Abstract
Background:
Nowadays, finding natural compounds with antimicrobial properties against pathogens is very important, especially for the food and drug industries.Objectives:
The antibacterial activity of chitosan coatings nanoemulsion (NE) containing Zataria multiflora and Bunium persicum essential oils (EOs) was evaluated in a food model (chicken breast fillets) during 15 days of refrigerated storage.Methods:
The chicken breast fillets were divided into seven groups: control, chitosan 2%, sonicated chitosan 2%, chitosan NE coating containing Z. muitiflora EO (ZMEO, 0.5%, and 1 %) and chitosan NE coating containing B. persicum EO (BPEO, 0.5%, and 1 %). Characteristics of chitosan NE coatings containing EOs were analyzed. Moreover, the antimicrobial activity of coatings against Listeria monocytogenes was investigated.Results:
The results showed good properties of the NE coatings. The analysis of EOs revealed that the major components for ZMEO were carvacrol (51.55%) and thymol (25.49%). In addition, the main components of BPEO were p-cumic aldehyde (38.39%) and p-cymene (18.36%). All treatments exhibited antimicrobial properties; however, the best result was recorded for chitosan NE coating containing 1% ZMEO, which was the lowest amount of L. monocytogenes (7.61 Log CFU/g). Moreover, L. monocytogenes analysis for chitosan NE coating containing 1% BPEO samples was 7.73 Log CFU/g.Conclusions:
Therefore, based on the results of this study, chitosan NE coating containing ZMEO and BPEO as natural preservatives can be recommended for meat products, especially chicken meats.Keywords
Listeria monocytogenes Bunium persicum Essential Oil Zataria multiflora Essential Oil Chitosan Edible Coating Nanoemulsion Chicken Breast Fillet
1. Background
Chicken meat is very susceptible to the growth of spoilage, pathogenic microorganisms, and oxidative reactions and has a very short shelf-life. The consumption of chicken meat has increased during last several years because of low-fat content, high nutritional value, distinctive flavor, and comparatively low cost of production compared to other meat products. High protein content, high moisture level, and relatively high pH value lead to microbial spoilage of meat, whereas aerobic condition causes the oxidation of lipid and protein (1, 2). There has been an increasing demand for healthy and safe foods during the last decades since several serious diseases are caused by food-borne pathogenic microorganisms (3). Each year, 48 million individuals are estimated to get sick from food-borne diseases in the United States (4). The presence of microorganisms in foodstuffs has a critical significance, as they can decompose and deteriorate food quality and some health problems. Thermal processing not only could be effective in inactivating pathogenic bacteria in food, but on the other hand, it could lead to the deterioration of nutritional and organoleptic properties of the food (3, 5).
Among the various types of known food-borne diseases, Salmonella and Listeria are known as major pathogenic bacteria in raw meat (beef and chicken), eggs (4), raw vegetables (6), dairy products (7), and ready-to-eat foods (5).
Many consumers have become aware of chemical preservatives' adverse effects to retard microbial growth in meat products (8). This knowledge formed a trend toward green consumerism and the replacement of chemical preservatives with natural antimicrobial agents (1). Therefore, many efforts have been carried out to investigate edible and natural compounds with antimicrobial properties to increase the shelf life and quality of various foods.
Many approaches have been proposed to improve the shelf life of food (9); one of them is the application of edible coatings (10). Chitosan (a cationic polysaccharide) is one of the edible coatings applied in the food industry owing to its bioactive features, including antifungal, antibacterial, and antioxidative activities. It is a natural biopolymer obtained from crab and shrimp shells and has been classified as generally recognized as safe (GRAS) (11-13). Chitosan coatings exhibited antibacterial activity against gram-negative and gram-positive bacteria (14). Their antimicrobial activity can be improved via some approaches. For instance, chitosan coatings can be incorporated with essential oils (EOs). Essential oils such as Bunium persicum and Zataria muitiflora are originated from herbal plants and well known as natural antimicrobial agents (15).
Nanoemulsions (NEs) are colloidal systems with an oil phase dispersed in an aqueous phase (16). Incorporating NEs with EOs leads to an increase in the contact surface of EOs. It reduces their necessary dose, which is beneficial since it reduces their undesirable effects on the organoleptic properties of foods (17, 18). Nanoemulsions containing EOs or their components, proved to be effective against a wide range of microorganisms (19, 20).
