Abstract
Background:
Cyclophosphamide (CP) is one of the most popular alkylating anticancer drugs despite its toxic side effects, including nephrotoxicity, hematotoxicity, mutagenicity, and immunotoxicity. Capparis spinosa is a multipurpose plant that contains a number of chemically active and diverse secondary metabolites, particularly flavonoids. Rutin and quercetin are two major flavonoids in the caper plant.Objectives:
This study was undertaken to investigate the protective effect of Capparis spinosa L. extract on nephrotoxicity induced by cyclophosphamide in mice.Methods:
In this experimental study, 40 male Swiss albino mice (20 - 25 g) were randomly divided into five groups with each group consisting of eight mice. Mice were pretreated with C. spinosa extract (CSE) orally in doses of 100, 200 and 400 mg/kg for five consecutive days, and CP (200 mg/kg, ip) was administrated on the fifth day 1 hour after the last dose of extract. The animals were sacrificed on the sixth day. Blood samples were collected to determine the serum creatinine (Cr) and blood urea nitrogen (BUN) levels. The malondialdehyde (MDA) and glutathione (GSH) levels were assayed in kidney tissue. The right kidney was maintained in 10% formalin for hematoxylin and eosin staining and histological examination.Results:
Different plant parts (fruit, leaves, and petals) were examined for antioxidant activity by 1,1-diphenyl-2-picrylhydrazyl assay, and leaf extract was used to determine nephroprotective effects. Results showed a significant increase in the levels of MDA, Cr, and BUN and a reduction of GSH by CP administration. Pre-treatment with CSE decreased the levels of MDA, Cr, and BUN. GSH increased in all doses, but the most significant alteration was observed in the doses of 200 and 400 mg/kg (P < 0.05). The nephroprotective effect of the CSE was confirmed by the histological examination of the kidneys.Conclusions:
Our results indicate that CSE ameliorates biochemical indices and oxidative stress parameters against CP-induced nephrotoxicity.Keywords
1. Background
Medicinal plants are an important source of new chemical substances with potential therapeutic effects (1-3). The genus of Capparis spinosa L. is an aromatic plant that originated from dry regions in West or Central Asia and is distributed particularly across the Mediterranean basin. Its fruit, roots, and barks are used for medical purposes. In traditional medicine, this plant is used as a diuretic and for the treatment of gout, rheumatism, and liver disease (4). Previously, the analgesic, anti-inflammatory, anti-hepatotoxicity, hypolipidemic, and anti-allergic effects of this plant have been reported (5-9). Research on C. spinosa reveals the presence of such compounds as alkaloid, lipids, polyphenols, flavonoids, and glucosinolates (10). It also has certain flavonoids, such as kaempferol, rutin, and quercetin (11-13), and thus this plant used as a remedial source. The medicinal properties of C. Spinosa (caper) are due to its valuable compounds and antioxidant properties (14).
Cyclophosphamide (CP) is a well-known bifunctional alkylating agent that transfers alkyl residues to a covalent bond with DNA, is widely used in cancer chemotherapy, and expresses its genotoxicity when metabolically activated (15). This compound is commonly used for the treatment of various cancers and is an immunosuppressant in organ transplantation, rheumatoid arthritis, systemic lupus erythematosus, multiple sclerosis, and other benign diseases (16). The reactive metabolites of CP chemically alkylate DNA and protein and produce cross-links, which are responsible for its cytotoxic effect (17). Using CP also leads to the production of reactive oxygen species (ROS), which results in peroxidative damage to the kidney and other vital organs (18). Studies have reported that CP treatment for non-Hodgkin’s lymphoma leads to the induction of secondary cancers in bladder and kidney (19). Furthermore, this drug or its metabolites are known to cause acute inflammation of the urinary bladder and may trigger renal damage (20, 21).
2. Objectives
This study aimed to evaluate the protective effects of the hydroalcoholic extract of C. spinosa L. on CP-induced nephrotoxicity.
