Abstract
Keywords
Middle-Age Exercise Cardiac Hypertrophy Pathologic Physiologic
1. Context
Aging is associated with the development of various cardiovascular disorders, such as coronary artery disease, hypertension and heart failure. Every decade of aging affects the integrity of the cardiovascular system even in the absence of pathological factors. These changes in cardiovascular physiology due to aging are different from pathology, which also increases during aging (1, 2). Evidence suggests that the aging process significantly affects the structure and function of the cardiovascular system, as the middle age and old age are followed by changes in the heart muscle. Variations in the functioning of the cardiomyocytes are central factors in aging-dependent changes, because they play an important role in cardiovascular hemodynamics. Aging influences the functioning of the cardiomyocytes at different levels, so that aging has a direct impact on calcium homeostasis, cardiac muscle contraction, and paired stimulation of contractile and cellular integrity of cardiomyocyte organelles (3, 4). These factors are necessary in the neohumoral regulation of cardiomyocyte function through adrenergic and renin-angiotensin systems (5-9). In addition, middle age and old age cause changes in the components and quality of extracellular matrices, affecting not only the structures of the cardiomyocytes, but also the cardiac function (10, 11). Cardiac hypertrophy is one of the key variations in the cardiac structure linked to aging that is caused by various underlying factors (12).
2. Effects of Aging on the Heart
The calendar age is a process dependent on time. For this reason, middle age and old age are considered to be in line with the increase in mortality, although the calendar age is not regarded to be a factor indicating an individual’s health. On the contrary, biological age is used as one of the measures of human health assessment. The term “functional aging” or “functional middle age” is defined by emphasizing intrinsic constraints in describing an individual’s health while taking into account the calendar age. This principle is based on whatever that humans can do in relation to others in society; however, it may be expanded to include indicators, such as the level of functional abilities maintained by tissues and organs in older ages. Finally, the concept of “successful middle age and successful aging” represents the process resulting from the balance between winning and losing (12, 13).
Based on this concept, the structural and functional changes of the heart that occur with aging in healthy people can be interpreted as a type of adaptation to vascular changes occurring during middle age. Middle age results in processes such as vascular stiffness and increased systolic pressure in vessels that together with increased peripheral vascular resistance can lead to aortic dilatation and vessel wall thickness. This, in turn, causes an increase in the ventricular wall thickness, the death of myocytes and the deposition of collagen (14, 15). At rest, the cardiac pump function remains constant through prolonged contractions, but this prevents complete myocardial rest and causes a decrease in the initial ventricular filling rate. The cardiac implants over-regulate to compensate for abnormal filling and prevent diminished end-diastolic volume, including enlargement of the left atrium and an increase in the participation of the atrium in the ventricular filling (14).
In sum, these adaptations are established in response to reduced heart storage due to middle age. The changes in cardiovascular storage are inadequate to create clinical heart failure, but these factors also affect the signs, symptoms, severity, and prognosis of heart failure that can be caused by any reason. The heart of a middle-aged or elderly person has a greater sensitivity to harmful effects and external factors, including increased vascular afterload and disproportionate arterial-ventricular load, as well as internal factors, including a reduction in cardiomyocyte contraction and decreased ability of the cardiomyocytes to respond to stress (16).
3. Cardiac Hypertrophy
The mammalian cardiomyocytes are generally withdrawn from the cell cycle shortly after birth. Therefore, cardiomyocytes are often found during the late stage of differentiation during middle age and do not proliferate under physiological conditions. The heart tissues show the flexibility that can empower the heart; under these conditions, it responds to environmental requirements, and the cells can grow or shrink and be destroyed through exposure to pathological and physiological stresses. Cardiac hypertrophy is subdivided into the physiological type that accompanies increased normal cardiac function and the pathological type associated with heart failure (17).
The increase in normal cardiac output mainly occurs through hypertrophy of the cardiomyocytes in response to body enlargement or exercise training. The enlarged cardiomyocytes receive adequate nutrition through the expansion of the capillary network, but abnormal cardiac functional and structural enlargement do not occur in this way. For this reason, physiological hypertrophy generally is not considered to be a risk factor for heart failure. In contrast, pathological hypertrophy is associated with the production of high levels of neurohumoral mediators, hemodynamic overload, damage, and loss of cardiomyocytes. In pathological regulation, the growth of cardiomyocytes exceeds the capacity of capillaries to supply nutrition and oxygen, leading to cardiac hypoxia and pathological remodeling in rodents (18, 19). Since cardiac hypertrophy plays a pivotal role in cardiac remodeling and is an independent factor for cardiac events, it is very important to understand this process. Previous studies have indicated that heart failure is associated with a complex range of pathological changes, including capillary expansion, metabolic disorders, sarcomeric irregularities, changes in calcium transport, inflammation, cellular aging, cell death and fibrosis. There is also some overlap between the mechanisms of physiological and pathological hypertrophies (17).
Accordingly, heart failure can be categorized into two types: heart failure with reduced ejection fraction (HFrEF) and heart failure with preserved ejection fraction (HFpEF). HFrEF is developed through the accumulation of myocardial damages and the upward trend of losing cardiomyocytes, and it occurs typically in response to myocardial infarction, hypertension or cardiomyopathy. Furthermore, the oxidative stress present within the cardiomyocytes induces cardiomyocyte death and replacement fibrosis (20, 21). Losing the cardiomyocytes causes increased changes in the extracellular matrix and participation in left ventricular dilatation and left ventricular eccentric remodeling (22).
It is estimated that 50% of patients with heart disease have HFpEF. In addition, concentric remodeling and left ventricular diastolic dysfunction can also be seen in these individuals. Obese or overweight people with hypertension, diabetes mellitus, chronic pulmonary disease, anemia and chronic kidney diseases may experience systemic inflammation; these systemic diseases increase the risk of HFpEF. The HFpEF structural changes are detectable through the hypertrophy of cardiomyocytes, interstitial fibrosis, and functional changes (23-26). Replacement fibrosis does not usually develop in HfpEF because cell death is not predictably increased in the HFpEF (27).