Chicken meat is a staple food in the human diet, but it is an enriched media for the growth of pathogenic microorganisms and spoilage bacteria. Many pathogenic bacteria can be found in chicken meats; among them, Listeria is of the major pathogenic bacteria in raw meat (beef and chicken), eggs (4), raw vegetables (6), dairy products (7), and ready-to-eat foods (5).
Few studies have used active chitosan coatings, especially NE forms to control pathogenic bacteria. Shukla et al. (21) applied chitosan coating combined with Syzygium aromaticum in chicken patties and improved the shelf life of samples. In another study, chitosan and pomegranate were used to improve the quality of chicken samples at 4°C (1). However, to our knowledge, no study has investigated the antibacterial effect of chitosan coating NE containing Zataria multiflora essential oil (ZMEO) and Bunium persicum essential oil (BPEO) against pathogenic bacteria on chicken meat.
2. Objectives
The aim of the present study was to (1) prepare and evaluate the characteristic of chitosan coating NEs embedded with ZMEO and PBEO; (2) assess the antibacterial properties of NE against Listeria monocytogenes in chicken breast fillets during 15 days of storage at 4°C.
3. Methods
3.1. Materials
Extraction and analysis of Z. multiflora and B. persicum were carried out at the Iranian Institute of Medicinal Plants, Karaj, Alborz province, Iran. The EOs were kept at 4°C in the refrigerator away from direct light until the analysis time. All of the culture media were obtained from Merck (Darmstadt, Germany). Listeria monocytogenes (ATCC: 7644, 7834, 10671, 82119) strains were prepared from the Department of Food Hygiene, Faculty of Veterinary Medicine, Ferdowsi University of Mashhad, Mashhad, Iran.
3.2. Chitosan Coating Nanoemulsion Containing Zataria multiflora Essential Oil and Bunium persicum Essential Oil Preparation
Chitosan coating NE containing ZMEO and PBEO with some modifications was formulated according to Severino et al. (22). The non-ionic surfactant of Tween 80 (HLB = 15) and sterile distilled water were used to prepare the coating. Distilled water was used to dissolve chitosan (2% w/v); subsequently, acetic acid glacial (1% w/v) was added to the mixture. Then, a plasticizer (0.75 mL/g glycerol) was added to the mixture, and they were stirred for 10 min to obtain a transparent mixture.
After preparation of the chitosan solution, ZMEO and PBEO were dissolved in two concentrations (0.5% and 1%) with an emulsifier (Tween 80, 0.2% w/v) and stirred by using a hotplate magnetic stirrer (IKA, C-MAG HS 10, Germany) to acquire a uniform, stable, and transparent homogenous mixture. Then, it was transferred to a beaker containing chitosan solution. Chitosan solution containing EOs were homogenized using an Ultra Turrax T25 (IKA Labortechnik, Germany) high shear mixer at 3,000 rpm for 3 min and ultrasound probe sonicator device (50°C, pulse; 45 s and rest; 15 s) for 6 minutes, to form NE. The investigated treatments were chitosan 2%, sonicated chitosan 2%, chitosan coating NE containing ZMEO at two levels (0.5 and 1%) and chitosan coating NE containing BPEO at two levels (0.5 and 1%).
3.3. Droplet Size, Size Distribution, and ζ-potential and Preparation of Listeria monocytogenes
ζ-potential test shows the stability of emulsions. The analysis was carried out according to Noori et al. (11). The mean particle size (Z-average) was evaluated using the dynamic light scattering (DLS) at 25°C on a Zetasizer Nano S90, (Malvern Instruments, Malvern, Worcestershire, UK). The dilution was performed with distilled water (100: 1) before the measurement. All of the measurements were recorded at 25°C.
To prepare suspensions of L. monocytogenes (four strains), bacteria were cultured in 10 mL of BHI, and incubation was carried out at 37°C for 24 hours. Then, they were incubated for 18 hours at 37°C in supplementary culture media (BHI), and bacterial suspensions were prepared. Subsequently, bacterial suspensions were diluted to obtain 0.5 MacFarland turbidity (108 × 1.5 CFU/mL).
3.4. Chicken Fillets Preparation, Inoculation of the Bacteria, and Listeria monocytogenes Analysis
The chicken breasts were obtained from a poultry vendor in Mashhad, Iran during the summer of 2018. They were filleted and transported to the laboratory. Then, they were washed with cold tap water. From chicken breasts 10 ± 1 g fillets were prepared. Then the samples were immersed in ethanol solution (70% v/v) and burnt to remove the surface microorganisms. In the end, 50 μL of 1.5 ×108 CFU/mL dilution of L. monocytogenes strains were inoculated to achieve a final concentration of ~ 106 CFU/g. After inoculation of the bacteria on each sample, they were kept at room temperature for 30 to 45 minutes, to attach the bacteria into the surface of the samples. Then, the fillet samples were randomly divided into seven groups (as presented in Table 1), and were dipped in different coating treatments for 1 min and then drained for 30 min. Finally, the treated samples were placed at 4 ± 1°C for 15 days and tested every three days (day 0, 3, 6, 9, 12, and 15).