3. Methods
3.1. Chemicals
5, 5-Dithiobis (2-nitrobenzoic acid) (DTNB), reduced glutathione (GSH), trichloro acetic acid (TCA), thiobarbituric acid (TBA), bovine serum albumin (BSA), and Bradford reagent were purchased from sigma-aldrich chemical company (St. Louis, MO, USA). All chemicals and reagents used were of analytical grade. CP was purchased from roche chemical company (Germany).
3.2. Extract Preparation
Fresh and ripe fruits, leaves, and petals of C. spinosa L. were collected from Khuzestan Province, Iran, in spring of 2015. Samples of the plant were identified by a botanist from the division of pharmacognosy, Ahvaz Jundishapur University of Medical Sciences. The plant material was shade dried, powdered, and soaked in a 70% aqueous ethanol solution in a large container for 3 days with occasional shaking. The solvent was filtered through a Whatman paper and then removed under vacuum in a rotary evaporator until dry. The percentage yield was 16% for a dried hydroalcoholic extract (w/w) (22, 23).
3.3. Radical Scavenging Capacity of CSE by 1,1-Diphenyl-2-Picrylhydrazyl (DPPH) Assay
The percentage of antioxidant activity (AA%) of each substance was assessed by DPPH assay. The DPPH radical scavenging activity was measured according to the method described by Brand-Williams et al. (24). The samples were reacted with the stable DPPH radical in an ethanol solution. The reaction mixture consisted of 0.5 mL of the sample, 3 mL of absolute ethanol, and 0.3 mL of the DPPH radical solution in 0.5 mM ethanol. DPPH is reduced when it reacts with an antioxidant compound, which can donate hydrogen. The changes in color (from deep violet to light yellow) were read [(Absorbance) Abs)] at 517 nm after 100 minutes of reaction using an ultraviolet-visible spectrophotometer (DU 800; Beckman Coulter, Fullerton, CA, USA). The mixture of ethanol (3.3 mL) and the sample (0.5 mL) served as blank. The control solution was prepared by mixing ethanol (3.5 mL) and the DPPH radical solution (0.3 mL). AA% was determined according to Mensor et al. (25).
3.4. Animals
Male Swiss albino mice (6 - 8 weeks old, 20 - 25 g) were obtained from the animal house of Ahvaz Jundishapur University of Medical Science, Iran. Mice were kept in polypropylene cages and given standard rat chow and drinking water ad libitum. The animals were maintained at a controlled temperature (20 ± 2°C) with a 12 hour light: 12 hour dark cycle. The animals were acclimated to the environment for a minimum of one week prior to inclusion in the experiment. The investigation was conducted according to the animal ethics committee guidelines for the use of experimental animals (ethics approval number: 1384.10.23)
3.5. Experimental Design
The animals were divided in to five groups with each group consisting of eight mice. As the negative control group, Group 1 received normal saline for five days; group 2 received CP (200 mg/kg, ip) (26) as the positive control only on the fifth day; groups 3 - 5 received C. spinosa extract (CSE) orally in doses of 100, 200 and 400 mg/kg, respectively, on five days and CP (200 mg/kg, ip) on the fifth day 1 hour after the last dose of extract administration.
3.6. Sample Collection
On the sixth day, 24 hours after the last administration, the animals were anaesthetized with diethyl ether, and blood samples were collected from the jugular vein. Serum was separated by centrifugation for 10 min at 3000 rpm and stored at -20 °C until analysis. Then, the animals were sacrificed by decapitation, and the kidneys were isolated and quickly washed with saline. For the histological examination, the right kidney was fixed in 10% phosphate-buffered formalin.
The left kidney was weighed and homogenized (1/10 w/v) in ice-cold Tris-HCl buffer (0.1M, pH 7.4). Protein content in the homogenates was measured by the method of Bradford (27) using crystalline BSA as standard.
3.7. Serum Analysis
Creatinine (Cr) and blood urine nitrogen (BUN) levels were measured using the RA-XT (automated) biochemical analyzer with their respective test kits (Technicon, Bayer S.A. Diagnostic).