3.1. Types of Cardiac Hypertrophy
The heart has the ability to respond to environmental conditions and it is able to grow or shrink. Depending on the strength and duration of stimulation, the heart size can increase, which can be categorized into two types of hypertrophy: pathological and physiological. The physiological hypertrophy is characterized by normal or incremental levels in contractile function and the normal organization of the heart structure (28). The pathological hypertrophy is also associated with increased cell death and fibrosis remodeling and can be detected by reductions in systolic and diastolic functions, which often lead to heart failure. The stimuli result in various cellular responses, including gene expression, protein synthesis, sarcomeric accumulation, cell metabolism and developed cardiac hypertrophy (29-31).
The collected documents suggest differences in pathological and physiological hypertrophies in terms of signaling pathways. Additionally, the pathological cardiac hypertrophy, especially in the left ventricle, has short-term benefits and long-term risks although the mechanism regulating this transition from compatibility to hypertrophic abnormality has not yet been specified (32).
According to heart geometry, cardiac hypertrophy is subjected to different types, including eccentric and concentric. Eccentric hypertrophy is developed by volume overload and non-pathological eccentric hypertrophy that is characterized by an increase in ventricular volume, wall and septal thickness. Pathological eccentric hypertrophy commonly develops after heart diseases, such as myocardial infarction and dilated cardiomyopathy, resulting in the dilatation of ventricles and the elongation of cardiomyocytes. Concentric hypertrophy is associated with an increase in the wall and septal thickness and a decrease in the left ventricular dimensions (24, 33, 34). The cardiomyocytes usually increase in thickness more than in length; this develops under pathological conditions such as high blood pressure and vascular disease, although some regulators, like wrestling, cause induction of non-pathological eccentric hypertrophy (35)
3.2. Pathological Concentric and Eccentric Hypertrophies
Left ventricular concentric hypertrophy occurs due to diseases such as hypertension (even without a specific disease), which enhance the risk of cardiovascular progression with high-risk levels of death from cardiovascular disease (35, 36). In accordance with the evidence, some degree of stress in the ventricular end-diastolic wall acts as a signal regulating hypertrophy. This can lead to a greater induction of left ventricular concentric hypertrophy in patients with hypertension and low levels of ventricular end-diastolic wall pressure, which are characterized by a significant increase in the relative wall thickness (37, 38). The cardiomyocytes existing in myocardial concentric remodeling that have excessive thickness show an increase in diameters, but have no significant elevation in length, significantly reducing the mean length to width (L/W) ratio (37). This phenomenon occurs because of the orientation in the contractile sarcomeric units that add to the cardiomyocytes.
In a heart that has afterload pressure, the sarcomeres are added in parallel, which shows how they change the L/W ratio (39, 40). This process is evident as the muscle wall enlargement remains unchanged in the dimensions of the ventricular chambers although the dimensions of the posterior left ventricular wall are significantly increased during systolic and diastolic periods. Therefore, the common consequence associated with concentric hypertrophy is increased ventricular diastolic stiffness, which causes cardiac dysfunction (41).
Pathological cardiac growth, especially eccentric hypertrophy, is caused by an increase in preload, including valve failure or increased volume overload (37). In rats with myocardial infarction, 77% of the animals with eccentric cardiac remodeling suffered from systolic dysfunction. Comparing the length and width of the cardiomyocytes showed that the L/W ratio was unchanged, as in a normal heart. This is due to the thickness and length of the cells. The mechanism of this total increase in size is due to the parallel addition of sarcomeres in a row and in response to an increase in volume overloads (37). In addition, when evaluating specific left ventricular parameters, the researchers found that the internal diameter was increased in rats with increased volume overload while the interventricular septum or the dimensions of the posterior left ventricular wall had no changes (42).
It is clear that middle-aged or old-aged people who live a sedentary life are somehow faced with pathological concentric hypertrophy. Existing evidence also suggests that underlying diseases in these individuals, including hypertension, vascular wall stiffness, and other diseases, can cause concentric hypertrophy (43) (Figure 1).
Pathological eccentric and concentric hypertrophy (43)
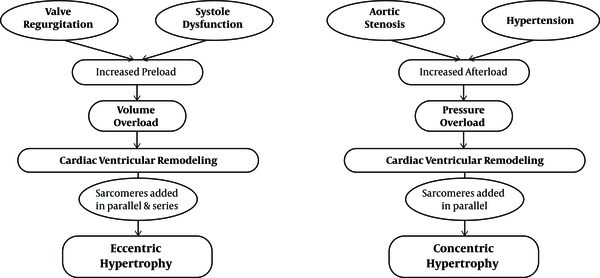
3.3. Middle-Aged Effect on Cardiac Responses to Exercise Training
Exercise training imposes physiological stress on the body, which requires responses coordinated by the cardiovascular, respiratory and nervous systems to increase blood flow and supply oxygen for the skeletal muscles. At rest, the muscles account for approximately 20% of the total blood flow, but this can be increased up to 80% during exercise training. Therefore, dysfunction in one of these systems can lead to a significant decrease in the maximum cardiac output during total exercise training (44).
It is well-defined that heart involvement contributes to increased cardiac output in response to the high metabolic requirements of exercise training and this is essentially dependent on the dynamic regulations of two physiological parameters, namely, heart rate (HR) and stroke volume (SV). In healthy adults, the adrenergic stimulation caused by exercise training rapidly increases heart rate and stroke volume. The stroke volume increases primarily by increasing myocardial contractions and reducing the peripheral vascular resistance. Elevated stroke volume increases by up to 40–50% of the maximum capacity corresponding to the intensity of exercise training, then reaches a plateau, and further reinforcement in output proceeds proportional to the increase in heart rate (45).