List of the Treatments in the Present Study
No. | Treatment | Description |
---|---|---|
1 | CON | Samples without any coating solution (control) |
2 | CH | Samples coated with chitosan solution |
3 | S + CH | Samples coated with sonicated-chitosan solution |
4 | NANO + CH + ZMEO 0.5% | Samples coated with chitosan solution containing Zataria multiflora essential oil (EO) 0.5% (w/v) |
5 | NANO + CH + ZMEO 1% | Samples coated with chitosan solution nanoemulsion (NE) containing Z. multiflora EO 1% (w/v) |
6 | NANO + CH + BPEO 0.5% | Samples coated with chitosan solution NE containing Bunium persicum EO 0.5% (w/v) |
7 | NANO + CH + BPEO 1% | Samples coated with chitosan solution NE containing B. persicum EO 1% (w/v) |
Ninety mL sterile peptone water (0.1%) and 10 g of fillets were added in a plastic bag and homogenized by a stomacher (Seward Medical, London, UK) for three minutes. Decimal dilutions of homogenized samples were made, and 10 μL of each dilution were transferred on Palcam agar and Xylose-lysin-decarboxycholate-agar (XLD) to count L. monocytogenes. The incubation was done for 24 - 48 h at 37°C.
3.5. Statistical Analysis
The results were presented as means ± standard deviations (SD). The results were analyzed through one-way analysis of variance (ANOVA). All analysis was carried out in triplicate, and the statistical analysis was done using SPSS version 21 software. Analysis of variance and Bonferroni post hoc test or Dunnette T3 post-test was used to determine the significant difference at the level of P < 0.05. Also, to evaluate the stability of the treatments Kruskal-Wallis, Friedman, and Wilcoxon signed-rank tests were carried out.
4. Results and Discussion
4.1. Zataria multiflora and Bunium persicum Essential Oils Composition
The analysis of ZMEO and BMEO were performed by GC-MS, which showed the presence of 13 and 17 components, respectively (Table 2). Also, 91.44% and 94.54% of chemical compounds were identified in ZMEO and BMEO, respectively. The main components of ZMEO were thymol (25.49%), carvacrol (51.55%), alpha-terpinen (4.44%), and p-cymene (5.23%), while, in the case of BPEO p-cymene (18.36%), gamma terpinene (7.38%), cumene (4.69%), p-cumic aldehyde (38.39%), and 2-caren-10-al (13.26%) were the major constituents. The data obtained from this study was in accordance with another study from Iran (23). However, in another study, the main components of the BPEO were α-methyl-benzenemethanol (14.59 %), p-cuminaldehyde (23.50 %), γ-terpinene (13.10 %), and β-cymene (8.48 %) that were different from the present results (24). These differences may be attributed to factors like plant growth phase, geographic location, and genetic and environmental factors (25).