3.8. GSH Assay
The levels of GSH in the tissue homogenate was measured following the method described by Ellman (28) based on the formation of a yellow colored complex with Ellman’s Reagent (DTNB). The homogenates were immediately precipitated with 0.1 mL of 25% TCA, and the precipitate was removed after centrifugation. Free endogenous-SH was assayed in a 3 mL volume glass with the addition of 2 mL of 0.5 mM DTNB prepared in 0.2 M phosphate buffer (pH = 8) to 0.1 mL of the supernatant. The yellow color that developed was read at 412 nm using a spectrophotometer (UV-1650 PC, Shimadzu, Japan). GSH content was expressed as nmol/mg protein.
3.9. Lipid Peroxidation Assay
Lipid peroxidation was expressed by measuring the amounts of malondialdehyde (MDA) through the TBA color reaction by the modified method of Buege and Aust (29). Briefly, 0.5 mL of kidney homogenate was mixed with 2.5 ml of TCA (10%, w/v). The samples were centrifuged at 1500 g for 10 minutes, and 2 mL of each sample supernatant was transferred to a test tube containing 1 mL of TBA solution (0.67%, w/v). The mixture was kept in boiling water for 10 minutes, forming a pink color solution. The mixture was then cooled immediately, and absorbance was measured at 532 nm by a spectrophotometer (UV-1650 PC, Shimadzu, Japan). The concentration of MDA was calculated on the basis of the absorbance coefficient of the TBA-MDA complex (ε = 1.56 × 105 cm-1 M-1), and it was expressed as nmol/mg protein.
3.10. Histopathological Assessments
For the histological examination, the kidneys were fixed in 10% formalin for at least 24 hours. Then, kidney tissues were dehydrated with a sequence of ethanol solutions, embedded in paraffin, cut into 5 µm sections, and stained with hematoxylin and eosin dye (H & E stain). These sections were then examined under a photomicroscope for the presence of necrosis, edema, hemorrhage, tubular degeneration, mononuclear/polymorphonuclear cell infiltration, and narrowing of Bowman’s capsule space (30).
3.11. Statistical Analysis
The results were expressed as mean ± SD, and all statistical comparisons were made by the one-way ANOVA test and Tukey’s post hoc analysis. A P value less than 0.05 was considered significant.
4. Results
The results of the antioxidant activities assay showed that the leaf extract had the lowest IC50 (61.55 µg/mL), followed by the petal extract (85.76 µg/mL) and the fruit extract (100.19 µg/mL). The leaf extract was chosen to evaluate the nephroprotective effects because of its low IC50.
Exactly 24 hours after CP administration, the mice developed severe nephrotoxicity, which was reflected by the significant increase (P < 005) in the BUN and Cr levels (Table 1). Pre-treated groups with CSE showed a decrease in the BUN and Cr levels in all doses, but the levels significantly decreased in the 400 mg/kg dose.
Effects of pretreatment with Capparis spinosa extract (CSE) on the serum levels of creatinine (Cr) and BUN in cyclophosphamide (CP)-induced nephrotoxicity
Table 2 shows the effect of CP and CSE pretreatment on the MDA and GSH levels in kidney tissue. The results clearly revealed that CP intoxication resulted in a marked increase in the amount of MDA in rats (P < 0.05). On the contrary, pre-treatment with CSE caused a diminished level of MDA compared with the CP group. Clearly, CP exposure resulted in the reduction of reduced GSH levels in kidney tissue. CSE pre-treatment increased the levels of reduced GSH levels in a dose-dependent manner.