While older adults can still increase their cardiac output in response to exercise training, the relative increase is usually reduced compared with younger people. The decrease in maximum heart rate, also known as chronotropic incompetence or disorder, is the main factor in reducing cardiac response to exercise training in adults. Natural aging results in a progressive decrease in the maximum heart rate of about 0.7 beats/minute/year (46). Although the mechanism of this chronotropic incompetence has not yet been specified, degenerative changes in the transmission system associated with autonomic dysregulation may play a major role. Most importantly, the decrease in the peak HR due to middle age has a strong association with a reduction in exercise training capacity, and this is an independent predictor of cardiovascular events and mortality (47-49).
The impact of middle age and aging on the enhancement of SV via exercise training has not yet been clearly demonstrated. Generally, the heart of older adults still has the ability to increase SV in response to exercise training (albeit at insufficient levels of compensation in reducing peak beats). The mechanism by which heart SV can be increased by exercise training may be altered with the age of the individual. However, the increase in myocardial contractions is considered to be the main measure for increasing SV in the hearts of young people. Enhanced SV due to exercise training in the heart of older adults is mainly associated with the elevation in end-diastolic volume or a slight change in contractions (50).
In general, normal middle age significantly reduces both chronotropic and inotropic responses of the heart to exercise training. Clinically, this phenomenon refers to the storage disorders of the heart, which shows the inability of the heart to strengthen the cardiac output in response to the increased need for physiological stresses, possibly due to exercise training or specific medications (51). Concomitant with middle-age-induced changes in the mechanisms of peripheral oxygen delivery and consumption in skeletal muscle, inadequate oxygen transfer from impaired cardiac storage is a major factor in reducing functional capacity in adults, especially in patients with heart disease (52-56). Maximal oxygen consumption is the standard method of measuring exercise training capacity. During normal middle age, VO2 max is reduced down to about 10 per decade in active healthy people, but this decreases in people over 70 years and among people with heart disease it is more accelerated (9, 57). This process shows that the mechanism leading to storage disorders of the heart during middle age may be due to an increased risk of heart disease by age (Table 1) (51).
Some Cardiovascular Changes in Middle Age (51)
CV Parameter at Park Exercise | Effects of Aging |
---|---|
Cardiac output | ↓ / NC |
Heart rate | ↓ |
LV stroke volume | ↑ / ↓ / NC |
LV end-diastolic volume | ↑ |
LV contractility | ↓ |
Early diastolic filling rate | ↓ |
VO2 max | ↓ |
(A-V) O2 difference | ↓ |
3.4. Physiological Hypertrophy
Similar to pathological hypertrophy, physiological hypertrophy is also a response of the heart to the strains caused by eccentric and concentric remodeling. Increased volume overload can mainly cause left ventricular dilatation, which can also be caused by endurance physical activities (58-60). Some researchers have demonstrated that the hearts of animals participating in endurance training was accompanied by the addition of new sarcomeres into a series of existing sarcomeres that previously had pathological cardiac hypertrophy (61). On the other hand, physiological concentric remodeling occurs due to increased pressure overload, which is caused by participating in resistance training programs. It is characterized by increasing myocardial volume and wall thickness without changing the size of the chambers (59, 60, 62). In resistance training programs, there is a significant increase in systolic blood pressure, and the sarcomeres are added in parallel to existing sarcomeres, increasing the thickness of the walls in a similar manner to pathological hypertrophy (63, 64). Interestingly, combined physical activities involving both pressure overload and volume overload, which have both aspects of endurance physical activities (running and swimming) along with resistance physical activities can lead to combined hypertrophy (65). Differences in the types of physiological stimuli can be observed in the differences in the types of hypertrophy. Right ventricular remodeling can also occur with endurance exercise training, although left ventricular dilation is caused by an increase in both systolic and diastolic functions (66).
The physiological cardiac remodeling in athletes is not associated with interstitial fibrosis, as occurs in pathological hypertrophy (67). In addition, a study found that exercise training prevents myocardial inflammation induced by isoproterenol injection (67). Moreover, exercise training can prevent myocardial deficiencies. It should be noted that inhibiting myocardial hypertrophy by exercise training could lead to lower NFkB expression (68). Physiological remodeling is a response to high exercise volume, reflecting the importance of exercise training in morphological changes in the heart60 (Figures 2 and 3).
Resistance, endurance and combination exercises and their effect on cardiac hypertrophy (43)
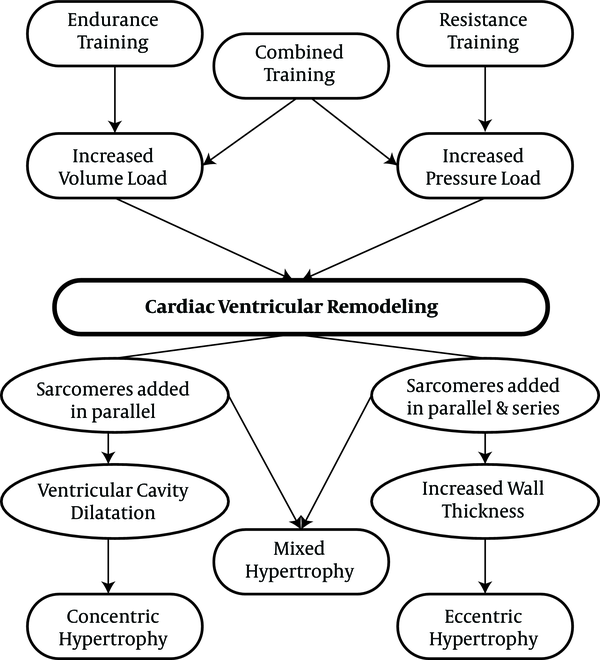
The effect of aerobic and resistance training on cardiac hypertrophy (69)
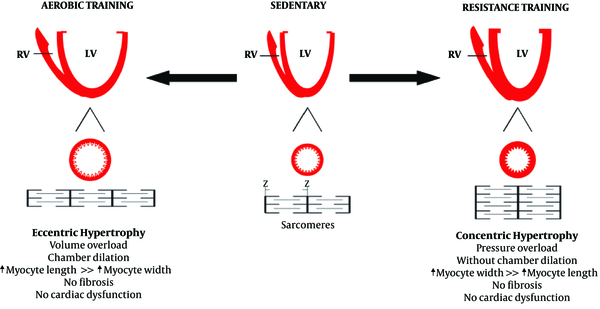
3.5. Effect of Exercise Training on Middle-Aged Hypertrophy
The distinctive concept of cardiac hypertrophy is particularly relevant to adult hearts. Contrary to findings in young animals, aerobic exercises generally cause some degree of cardiac hypertrophy (70). Studies based on exercise training of adults and older people have shown a wide variety of cardiac development in response to exercise training (71-76), with some studies indicating reversal of middle-aged hypertrophy through exercise training. One of these studies has evaluated the effect of exercise training on the growth of cardiomyocytes in the heart of adults. Kwak et al. trained young and adult rats at 75% VO2 max for 12 weeks. Their findings indicated that the training induced the hypertrophy of cardiomyocytes in young rats. These results were associated with a regression in the size of cardiomyocytes (69% reduction in cross-sectional surface area) in adult samples (74). Alternatively, in another study, training on a treadmill or with swimming at low to moderate intensity in adult Wistar rats did not affect the size of the cardiomyocytes. Differences in training periods and age of animal samples may result in different findings. In addition, only a small number of studies investigated the decrease in blood pressure via exercise training in adult animal specimens (75, 77).