Chemical Composition of Zataria multiflora Essential Oil and Bunium persicum Essential Oil Analyzed by GC/MS
Zataria multiflora Essential Oil | Bunium persicum Essential Oil | ||||
---|---|---|---|---|---|
Compounds | RT | Relative Percent of Compounds | Compounds | RT | Relative Percent of Compounds |
1R-.alpha.-Pinene | 11.04 | 1.68 | Cumene | 11.937 | 4.69 |
-.alpha.-Phellandrene | 13.66 | 0.1 | Beta- Pinene | 14.355 | 3.89 |
(+)-4-Carane | 14.11 | 1.12 | (+)-Camphene | 14.975 | 0.50 |
p-Cymene | 14.43 | 5.23 | Alpha-Terpinen | 15.478 | 0.36 |
a-Terpinen | 15.71 | 4.44 | P-Cymene | 16.059 | 18.36 |
Bornel | 19.96 | 0.11 | Gama-Terpinen | 17.222 | 7.38 |
Terpinen-4-ol | 20.37 | 0.39 | R-(+)-Pulegone | 22.739 | 2.73 |
Alpha Terpineol | 20.98 | 0.21 | Alpha-Terpieol | 23.560 | 0.34 |
Bornyl acetate | 24.50 | 0.20 | 3-Caren-10-al | 24.033 | 0.14 |
Thymol | 25.60 | 25.49 | P-Cumic aldehyde | 25.737 | 38.39 |
Carvacrol | 26.07 | 51.55 | Alpha-Thujenal | 26.171 | 1.27 |
Valencen | 31.91 | 0.60 | Phellandral | 26.964 | 0.35 |
Caryophyllene oxide | 39.23 | 0.32 | Isogeraniol | 27.070 | 0.59 |
- | - | 91.44% | 2-Caren-10-al | 27.442 | 13.26 |
P-Cymen-7-ol | 28.984 | 1.33 | |||
Carvacrol | 30.557 | 0.91 | |||
Caryophyllen | 35.307 | 0.05 | |||
- | - | 94.54% |
4.2. Particle Size of Nanoemulsions
Table 3 shows the particle size for NEs as well as the polydispersity index. Based on the results, NEs were stable and showed a low particle diameter and uniform distribution. As represented in Table 2, the highest particle size of the chitosan coating combined with essential oils was about 1363 ± 5 nm, and when converted to sonicated chitosan, the mean particle size was reduced to 532 ± 2 nm. BPEO 0.5% and 1% particle size were 159 ± 1 nm and 188 ± 1 nm, respectively, indicating the lowest particle size. The lowest particle size was associated with chitosan containing BPEO 0.5% with a mean particle diameter of 159 nm, similar to the results of the study done by Artiga-Artigas et al. (26). In our study, the particle size was measured by DLS method, and the particle size was less than 600 nm in the range, which corresponded with the data obtained by the study of Jafari et al. (27), Hatanaka et al. (28) and Salvia-Trujillo et al. (29). In another study performed by Sow et al. (30), the average size of particles was 309 ± 19 nm (mean ± SD), which was in agreement with the present study, because the particle size was below 600 nm.
Treatment | Particle Size | ζ-potential | Particle Size Distribution | P Value |
---|---|---|---|---|
CH | 1363 ± 5 nm A | 53.566 ± 51 mV A | 0.47 ± 0.015 A | < 0.05 |
S + CH | 532 ± 2 nm B | 43.13 ± 32 mV B | 0.28 ± 0.015 B | |
Nano + CH + ZMEO %0.5 | 243 ± 4 nm C | 44.46 ± 3.5 mV C | 0.30 ± 0.04 C | |
Nano + CH + ZMEO %1 | 277 ± 2 nm D | 37.46 ±2.8 mV D | 0.49 ± 0.02 D | |
Nano + CH + BPEO %0.5 | 159 ± 1 nm E | 47.33 ± 2.5 mV E | 0.29 ± 0.02 E | |
Nano + CH + BPEO %1 | 188 ± 1 nm F | 35.86 ± 3.3 mV F | 0.41 ± 0.02 F |
4.3. Antimicrobial Effects of Chitosan Coating Nanoemulsion in Combination with Zataria multiflora and Bunium persicum Essential Oils
The effects of chitosan coating NE containing Z. multiflora and B. persicum EOs and its effects against L. monocytogenes inoculated on chicken breast fillets were investigated during 15 days of storage (4°C). The results are summarized in Tables 3 and 4.
Reduction of Listeria monocytogenes Counts in Treatment Groups During 15 Days Storage at 4°C a
Mean Difference I - J | Group(J) | Chitosan | Nanochitosan | Nano-chitosan + BPEO 0.5% | Nano-chitosan + BPEO 1% | Nano-chitosan + ZMEO 0.5% | Nano-chitosan + ZMEO 1% |
---|---|---|---|---|---|---|---|
Group(I) | |||||||
Control | 0.14* | 0.35* | 0.61* | 1.03* | 1.00* | 1.25* | |
Chitosan | 0.20* | 0.47* | 0.88* | 0.86* | 1.10* | ||
Nanochitosan | 0.26* | 0.67* | 0.65* | 0.90* | |||
Nano-chitosan + BPEO 0.5% | 0.41* | 0.02* | 0.22* | ||||
Nano-chitosan + BPEO 1% | 0.02 | 0.22* | |||||
Nano-chitosan + ZMEO 0.5% | 0.24* |
Changes in bacterial counts during 15 days of storage were represented in Figure 1. Chitosan coating nanoemulsion containing ZMEO 1% showed the highest antimicrobial activity against L. monocytogenes, while simple chitosan coating represented low antimicrobial activity against the tested bacterium. The data indicated that after 15 days of storage, the control group showed an increase in L. monocytogenes (from 6.20 Log CFU/g on day 1 to 9.20 Log CFU/g on day 15). Treatment groups exhibited a microbial load of 6.07 Log CFU/g up to 9 Log CFU/g, indicating a slight decrease compared to the control group. This agreed with the result of Hassanzadeh et al. (12). The antimicrobial activity of chitosan coatings can be found in many researches, reporting similar results (14, 31, 32). Antimicrobial properties of chitosan coatings may be associated with the alteration in the penetrability of the cell membrane, the interactions between the chitosan amine groups, and the electronegative load at the cell surface, which causes leakage of intracellular electrolytes and proteinaceous components (3, 33).
