilan J, et al. Changes in PGC-1alpha/SIRT1 signaling impact on mitochondrial homeostasis in amyloid-beta peptide toxicity model. Front Pharmacol. 2020;11:709. [PubMed ID: 32523530]. [PubMed Central ID: PMC7261959]. https://doi.org/10.3389/fphar.2020.00709.
-
20.
Jia J, Yin J, Zhang Y, Xu G, Wang M, Jiang H, et al. Thioredoxin-1 promotes mitochondrial biogenesis through regulating AMPK/Sirt1/PGC1alpha pathway in alzheimer's disease. ASN Neuro. 2023;15:17590914231159200. [PubMed ID: 36823760]. [PubMed Central ID: PMC9969465]. https://doi.org/10.1177/17590914231159226.
-
21.
Silva P, Vauzour D. Wine polyphenols and neurodegenerative diseases: An update on the molecular mechanisms underpinning their protective effects. Beverages. 2018;4(4):96. https://doi.org/10.3390/beverages4040096.
-
22.
Azarian F, Farsi S, Hosseini SA, Azarbayjani MA. Effect of endurance training with saffron consumption on PGC1-α gene expression in hippocampus tissue of rats with Alzheimer’s disease. Ann Mil Health Sci Res. 2020;18(1). e99131. https://doi.org/10.5812/amh.99131.
-
23.
Lin JY, Kuo WW, Baskaran R, Kuo CH, Chen YA, Chen WS, et al. Swimming exercise stimulates IGF1/ PI3K/Akt and AMPK/SIRT1/PGC1alpha survival signaling to suppress apoptosis and inflammation in aging hippocampus. Aging (Albany NY). 2020;12(8):6852-64. [PubMed ID: 32320382]. [PubMed Central ID: PMC7202519]. https://doi.org/10.18632/aging.103046.
-
24.
Sung B, Chung JW, Bae HR, Choi JS, Kim CM, Kim ND. Humulus japonicus extract exhibits antioxidative and anti-aging effects via modulation of the AMPK-SIRT1 pathway. Exp Ther Med. 2015;9(5):1819-26. [PubMed ID: 26136899]. [PubMed Central ID: PMC4471809]. https://doi.org/10.3892/etm.2015.2302.
-
25.
Skandari M, Nazemzadegan GH, Daryanosh F, Samadi M, Honarpisheh S, Hasanpor M. [Comparative effect of single bout of continuous endurance and high intensity interval exercise on serum BDNF in rat]. Feyz Medical Sciences Journal. 2016;20(2):141-6. FA.
-
26.
Ghasemi E, Afzalpour ME, Zarban A. [The effects of 10 weeks of high-intensity interval training and green tea supplementation on serum levels of sirtuin 1 and catalase in overweight women]. Sport Physiology. 2016;8(32):169-84. FA. https://doi.org/10.1016/j.scispo.2016.09.004.
-
27.
Ahmed T, Javed S, Javed S, Tariq A, Samec D, Tejada S, et al. Resveratrol and alzheimer's disease: Mechanistic insights. Mol Neurobiol. 2017;54(4):2622-35. [PubMed ID: 26993301]. https://doi.org/10.1007/s12035-016-9839-9.
-
28.
Cosin-Tomas M, Senserrich J, Arumi-Planas M, Alquezar C, Pallas M, Martin-Requero A, et al. Role of resveratrol and selenium on oxidative stress and expression of antioxidant and anti-aging genes in immortalized lymphocytes from alzheimer's disease patients. Nutrients. 2019;11(8). [PubMed ID: 31370365]. [PubMed Central ID: PMC6723840]. https://doi.org/10.3390/nu11081764.
-
29.
Scarpulla RC. Metabolic control of mitochondrial biogenesis through the PGC-1 family regulatory network. Biochim Biophys Acta. 2011;1813(7):1269-78. [PubMed ID: 20933024]. [PubMed Central ID: PMC3035754]. https://doi.org/10.1016/j.bbamcr.2010.09.019.
-
30.
Price NL, Gomes AP, Ling AJ, Duarte FV, Martin-Montalvo A, North BJ, et al. SIRT1 is required for AMPK activation and the beneficial effects of resveratrol on mitochondrial function. Cell Metab. 2012;15(5):675-90. [PubMed ID: 22560220]. [PubMed Central ID: PMC3545644]. https://doi.org/10.1016/j.cmet.2012.04.003.
-
31.
Yang AJT, Bagit A, MacPherson REK. Resveratrol, metabolic dysregulation, and alzheimer's disease: Considerations for neurogenerative disease. Int J Mol Sci. 2021;22(9). [PubMed ID: 33924876]. [PubMed Central ID: PMC8125227]. https://doi.org/10.3390/ijms22094628.
-
32.
Gao J, Wang H, Li Y, Li W. Resveratrol attenuates cerebral ischaemia reperfusion injury via modulating mitochondrial dynamics homeostasis and activating AMPK-Mfn1 pathway. Int J Exp Pathol. 2019;100(5-6):337-49. [PubMed ID: 31867811]. [PubMed Central ID: PMC7042761]. https://doi.org/10.1111/iep.12336.
-
33.
Ma X, Sun Z, Han X, Li S, Jiang X, Chen S, et al. Neuroprotective effect of resveratrol via activation of Sirt1 signaling in a rat model of combined diabetes and alzheimer's disease. Front Neurosci. 2019;13:1400. [PubMed ID: 32038127]. [PubMed Central ID: PMC6985467]. https://doi.org/10.3389/fnins.2019.01400.
-
34.
Liao ZY, Chen JL, Xiao MH, Sun Y, Zhao YX, Pu D, et al. The effect of exercise, resveratrol or their combination on Sarcopenia in aged rats via regulation of AMPK/Sirt1 pathway. Exp Gerontol. 2017;98:177-83. [PubMed ID: 28847722]. https://doi.org/10.1016/j.exger.2017.08.032.
-
35.
Lin CH, Lin CC, Ting WJ, Pai PY, Kuo CH, Ho TJ, et al. Resveratrol enhanced FOXO3 phosphorylation via synergetic activation of SIRT1 and PI3K/Akt signaling to improve the effects of exercise in elderly rat hearts. Age (Dordr). 2014;36(5):9705. [PubMed ID: 25158994]. [PubMed Central ID: PMC4453936]. https://doi.org/10.1007/s11357-014-9705-5.