It is not surprising that the molecular basis for the different potential effects of growth due to exercise training in adult heart samples is not well defined. Obviously, the cytoprotective effects of exercise training may improve the survival of adult hearts. Therefore, stimuli for active pathological hypertrophy are reduced. In fact, trained adult rat hearts showed a decrease in several apoptotic indexes, which usually rises in the hearts of adults. However, it has not been completely determined as to what the mechanisms of these changes induced by exercise training are, including decreased translation of factors for cell death and inhibiting pathological growth stimuli in the heart of older adults (74, 76, 78).
The signaling mechanism of exercise training, which potentially improves the cardiomyocytes in adults, might be related to cardioprotective effects of the IGF1/PI3K/Akt pathway. Increasing expression of cardiac IGF1, PI3K and Akt1 is indicative of improved survival of cardiomyocytes in adult rats exposed to ischemic damages (28, 36, 79). More importantly, several studies have shown that similar to young animal specimens, aerobic exercise training causes increased Akt phosphorylation in the heart of adult and old rats, although its level is low (77, 78, 80). Although low levels of Akt activity in the heart of trained rats is sufficient to increase cell survival and decrease pathological growth, it is not enough to develop physiological growth (51). Finally, the variation in cardiac growth responses to exercise training among young and adult animals is probably related to fundamental differences in the substrates between the young and the adults and the pathological hypertrophy pathways and apoptosis. Indeed, when young and adult rats participated in the same 12-week training, it was found that the levels of MAPK and calcineurin/NFAT signaling were decreased in the hearts of adults and did not change in the hearts of the youth, or the changes were not significant, although Akt was increased in both groups (78, 80). In this regard, some studies have shown that Akt causes inhibition of MAPK pathways that participate in the development of hypertrophy (81).
Although the role of exercise training through other mechanisms has been less considered, including changes in anti-aging hormones and oxidative stress, MAPKs may be involved in the hypertrophy of the middle-aged heart. In this context, it has also been reported that exercise training can be effective in improving age-related mitochondrial disorders (82, 83).
4. Conclusions
According to these findings, middle age along with physical inactivity seems to be associated with pathological hypertrophy, during which the intraventricular volume decreases and the heart wall thickness increases. However, exercise training through various mechanisms creates the possibility of driving pathological hypertrophy towards physiological hypertrophy. In addition, the type and duration of the exercise training can influence the respective effects.
References
-
1.
Czuriga D, Papp Z, Czuriga I, Balogh A. Cardiac aging-a review. Eur Surg Res. 2011;43(2):69-77. https://doi.org/10.1007/s10353-011-0600-3.
-
2.
Lee HY, Oh BH. Aging and arterial stiffness. CIRC J. 2010;74(11):2257-62. https://doi.org/10.1253/circj.CJ-10-0910.
-
3.
Howlett KF, Sakamoto K, Hirshman MF, Aschenbach WG, Dow M, White MF, et al. Insulin signaling after exercise in insulin receptor substrate-2-deficient mice. Diabetes. 2002;51(2):479-83. [PubMed ID: 11812758].
-
4.
Rosen BD, Fernandes VR, Nasir K, Helle-Valle T, Jerosch-Herold M, Bluemke DA, et al. Age, increased left ventricular mass, and lower regional myocardial perfusion are related to greater extent of myocardial dyssynchrony in asymptomatic individuals: the multi-ethnic study of atherosclerosis. Circulation. 2009;120(10):859-66. [PubMed ID: 19704101]. [PubMed Central ID: PMC2751872]. https://doi.org/10.1161/CIRCULATIONAHA.108.787408.
-
5.
Dai DF, Rabinovitch PS. Cardiac aging in mice and humans: the role of mitochondrial oxidative stress. Trends Cardiovasc Med. 2009;19(7):213-20. [PubMed ID: 20382344]. [PubMed Central ID: PMC2858758]. https://doi.org/10.1016/j.tcm.2009.12.004.
-
6.
De Meyer GR, De Keulenaer GW, Martinet W. Role of autophagy in heart failure associated with aging. Heart Fail Rev. 2010;15(5):423-30. [PubMed ID: 20383579]. https://doi.org/10.1007/s10741-010-9166-6.
-
7.
Hotta H, Uchida S. Aging of the autonomic nervous system and possible improvements in autonomic activity using somatic afferent stimulation. Geriatr Gerontol Int. 2010;10 Suppl 1:S127-36. [PubMed ID: 20590828]. https://doi.org/10.1111/j.1447-0594.2010.00592.x.
-
8.
Benigni A, Cassis P, Remuzzi G. Angiotensin II revisited: new roles in inflammation, immunology and aging. EMBO Mol Med. 2010;2(7):247-57. [PubMed ID: 20597104]. [PubMed Central ID: PMC3377325]. https://doi.org/10.1002/emmm.201000080.
-
9.