Changes in bacterial count (CFU/g) of chicken breast fillet samples inoculated with L. monocytogenes during the storage period (mean ± SD)
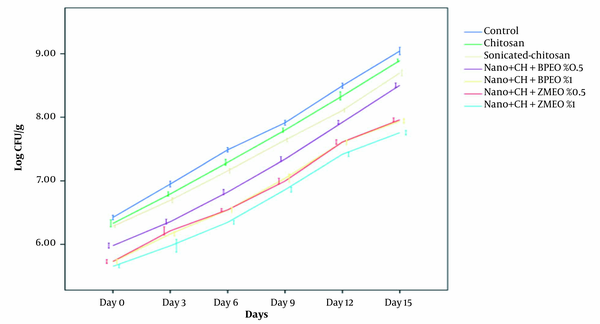
Sonicated-chitosan coating showed an increase of L. monocytogenes count from 5.89 Log CFU/g up to 8.76 Log CFU/g after 15 days of storage in the refrigerator, which showed a significant reduction in bacterial count in comparison with the chitosan and the control group. The reason is that in this form, the surface/volume ratio increases, and chitosan coating can easily penetrate to the bacterial membrane.
In the chitosan coating NE containing BPEO 0.5% and 1%, L. monocytogenes population decreased significantly from an initial value of 5.78 - 8.27 Log CFU/g to 5.41 Log CFU/g - 7.73 Log CFU/g after 15 days storage at the refrigerator compared to other treatments (nanochitosan, chitosan, and control groups). Antimicrobial activity of BPEO 1% was higher than BPEO 0.5% due to its higher concentration of EO. As mentioned above, chitosan coating alone has little antimicrobial activity. Noori et al. reported that adding EO to the NE system caused a significant increase in the antimicrobial activity (P < 0.05) (11). Antimicrobial activity of BEO is due to the presence of p-cymene, gamma terpinene, cumene, p-cumic aldehyde, and other active constituents, which are often terpenic compounds (24, 34). Topuz et al. reported that nanoemulsion of anise oil reduced Escherihia coli O157: H7 and L. monocytogenes population more than coarse emulsions (15). This could be due to low particle size and higher exposed area to the microbial cells (15).
In the NE chitosan coating containing ZMEO, 0.5% and 1% L. monocytogenes population increased significantly from 5.41 Log CFU/g - 7.83 Log CFU/g to 5.19 Log CFU/g - 7.61 Log CFU/g during 15 days of refrigerated storage. Although bacterial number has been increased during storage, ZMEO treatments (0.5% and 1%) significantly reduced bacterial count (1 and 1.25 Log CFU/g, respectively) compared to the control group. Among these treatments, ZMEO 1% had the highest antimicrobial activity against both bacteria due to the higher concentration of EO and thymol and carvacrol as the main antimicrobial compounds (34-36). These results agreed with those reported by Abdollahzadeh et al., indicating that the combination of thyme EO 0.4% and nisin with 1000 IU/g concentration were efficient means of L. monocytogenesin controlling in minced fish (36). Moreover, in another study, Z. multiflora which contained 36.62% carvacrol, was used in fresh chicken fillets and significantly improved the shelf life of samples (37).
Based on our findings (Figure 1), the antimicrobial activity of NE chitosan coating containing EOs was excellent against L. monocytogenes. All treatments exhibited an increasing trend during 15 days of storage at 4°C. However, the best result was observed in chitosan nanoemulsion combined with 1% ZMEO followed by chitosan nanoemulsion combined with 1% BPEO.