Effects of pretreatment with Capparis spinosa extract (CSE) on tissue MDA and GSH levels in cyclophosphamide (CP)-induced nephrotoxicity
The histopathological study of the kidneys in the negative control group showed a normal architecture. In this group, the structure of glomerular was clear, capsular space was small, the structure of the epithelial cells in the proximal convoluted and distal convoluted tubules was normal, and the boundaries of the visceral and parietal layers of the renal capsule were clear (Figure 1A). In the CP-intoxicated group, the glomerular were atrophied and disintegrated, the capsular spaces clearly widened, the visceral and parietal layers of the renal capsule were destroyed with some even disappearing, the epithelial cells in the proximal convoluted and distal convoluted tubules were swollen with some epithelial cells having vacuolization, the structure of the epithelial cells was unclear, and some cell fragments were found in the tubules (Figure 1B).
Histopathological observations (kidney sections stained with H&E, magnification x 100) showing the effects of CSE on CP-induced nephrotoxicity changes in mouse kidney. (A) Normal, (B) CP-treated group, (C), (D), and (E) are CP group pre-treated with 100, 200 and 400 mg/kg of CSE, respectively.
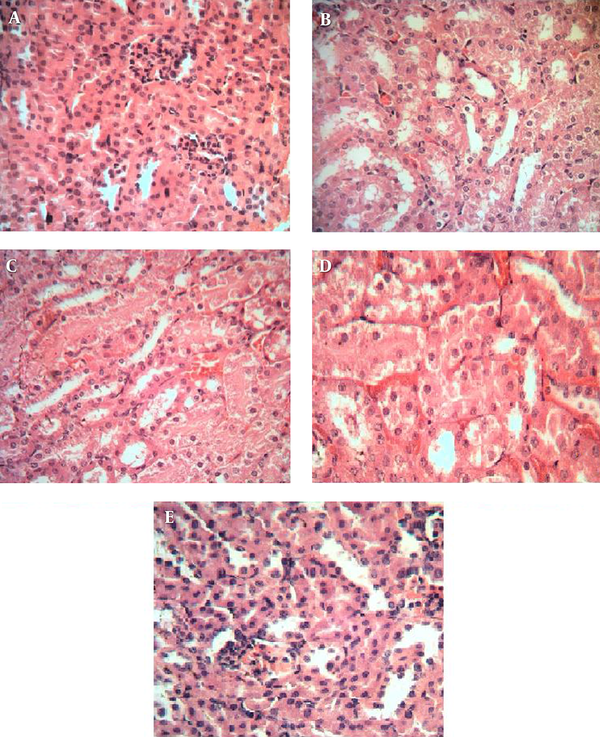
Pre-treatment with the CSE at the doses of 200 and 400 mg/kg showed considerable improvement in the proximal and distal convoluted tubules (Figure 1D, E). The atrophy degree of glomeruli decreased, the capsular space was smaller in comparison with that of the CP-treated group (positive control), the boundaries of the visceral and parietal layers of the renal capsule were clear, the epithelial cells in the proximal convoluted and distal convoluted tubules were slightly swollen, and cell fragments were visible in some tubules.
5. Discussion
Kidneys play a principal role in the excretion of metabolic wastes and in the regulation of extracellular fluid volume, electrolyte composition, and acid–base balance (31). Toxic effects on the kidneys related to medications are both common and expected, and can disrupt any or all of these functions (32). A wide variety of drugs, environmental chemicals, and metals constitute important causes of acute renal failure and chronic kidney disease (33, 34). Nephrotoxicity due to CP has been well documented in both humans and animals (35).
CP leads to the toxicity of renal cells because of its toxic metabolites. The two active metabolites of CP is phosphoramide mustard (PAM) and acrolein (ACR). CP antineoplastic effects are associated with PAM, and ACR is responsible for its toxic side effects (36).
ACR causes cellular damage after binding with GSH and reduces its level in the cell. It impairs the GSH-dependent antioxidant system and increases free radical generation (37). ACR interferes with the tissue antioxidant defense system and results in the necrosis of tubular epithelial cells (38-40). Antioxidant agents detoxify the toxic effect of ACR.
Therefore, understanding the role played by antioxidants agents during drug-mediated toxicity is important to determine if they have a protective effect against oxidative stress induced by reactive intermediates (41, 42).