Tartibian B, Botelho Teixeira AM, Baghaiee B. Moderate intensity exercise is associated with decreased angiotensin-converting enzyme, increased beta2-adrenergic receptor gene expression, and lower blood pressure in middle-aged men. J Aging Phys Act. 2015;23(2):212-20. [PubMed ID: 24809305]. https://doi.org/10.1123/japa.2013-0136.
-
10.
Ma Y, Chiao YA, Zhang J, Manicone AM, Jin YF, Lindsey ML. Matrix metalloproteinase-28 deletion amplifies inflammatory and extracellular matrix responses to cardiac aging. Microsc Microanal. 2012;18(1):81-90. [PubMed ID: 22153350]. [PubMed Central ID: PMC3972008]. https://doi.org/10.1017/S1431927611012220.
-
11.
Neilan TG, Coelho-Filho OR, Shah RV, Abbasi SA, Heydari B, Watanabe E, et al. Myocardial extracellular volume fraction from T1 measurements in healthy volunteers and mice: relationship to aging and cardiac dimensions. JACC Cardiovasc Imaging. 2013;6(6):672-83. [PubMed ID: 23643283]. [PubMed Central ID: PMC3683385]. https://doi.org/10.1016/j.jcmg.2012.09.020.
-
12.
Lakatta EG. Arterial and cardiac aging: major shareholders in cardiovascular disease enterprises: Part III: cellular and molecular clues to heart and arterial aging. Circulation. 2003;107(3):490-7. [PubMed ID: 12551876].
-
13.
Anton B, Vitetta L, Cortizo F, Sali A. Can we delay aging? The biology and science of aging. Ann N Y Acad Sci. 2005;1057:525-35. [PubMed ID: 16399917]. https://doi.org/10.1196/annals.1356.040.
-
14.
Lakatta EG, Levy D. Arterial and cardiac aging: major shareholders in cardiovascular disease enterprises: Part I: aging arteries: a "set up" for vascular disease. Circulation. 2003;107(1):139-46. [PubMed ID: 12515756].
-
15.
Baghaiee B, Siahkuhian M, Hakimi M, Bolboli L, Dehrashid A. [The Effect Paraoxonase-1, Hydrogen Peroxide and Adiponectin Changes on Systolic and Diastolic Blood Pressure of Men’s with High Blood Pressure Fallowing to 12 Week Moderate Aerobic exercise]. JSKUMS. 2016;18:81-92. Persian.
-
16.
Lakatta EG, Levy D. Arterial and cardiac aging: major shareholders in cardiovascular disease enterprises: Part II: the aging heart in health: links to heart disease. Circulation. 2003;107(2):346-54. [PubMed ID: 12538439].
-
17.
Shimizu I, Minamino T. Physiological and pathological cardiac hypertrophy. J Mol Cell Cardiol. 2016;97:245-62. [PubMed ID: 27262674]. https://doi.org/10.1016/j.yjmcc.2016.06.001.
-
18.
Shimizu I, Minamino T, Toko H, Okada S, Ikeda H, Yasuda N, et al. Excessive cardiac insulin signaling exacerbates systolic dysfunction induced by pressure overload in rodents. J Clin Invest. 2010;120(5):1506-14. [PubMed ID: 20407209]. [PubMed Central ID: PMC2860916]. https://doi.org/10.1172/JCI40096.
-
19.
Sano M, Minamino T, Toko H, Miyauchi H, Orimo M, Qin Y, et al. p53-induced inhibition of Hif-1 causes cardiac dysfunction during pressure overload. Nature. 2007;446(7134):444-8. [PubMed ID: 17334357]. https://doi.org/10.1038/nature05602.
-
20.
Paulus WJ, Tschope C. A novel paradigm for heart failure with preserved ejection fraction: comorbidities drive myocardial dysfunction and remodeling through coronary microvascular endothelial inflammation. J Am Coll Cardiol. 2013;62(4):263-71. [PubMed ID: 23684677]. https://doi.org/10.1016/j.jacc.2013.02.092.
-
21.
Baghaiee B, Nakhostin-Roohi B, Siahkuhian M, Bolboli L. [Effect of oxidative stress and exercise-induced adaptations]. J Gorgan Univ Med Sci. 2015;17(2):1-14. Persian.
-
22.
Janicki JS, Brower GL, Gardner JD, Chancey AL, Stewart JA Jr. The dynamic interaction between matrix metalloproteinase activity and adverse myocardial remodeling. Heart Fail Rev. 2004;9(1):33-42. [PubMed ID: 14739766]. https://doi.org/10.1023/B:HREV.0000011392.03037.7e.
-
23.
van Heerebeek L, Borbely A, Niessen HW, Bronzwaer JG, van der Velden J, Stienen GJ, et al. Myocardial structure and function differ in systolic and diastolic heart failure. Circulation. 2006;113(16):1966-73. [PubMed ID: 16618817]. https://doi.org/10.1161/CIRCULATIONAHA.105.587519.
-
24.
Selby DE, Palmer BM, LeWinter MM, Meyer M. Tachycardia-induced diastolic dysfunction and resting tone in myocardium from patients with a normal ejection fraction. J Am Coll Cardiol. 2011;58(2):147-54. [PubMed ID: 21718911]. [PubMed Central ID: PMC3147146]. https://doi.org/10.1016/j.jacc.2010.10.069.
-
25.
Kasner M, Westermann D, Lopez B, Gaub R, Escher F, Kuhl U, et al. Diastolic tissue Doppler indexes correlate with the degree of collagen expression and cross-linking in heart failure and normal ejection fraction. J Am Coll Cardiol. 2011;57(8):977-85. [PubMed ID: 21329845]. https://doi.org/10.1016/j.jacc.2010.10.024.
-
26.
Borbely A, van der Velden J, Papp Z, Bronzwaer JG, Edes I, Stienen GJ, et al. Cardiomyocyte stiffness in diastolic heart failure. Circulation. 2005;111(6):774-81. [PubMed ID: 15699264]. https://doi.org/10.1161/01.CIR.0000155257.33485.6D.