4.4. Conclusions
In the current study, preparation, characteristic properties and application of nanochitosan-based edible coating containing ZMEO and BPEO were performed. The best results of nanoemulsion stability tests were related to chitosan coating containing nanoemulsion of PBEO 0.5%. The use of ZMEO and BPEO antimicrobial agents in nanochitosan coating can effectively maintain their antibacterial activity in chicken breast fillets during storage and can significantly reduce the growth of these two pathogens. Our results also showed that nanochitosan containing ZMEO 1% had the best antimicrobial effect on the growth of L. monocytogenes in chicken breast fillets. Finally, it should be considered that the introduced treatments cannot guarantee the safety of cold chicken breasts when contaminated with high doses (106 CFU/g) of L. monocytogenes due to their ability to grow at cold temperatures. However, the samples treated with essential oils can reduce the microbial count for about 1.25 Log CFU/g after 15 days of storage at 4°C. Therefore, due to the interest of consumers and manufacturers in using natural additives instead of chemical additives in food, nanochitosan containing EOs can be used efficiently in chicken breast fillets to enhance its safety against the two pathogenic bacteria.
Acknowledgements
References
-
1.
Bazargani-Gilani B, Aliakbarlu J, Tajik H. Effect of pomegranate juice dipping and chitosan coating enriched with Zataria multiflora Boiss essential oil on the shelf-life of chicken meat during refrigerated storage. Innov Food Sci Emerg Technol. 2015;29:280-7. https://doi.org/10.1016/j.ifset.2015.04.007.
-
2.
Konuk Takma D, Korel F. Active packaging films as a carrier of black cumin essential oil: Development and effect on quality and shelf-life of chicken breast meat. Food Packag Shelf Life. 2019;19:210-7. https://doi.org/10.1016/j.fpsl.2018.11.002.
-
3.
Severino R, Ferrari G, Vu K, Donsì F, Salmieri S, Lacroix M. Antimicrobial effects of modified chitosan based coating containing nanoemulsion of essential oils, modified atmosphere packaging and gamma irradiation against Escherichia coli O157:H7 and Salmonella Typhimurium on green beans. Food Control. 2015;50:215-22. https://doi.org/10.1016/j.foodcont.2014.08.029.
-
4.
Jones DR, Anderson KE, Guard JY. Prevalence of coliforms, Salmonella, Listeria, and Campylobacter associated with eggs and the environment of conventional cage and free-range egg production. Poult Sci. 2012;91(5):1195-202. [PubMed ID: 22499879]. https://doi.org/10.3382/ps.2011-01795.
-
5.
Rajaei A, Hadian M, Mohsenifar A, Rahmani-Cherati T, Tabatabaei M. A coating based on clove essential oils encapsulated by chitosan-myristic acid nanogel efficiently enhanced the shelf-life of beef cutlets. Food Packag Shelf Life. 2017;14:137-45. https://doi.org/10.1016/j.fpsl.2017.10.005.
-
6.
Sant'Ana AS, Barbosa MS, Destro MT, Landgraf M, Franco BD. Growth potential of Salmonella spp. and Listeria monocytogenes in nine types of ready-to-eat vegetables stored at variable temperature conditions during shelf-life. Int J Food Microbiol. 2012;157(1):52-8. [PubMed ID: 22561064]. https://doi.org/10.1016/j.ijfoodmicro.2012.04.011.
-
7.
Modi R, Hirvi Y, Hill A, Griffiths MW. Effect of phage on survival of Salmonella enteritidis during manufacture and storage of cheddar cheese made from raw and pasteurized milk. J Food Prot. 2001;64(7):927-33. [PubMed ID: 11456198]. https://doi.org/10.4315/0362-028x-64.7.927.
-
8.
Moghimi R, Ghaderi L, Rafati H, Aliahmadi A, McClements DJ. Superior antibacterial activity of nanoemulsion of Thymus daenensis essential oil against E. coli. Food Chem. 2016;194:410-5. [PubMed ID: 26471573]. https://doi.org/10.1016/j.foodchem.2015.07.139.
-
9.
Zehi ZB, Afshari A, Noori SMA, Jannat B, Hashemi M. The Effects of X-Ray Irradiation on Safety and Nutritional Value of Food: A Systematic Review Article. Curr Pharm Biotechnol. 2020;21(10):919-26. [PubMed ID: 32072900]. https://doi.org/10.2174/1389201021666200219093834.
-
10.
Guilbert S, Gontard N, Cuq B. Technology and applications of edible protective films. Packag Technol Sci. 1995;8(6):339-46. https://doi.org/10.1002/pts.2770080607.
-
11.
Noori S, Zeynali F, Almasi H. Antimicrobial and antioxidant efficiency of nanoemulsion-based edible coating containing ginger (Zingiber officinale) essential oil and its effect on safety and quality attributes of chicken breast fillets. Food Control. 2018;84:312-20. https://doi.org/10.1016/j.foodcont.2017.08.015.