C. spinosa is a multipurpose plant that contains a number of chemically active and diverse secondary metabolites, particularly flavonoids. Rutin and quercetin are two major flavonoids in the caper plant. Moghaddasian et al. developed an HPLC method for the simultaneous determination of rutin and quercetin contents in different parts of C. spinosa at the floral budding stage.
The highest amount of rutin (25.82 mg/g) and quercetin (10.4 mg/g) was measured in the leaf of the caper plant (13). The significant amounts of these antioxidants confirmed the nutritional and medicinal value of caper. Ramezani et al. showed that the amounts of rutin in the leaves, fruits, and flowers of C. spinosa that grow wild in Khuzestan, Iran, were 61.09, 6.03 and 43.72 mg per 100 g of dried powder, respectively (12).
The results of our study showed that the leaf, petal, and fruit extracts of C. spinosa have the highest antioxidant activities, respectively.
C. spinosa has been reported to possess profound anti-inflammatory and antioxidant activities. Furthermore, it protects against several models of oxidative stress (6, 43). These properties motivate us to examine the protective effects of this extract in nephrotoxicity induced by CP.
In a similar study, Ayhanci et al. investigated the protective effects of seleno L-methionine (SLM) in nephrotoxicity induced by CP. They reported that intraperitoneal injection of SLM for six days restored the GSH values close to the control group, and further microscopic observations confirmed their work (44).
Lavin et al. investigated the adverse effects of CP on various organs in rats. They reported that a single dose of CP (200 mg/kg, intraperitoneal) caused the necrosis of the tubular epithelium in the experimental animals (38).
BUN and Cr are two of the conventional test indices for kidney functions and renal structural integrity. In our study, increased Cr and BUN levels in the CP-treated mice showed renal toxicity. This elevation in the Cr and BUN levels could be due to the destruction generated in the kidney tubules established by the marked changes in kidney tissues in comparison with the control group.
Pre-treatment with CSE significantly decreased the BUN and Cr levels in the CP-treated mice.
MDA is one of the most common markers of lipid peroxidation. Lipid peroxidation is a well-known mechanism of cellular injury in the human body. MDA is an extremely reactive three-carbon dialdehyde and the main oxidative degradation product of a membrane unsaturated fatty acid because of its toxic attributes. As CP toxicity causes reactive oxygen metabolites in many tissues, specifically those of kidneys, measuring the MDA level is valuable in the diagnosis of CP-induced toxicity.
In the present study, CSE pre-treatment significantly decreased MDA formation because of ROS in mice treated with CP. Pre-treatment with CSE restored the MDA level. This finding suggests that CSE may be successful in quenching free radicals, thus inhibiting LPO and protecting against membrane damage from oxidative damage in mice. Our results are consistent with those of some studies indicating CP intoxication, significant depletion of the GSH level, and significant increase in the MDA, BUN, and Cr levels, thus corroborating the state of oxidative stress (20, 45-47).
Furthermore, the protective effects of CSE were confirmed by the histopathological examination of the kidneys. Considerable improvement in the proximal and distal convoluted tubules and atrophy of the glomerular in pretreated groups were found. Our results demonstrate that the protective effects of CSE are dose dependent as the best results were observed in the doses of 200 and 400 mg/kg. We believe that this protective effect may be mediated by the antioxidant and GSH preservation effects of CSE. In conclusion, the results of the present study indicate that the hydroalcoholic extract of C. spinosa has protective effects against CP-induced nephrotoxicity in mice.
Acknowledgements
References
-
1.
Kalantari H, Nazari Z, Keliddar A, Foruozandeh H, Kalantar M. Study of the protective effect of livergol against liver toxicity caused by bromobenzene in mice. Iranian J Pharmaceutical Sci. 2015;10(2):11-20.
-
2.
Javad-Mousavi SA, Hemmati AA, Mehrzadi S, Hosseinzadeh A, Houshmand G, Rashidi Nooshabadi MR, et al. Protective effect of Berberis vulgaris fruit extract against Paraquat-induced pulmonary fibrosis in rats. Biomed Pharmacother. 2016;81:329-36. [PubMed ID: 27261610]. https://doi.org/10.1016/j.biopha.2016.04.027.