-
27.
Burchfield JS, Xie M, Hill JA. Pathological ventricular remodeling: mechanisms: part 1 of 2. Circulation. 2013;128(4):388-400. [PubMed ID: 23877061]. [PubMed Central ID: PMC3801217]. https://doi.org/10.1161/CIRCULATIONAHA.113.001878.
-
28.
Weeks KL, McMullen JR. The athlete's heart vs. the failing heart: can signaling explain the two distinct outcomes? Physiology (Bethesda). 2011;26(2):97-105. [PubMed ID: 21487028]. https://doi.org/10.1152/physiol.00043.2010.
-
29.
Lyon RC, Zanella F, Omens JH, Sheikh F. Mechanotransduction in cardiac hypertrophy and failure. Circ Res. 2015;116(8):1462-76. [PubMed ID: 25858069]. [PubMed Central ID: PMC4394185]. https://doi.org/10.1161/CIRCRESAHA.116.304937.
-
30.
Francis GS, McDonald KM, Cohn JN. Neurohumoral activation in preclinical heart failure. Remodeling and the potential for intervention. Circulation. 1993;87(5 Suppl):IV90-6. [PubMed ID: 8097970].
-
31.
Maillet M, van Berlo JH, Molkentin JD. Molecular basis of physiological heart growth: fundamental concepts and new players. Nat Rev Mol Cell Biol. 2013;14(1):38-48. [PubMed ID: 23258295]. [PubMed Central ID: PMC4416212]. https://doi.org/10.1038/nrm3495.
-
32.
Schiattarella GG, Hill JA. Inhibition of hypertrophy is a good therapeutic strategy in ventricular pressure overload. Circulation. 2015;131(16):1435-47. [PubMed ID: 25901069]. [PubMed Central ID: PMC4408778]. https://doi.org/10.1161/CIRCULATIONAHA.115.013894.
-
33.
Heineke J, Molkentin JD. Regulation of cardiac hypertrophy by intracellular signalling pathways. Nat Rev Mol Cell Biol. 2006;7(8):589-600. [PubMed ID: 16936699]. https://doi.org/10.1038/nrm1983.
-
34.
Bernardo BC, Weeks KL, Pretorius L, McMullen JR. Molecular distinction between physiological and pathological cardiac hypertrophy: experimental findings and therapeutic strategies. Pharmacol Ther. 2010;128(1):191-227. [PubMed ID: 20438756]. https://doi.org/10.1016/j.pharmthera.2010.04.005.
-
35.
Grossman W, Jones D, McLaurin LP. Wall stress and patterns of hypertrophy in the human left ventricle. J Clin Invest. 1975;56(1):56-64. [PubMed ID: 124746]. [PubMed Central ID: PMC436555]. https://doi.org/10.1172/JCI108079.
-
36.
Matsui T, Tao J, del Monte F, Lee KH, Li L, Picard M, et al. Akt activation preserves cardiac function and prevents injury after transient cardiac ischemia in vivo. Circulation. 2001;104(3):330-5. [PubMed ID: 11457753].
-
37.
Sawada K, Kawamura K. Architecture of myocardial cells in human cardiac ventricles with concentric and eccentric hypertrophy as demonstrated by quantitative scanning electron microscopy. Heart Vessels. 1991;6(3):129-42. [PubMed ID: 1833369].
-
38.
Yamamoto S, James TN, Sawada K, Okabe M, Kawamura K. Generation of new intercellular junctions between cardiocytes. A possible mechanism compensating for mechanical overload in the hypertrophied human adult myocardium. Circ Res. 1996;78(3):362-70. [PubMed ID: 8593694].
-
39.
Linzbach AJ. Heart failure from the point of view of quantitative anatomy. Am J Cardiol. 1960;5:370-82. [PubMed ID: 14417346].
-
40.
Linzbach AJ. Hypertrophy, hyperplasia and structural dilatation of the human heart. Adv Cardiol. 1976;18(0):1-14. [PubMed ID: 136171]. https://doi.org/10.1159/000399507.
-
41.
Kehat I, Davis J, Tiburcy M, Accornero F, Saba-El-Leil MK, Maillet M, et al. Extracellular signal-regulated kinases 1 and 2 regulate the balance between eccentric and concentric cardiac growth. Circ Res. 2011;108(2):176-83. [PubMed ID: 21127295]. [PubMed Central ID: PMC3032171]. https://doi.org/10.1161/CIRCRESAHA.110.231514.
-
42.
Cantor EJ, Babick AP, Vasanji Z, Dhalla NS, Netticadan T. A comparative serial echocardiographic analysis of cardiac structure and function in rats subjected to pressure or volume overload. J Mol Cell Cardiol. 2005;38(5):777-86. [PubMed ID: 15850571]. https://doi.org/10.1016/j.yjmcc.2005.02.012.
-
43.
Müller AL, Dhalla NS. Differences in concentric cardiac hypertrophy and eccentric hypertrophy. Cardiac Adaptations. New York: Springer; 2013. p. 147-66.
-
44.
Laughlin MH. Cardiovascular response to exercise. Adv Physiol Educ. 1999;277(6):244-59. https://doi.org/10.1152/advances.1999.277.6.S244.
-
45.
Vella CA, Robergs RA. A review of the stroke volume response to upright exercise in healthy subjects. Br J Sports Med. 2005;39(4):190-5. [PubMed ID: 15793084]. [PubMed Central ID: PMC1725174]. https://doi.org/10.1136/bjsm.2004.013037.
-
46.
Gulati M, Shaw LJ, Thisted RA, Black HR, Bairey Merz CN, Arnsdorf MF. Heart rate response to exercise stress testing in asymptomatic women: the st. James women take heart project. Circulation. 2010;122(2):130-7. [PubMed ID: 20585008]. https://doi.org/10.1161/CIRCULATIONAHA.110.939249.
-
47.
Brubaker PH, Kitzman DW. Chronotropic incompetence: causes, consequences, and management. Circulation. 2011;123(9):1010-20. [PubMed ID: 21382903]. [PubMed Central ID: PMC3065291]. https://doi.org/10.1161/CIRCULATIONAHA.110.940577.