-
12.
Hassanzadeh P, Tajik H, Rohani SMR, Moradi M, Hashemi M, Aliakbarlu J. Effect of functional chitosan coating and gamma irradiation on the shelf-life of chicken meat during refrigerated storage. Radiat Phys Chem. 2017;141:103-9. https://doi.org/10.1016/j.radphyschem.2017.06.014.
-
13.
Khanjari A, Karabagias IK, Kontominas MG. Combined effect of N,O-carboxymethyl chitosan and oregano essential oil to extend shelf life and control Listeria monocytogenes in raw chicken meat fillets. LWT - Food Sci Technol. 2013;53(1):94-9. https://doi.org/10.1016/j.lwt.2013.02.012.
-
14.
Vardaka VD, Yehia HM, Savvaidis IN. Effects of Citrox and chitosan on the survival of Escherichia coli O157:H7 and Salmonella enterica in vacuum-packaged turkey meat. Food Microbiol. 2016;58:128-34. [PubMed ID: 27217368]. https://doi.org/10.1016/j.fm.2016.04.003.
-
15.
Topuz OK, Ozvural EB, Zhao Q, Huang Q, Chikindas M, Golukcu M. Physical and antimicrobial properties of anise oil loaded nanoemulsions on the survival of foodborne pathogens. Food Chem. 2016;203:117-23. [PubMed ID: 26948596]. https://doi.org/10.1016/j.foodchem.2016.02.051.
-
16.
Zambrano-Zaragoza ML, Gonzalez-Reza R, Mendoza-Munoz N, Miranda-Linares V, Bernal-Couoh TF, Mendoza-Elvira S, et al. Nanosystems in Edible Coatings: A Novel Strategy for Food Preservation. Int J Mol Sci. 2018;19(3). [PubMed ID: 29494548]. [PubMed Central ID: PMC5877566]. https://doi.org/10.3390/ijms19030705.
-
17.
Guerra-Rosas MI, Morales-Castro J, Ochoa-Martínez LA, Salvia-Trujillo L, Martín-Belloso O. Long-term stability of food-grade nanoemulsions from high methoxyl pectin containing essential oils. Food Hydrocoll. 2016;52:438-46. https://doi.org/10.1016/j.foodhyd.2015.07.017.
-
18.
Hashemi M, Hashemi M, Daneshamooz S, Raeisi M, Jannat B, Taheri S, et al. An Overview on Antioxidants Activity of Polysaccharide Edible Films and Coatings Contains Essential Oils and Herb Extracts in Meat and Meat Products. Adv Anim Vet Sci. 2020;8(2). https://doi.org/10.17582/journal.aavs/2020/8.2.198.207.
-
19.
Zhang Z, Vriesekoop F, Yuan Q, Liang H. Effects of nisin on the antimicrobial activity of d-limonene and its nanoemulsion. Food Chem. 2014;150:307-12. [PubMed ID: 24360455]. https://doi.org/10.1016/j.foodchem.2013.10.160.
-
20.
Mohajer F, Khanzadi S, Keykhosravy K, Noori SMA, Azizzadeh M, Hashemi M. Impact of gelatin nanogel coating containing thymol and nisin on the microbial quality of rainbow trout fillets and the inoculated Listeria monocytogenes. Aquac Res. 2021;52(8):3958-65. https://doi.org/10.1111/are.15239.
-
21.
Shukla V, Mendiratta SK, Zende RJ, Agrawal RK, Kumar Jaiswal R. Effects of chitosan coating enriched with Syzygium aromaticum essential oil on quality and shelf‐life of chicken patties. J Food Process Preserv. 2020;44(11). https://doi.org/10.1111/jfpp.14870.
-
22.
Severino R, Vu K, Donsì F, Salmieri S, Ferrari G, Lacroix M. Antimicrobial effects of different combined non-thermal treatments against Listeria monocytogenes in broccoli florets. J Food Eng. 2014;124:1-10. https://doi.org/10.1016/j.jfoodeng.2013.09.026.
-
23.
Mahboubi M, Heidarytabar R, Mahdizadeh E, Hosseini H. Antimicrobial activity and chemical composition of Thymus species and Zataria multiflora essential oils. Agric Nat Resour. 2017;51(5):395-401. https://doi.org/10.1016/j.anres.2018.02.001.
-
24.