-
48.
Higginbotham MB, Morris KG, Williams RS, Coleman RE, Cobb FR. Physiologic basis for the age-related decline in aerobic work capacity. Am J Cardiol. 1986;57(15):1374-9. [PubMed ID: 3717040].
-
49.
Lauer MS, Francis GS, Okin PM, Pashkow FJ, Snader CE, Marwick TH. Impaired chronotropic response to exercise stress testing as a predictor of mortality. JAMA. 1999;281(6):524-9. [PubMed ID: 10022108].
-
50.
Kappagoda T, Amsterdam EA. Exercise and heart failure in the elderly. Heart Fail Rev. 2012;17(4-5):635-62. [PubMed ID: 22327748]. https://doi.org/10.1007/s10741-011-9297-4.
-
51.
Roh J, Rhee J, Chaudhari V, Rosenzweig A. The Role of Exercise in Cardiac Aging: From Physiology to Molecular Mechanisms. Circ Res. 2016;118(2):279-95. [PubMed ID: 26838314]. [PubMed Central ID: PMC4914047]. https://doi.org/10.1161/CIRCRESAHA.115.305250.
-
52.
Ogawa T, Spina RJ, Martin WH 3rd, Kohrt WM, Schechtman KB, Holloszy JO, et al. Effects of aging, sex, and physical training on cardiovascular responses to exercise. Circulation. 1992;86(2):494-503. [PubMed ID: 1638717].
-
53.
Stratton JR, Levy WC, Cerqueira MD, Schwartz RS, Abrass IB. Cardiovascular responses to exercise. Effects of aging and exercise training in healthy men. Circulation. 1994;89(4):1648-55. [PubMed ID: 8149532].
-
54.
Fleg JL, Lakatta EG. Role of muscle loss in the age-associated reduction in VO2 max. J Appl Physiol (1985). 1988;65(3):1147-51. [PubMed ID: 3182484]. https://doi.org/10.1152/jappl.1988.65.3.1147.
-
55.
Haykowsky MJ, Brubaker PH, Stewart KP, Morgan TM, Eggebeen J, Kitzman DW. Effect of endurance training on the determinants of peak exercise oxygen consumption in elderly patients with stable compensated heart failure and preserved ejection fraction. J Am Coll Cardiol. 2012;60(2):120-8. [PubMed ID: 22766338]. [PubMed Central ID: PMC3429944]. https://doi.org/10.1016/j.jacc.2012.02.055.
-
56.
Borlaug BA, Olson TP, Lam CS, Flood KS, Lerman A, Johnson BD, et al. Global cardiovascular reserve dysfunction in heart failure with preserved ejection fraction. J Am Coll Cardiol. 2010;56(11):845-54. [PubMed ID: 20813282]. [PubMed Central ID: PMC2950645]. https://doi.org/10.1016/j.jacc.2010.03.077.
-
57.
Fleg JL, O'Connor F, Gerstenblith G, Becker LC, Clulow J, Schulman SP, et al. Impact of age on the cardiovascular response to dynamic upright exercise in healthy men and women. J Appl Physiol (1985). 1995;78(3):890-900. [PubMed ID: 7775334]. https://doi.org/10.1152/jappl.1995.78.3.890.
-
58.
Pluim BM, Lamb HJ, Kayser HW, Leujes F, Beyerbacht HP, Zwinderman AH, et al. Functional and metabolic evaluation of the athlete's heart by magnetic resonance imaging and dobutamine stress magnetic resonance spectroscopy. Circulation. 1998;97(7):666-72. [PubMed ID: 9495302].
-
59.
Baggish AL, Wang F, Weiner RB, Elinoff JM, Tournoux F, Boland A, et al. Training-specific changes in cardiac structure and function: a prospective and longitudinal assessment of competitive athletes. J Appl Physiol (1985). 2008;104(4):1121-8. [PubMed ID: 18096751]. https://doi.org/10.1152/japplphysiol.01170.2007.
-
60.
Colan SD. Mechanics of left ventricular systolic and diastolic function in physiologic hypertrophy of the athlete's heart. Cardiol Clin. 1997;15(3):355-72. [PubMed ID: 9276162].
-
61.
Vinereanu D, Florescu N, Sculthorpe N, Tweddel AC, Stephens MR, Fraser AG. Left ventricular long-axis diastolic function is augmented in the hearts of endurance-trained compared with strength-trained athletes. Clin Sci (Lond). 2002;103(3):249-57. [PubMed ID: 12193150]. https://doi.org/10.1042/cs1030249.
-
62.
Lalande S, Baldi JC. Left ventricular mass in elite olympic weight lifters. Am J Cardiol. 2007;100(7):1177-80. [PubMed ID: 17884384]. https://doi.org/10.1016/j.amjcard.2007.05.036.
-
63.
MacDougall JD, Tuxen D, Sale DG, Moroz JR, Sutton JR. Arterial blood pressure response to heavy resistance exercise. J Appl Physiol (1985). 1985;58(3):785-90. [PubMed ID: 3980383]. https://doi.org/10.1152/jappl.1985.58.3.785.
-
64.
Scharf M, Brem MH, Wilhelm M, Schoepf UJ, Uder M, Lell MM. Atrial and ventricular functional and structural adaptations of the heart in elite triathletes assessed with cardiac MR imaging. Radiology. 2010;257(1):71-9. [PubMed ID: 20807850]. https://doi.org/10.1148/radiol.10092377.
-
65.
Fagard R. Athlete's heart. Heart. 2003;89(12):1455-61. [PubMed ID: 14617564]. [PubMed Central ID: PMC1767992].
-
66.
Mihl C, Dassen WR, Kuipers H. Cardiac remodelling: concentric versus eccentric hypertrophy in strength and endurance athletes. Neth Heart J. 2008;16(4):129-33. [PubMed ID: 18427637]. [PubMed Central ID: PMC2300466].
-
67.
Serra AJ, Higuchi ML, Ihara SS, Antonio EL, Santos MH, Bombig MT, et al. Exercise training prevents beta-adrenergic hyperactivity-induced myocardial hypertrophy and lesions. Eur J Heart Fail. 2008;10(6):534-9. [PubMed ID: 18502686]. https://doi.org/10.1016/j.ejheart.2008.03.016.
-
68.
Serra AJ, Santos MH, Bocalini DS, Antonio EL, Levy RF, Santos AA, et al. Exercise training inhibits inflammatory cytokines and more than prevents myocardial dysfunction in rats with sustained beta-adrenergic hyperactivity. J Physiol. 2010;588(Pt 13):2431-42. [PubMed ID: 20442263]. [PubMed Central ID: PMC2915518]. https://doi.org/10.1113/jphysiol.2010.187310.
-
69.
Fernandes T, Soci UP, Oliveira EM. Eccentric and concentric cardiac hypertrophy induced by exercise training: microRNAs and molecular determinants. Braz J Med Biol Res. 2011;44(9):836-47. [PubMed ID: 21881810].
-
70.
Wang Y, Wisloff U, Kemi OJ. Animal models in the study of exercise-induced cardiac hypertrophy. Physiol Res. 2010;59(5):633-44. [PubMed ID: 20406038].
-
71.
Wright KJ, Thomas MM, Betik AC, Belke D, Hepple RT. Exercise training initiated in late middle age attenuates cardiac fibrosis and advanced glycation end-product accumulation in senescent rats. Exp Gerontol. 2014;50:9-18. [PubMed ID: 24280067]. https://doi.org/10.1016/j.exger.2013.11.006.
-
72.
Choi SY, Chang HJ, Choi SI, Kim KI, Cho YS, Youn TJ, et al. Long-term exercise training attenuates age-related diastolic dysfunction: association of myocardial collagen cross-linking. J Korean Med Sci. 2009;24(1):32-9. [PubMed ID: 19270810]. [PubMed Central ID: PMC2650965]. https://doi.org/10.3346/jkms.2009.24.1.32.
-
73.
Wang W, Zhang H, Xue G, Zhang L, Zhang W, Wang L, et al. Exercise training preserves ischemic preconditioning in aged rat hearts by restoring the myocardial polyamine pool. Oxid Med Cell Longev. 2014;2014:457429. [PubMed ID: 25404991]. [PubMed Central ID: PMC4227379]. https://doi.org/10.1155/2014/457429.
-
74.
Kwak HB, Song W, Lawler JM. Exercise training attenuates age-induced elevation in Bax/Bcl-2 ratio, apoptosis, and remodeling in the rat heart. FASEB J. 2006;20(6):791-3. [PubMed ID: 16459353]. https://doi.org/10.1096/fj.05-5116fje.
-
75.
Rossoni LV, Oliveira RA, Caffaro RR, Miana M, Sanz-Rosa D, Koike MK, et al. Cardiac benefits of exercise training in aging spontaneously hypertensive rats. J Hypertens. 2011;29(12):2349-58. [PubMed ID: 22045123]. https://doi.org/10.1097/HJH.0b013e32834d2532.
-
76.
Huang CY, Yang AL, Lin YM, Wu FN, Lin JA, Chan YS, et al. Anti-apoptotic and pro-survival effects of exercise training on hypertensive hearts. J Appl Physiol (1985). 2012;112(5):883-91. [PubMed ID: 22207725]. https://doi.org/10.1152/japplphysiol.00605.2011.
-
77.
Iemitsu M, Maeda S, Jesmin S, Otsuki T, Miyauchi T. Exercise training improves aging-induced downregulation of VEGF angiogenic signaling cascade in hearts. Am J Physiol Heart Circ Physiol. 2006;291(3):H1290-8. [PubMed ID: 16617130]. https://doi.org/10.1152/ajpheart.00820.2005.
-
78.
Lai CH, Ho TJ, Kuo WW, Day CH, Pai PY, Chung LC, et al. Exercise training enhanced SIRT1 longevity signaling replaces the IGF1 survival pathway to attenuate aging-induced rat heart apoptosis. Age (Dordr). 2014;36(5):9706. [PubMed ID: 25148910]. [PubMed Central ID: PMC4453937]. https://doi.org/10.1007/s11357-014-9706-4.
-
79.
Yamashita K, Kajstura J, Discher DJ, Wasserlauf BJ, Bishopric NH, Anversa P, et al. Reperfusion-activated Akt kinase prevents apoptosis in transgenic mouse hearts overexpressing insulin-like growth factor-1. Circ Res. 2001;88(6):609-14. [PubMed ID: 11282895].
-
80.
Liao PH, Hsieh DJ, Kuo CH, Day CH, Shen CY, Lai CH, et al. Moderate exercise training attenuates aging-induced cardiac inflammation, hypertrophy and fibrosis injuries of rat hearts. Oncotarget. 2015;6(34):35383-94. [PubMed ID: 26496028]. [PubMed Central ID: PMC4742112]. https://doi.org/10.18632/oncotarget.6168.
-
81.
DeBosch B, Treskov I, Lupu TS, Weinheimer C, Kovacs A, Courtois M, et al. Akt1 is required for physiological cardiac growth. Circulation. 2006;113(17):2097-104. [PubMed ID: 16636172]. https://doi.org/10.1161/CIRCULATIONAHA.105.595231.
-
82.
Jiang Y, Chen C, Li Z, Guo W, Gegner JA, Lin S, et al. Characterization of the structure and function of a new mitogen-activated protein kinase (p38beta). J Biol Chem. 1996;271(30):17920-6. [PubMed ID: 8663524].
-
83.
Gioscia-Ryan RA, Battson ML, Cuevas LM, Zigler MC, Sindler AL, Seals DR. Voluntary aerobic exercise increases arterial resilience and mitochondrial health with aging in mice. Aging (Albany NY). 2016;8(11):2897-914. [PubMed ID: 27875805]. [PubMed Central ID: PMC5191877]. https://doi.org/10.18632/aging.101099.