Sanei-Dehkordi A, Vatandoost H, Abaei MR, Davari B, Sedaghat MM. Chemical Composition and Larvicidal Activity ofBunium persicumEssential Oil Against Two Important Mosquitoes Vectors. J Essent Oil Bear Pl. 2016;19(2):349-57. https://doi.org/10.1080/0972060x.2015.1137240.
-
25.
Hashemi M, Ehsani A, Afshari A, Aminzare M, Raeisi M. Chemical composition and antifungal effect of Echinophora platyloba essential oil against Aspergillus flavus, Penicillium expansum and Fusarium graminearum. Journal of Chemical Health Risks. 2016;6(2). https://doi.org/10.22034/JCHR.2016.544133.
-
26.
Artiga-Artigas M, Acevedo-Fani A, Martín-Belloso O. Improving the shelf life of low-fat cut cheese using nanoemulsion-based edible coatings containing oregano essential oil and mandarin fiber. Food Control. 2017;76:1-12. https://doi.org/10.1016/j.foodcont.2017.01.001.
-
27.
Jafari SM, He Y, Bhandari B. Optimization of nano-emulsions production by microfluidization. Eur Food Res Technol. 2006;225(5-6):733-41. https://doi.org/10.1007/s00217-006-0476-9.
-
28.
Hatanaka J, Chikamori H, Sato H, Uchida S, Debari K, Onoue S, et al. Physicochemical and pharmacological characterization of alpha-tocopherol-loaded nano-emulsion system. Int J Pharm. 2010;396(1-2):188-93. [PubMed ID: 20558261]. https://doi.org/10.1016/j.ijpharm.2010.06.017.
-
29.
Salvia-Trujillo L, Rojas-Graü MA, Soliva-Fortuny R, Martín-Belloso O. Effect of processing parameters on physicochemical characteristics of microfluidized lemongrass essential oil-alginate nanoemulsions. Food Hydrocoll. 2013;30(1):401-7. https://doi.org/10.1016/j.foodhyd.2012.07.004.
-
30.
Sow L, Tirtawinata F, Yang H, Shao Q, Wang S. Carvacrol nanoemulsion combined with acid electrolysed water to inactivate bacteria, yeast in vitro and native microflora on shredded cabbages. Food Control. 2017;76:88-95. https://doi.org/10.1016/j.foodcont.2017.01.007.
-
31.
Yuan G, Chen X, Li D. Chitosan films and coatings containing essential oils: The antioxidant and antimicrobial activity, and application in food systems. Food Res Int. 2016;89(Pt 1):117-28. [PubMed ID: 28460897]. https://doi.org/10.1016/j.foodres.2016.10.004.
-
32.
Kanatt SR, Rao MS, Chawla SP, Sharma A. Effects of chitosan coating on shelf-life of ready-to-cook meat products during chilled storage. LWT - Food Sci Technol. 2013;53(1):321-6. https://doi.org/10.1016/j.lwt.2013.01.019.
-
33.
Kakaei S, Shahbazi Y. Effect of chitosan-gelatin film incorporated with ethanolic red grape seed extract and Ziziphora clinopodioides essential oil on survival of Listeria monocytogenes and chemical, microbial and sensory properties of minced trout fillet. LWT - Food Sci Technol. 2016;72:432-8. https://doi.org/10.1016/j.lwt.2016.05.021.
-
34.
Calo JR, Crandall P, O'Bryan CA, Ricke SC. Essential oils as antimicrobials in food systems – A review. Food Control. 2015;54:111-9. https://doi.org/10.1016/j.foodcont.2014.12.040.
-
35.
Shahbazi Y, Shavisi N. Characterization of active nanochitosan film containing natural preservative agents. Nanomed Res J. 2018;3(2):109-16. https://doi.org/10.22034/NMRJ.2018.02.008.
-
36.
Abdollahzadeh E, Rezaei M, Hosseini H. Antibacterial activity of plant essential oils and extracts: The role of thyme essential oil, nisin, and their combination to control Listeria monocytogenes inoculated in minced fish meat. Food Control. 2014;35(1):177-83. https://doi.org/10.1016/j.foodcont.2013.07.004.
-
37.
Abbasi Z, Aminzare M, Hassanzad Azar H, Rostamizadeh K. Effect of corn starch coating incorporated with nanoemulsion of Zataria multiflora essential oil fortified with cinnamaldehyde on microbial quality of fresh chicken meat and fate of inoculated Listeria monocytogenes. J Food Sci Technol. 2021;58(7):2677-87. [PubMed ID: 34194103]. [PubMed Central ID: PMC8196117]. https://doi.org/10.1007/s13197-020-04774-y.