-
3.
Kalantari H, Mombeyni M, Dianat M, Badavi M, Goudarzi M. The Effect of Prunus cerasus (Sour Cherry) Kernel Seed Extract on QT Interval of Heart and its Histopathology in Biliary Cirrhosis Induced by Bile Duct Ligation in Rats. Jundishapur J Nat Pharm Prod. 2015;10(4).
-
4.
Tlili N, Elfalleh W, Saadaoui E, Khaldi A, Triki S, Nasri N. The caper (Capparis L.): ethnopharmacology, phytochemical and pharmacological properties. Fitoterapia. 2011;82(2):93-101. [PubMed ID: 20851750]. https://doi.org/10.1016/j.fitote.2010.09.006.
-
5.
Liu HJ, Yang T, Cheng XM, Wang CH. Comparative evaluation of anti-inflammatory and analgesic activities of various medicinal parts of capparis spinosa: A consideration of ecological environment and resource conservation. Indian J Pharmaceutical Sci Res. 2014;4(1):53-9.
-
6.
Bonina F, Puglia C, Ventura D, Aquino R, Tortora S, Sacchi A, et al. In vitro antioxidant and in vivo photoprotective effects of a lyophilized extract of Capparis spinosa L buds. J Cosmet Sci. 2002;53(6):321-35. [PubMed ID: 12512010].
-
7.
Rahmani R, Mahmoodi M, Karimi M, Hoseini F, Heydari R, Salehi M. Effect of hydroalcoholic extract of capparis spinosa fruit on blood sugar and lipid profile of diabetic and normal rats. Zahedan J Res Med Sci. 2013;15(11):34-8.
-
8.
Aghel N, Rashidi I, Mombeini A. Hepatoprotective activity of Capparis spinosa root bark against CCl4 induced hepatic damage in mice. Iranian J Pharmaceutical Res. 2010:285-90.
-
9.
Eddouks M, Lemhadri A, Michel JB. Hypolipidemic activity of aqueous extract of Capparis spinosa L. in normal and diabetic rats. J Ethnopharmacol. 2005;98(3):345-50. [PubMed ID: 15814271]. https://doi.org/10.1016/j.jep.2005.01.053.
-
10.
Brevard H, Brambilla M, Chaintreau A, Marion J, Diserens H. Occurrence of elemental sulphur in capers (Capparis spinosa L.) and first investigation of the flavour profile. Flavour Frag J. 1992;7(6):313-21.
-
11.
Sharaf M, el-Ansari MA, Saleh NA. Quercetin triglycoside from Capparis spinosa. Fitoterapia. 2000;71(1):46-9. [PubMed ID: 11449469].
-
12.
Ramezani Z, Aghel N, Keyghobadi H. Rutin from different parts of Capparis spinosa growing wild in Khuzestan/Iran. Pak J Biol Sci. 2008;11(5):768-72. [PubMed ID: 18819575].
-
13.
Moghaddasian B, Eradatmand A, Alaghemand A. Simultaneous determination of rutin and quercetin in different parts of Capparis spinosa. Bull Environ Pharmacol Life Sci. 2013;2:35-8.
-
14.
Prakash D, Suri S, Upadhyay G, Singh BN. Total phenol, antioxidant and free radical scavenging activities of some medicinal plants. Int J Food Sci Nutr. 2007;58(1):18-28. [PubMed ID: 17415953].
-
15.
Baumann F, Preiss R. Cyclophosphamide and related anticancer drugs. J Chromatogr B Biomed Sci Appl. 2001;764(1-2):173-92. [PubMed ID: 11817027].
-
16.
Uber WE, Self SE, Van Bakel AB, Pereira NL. Acute antibody-mediated rejection following heart transplantation. Am J Transplant. 2007;7(9):2064-74. [PubMed ID: 17614978]. https://doi.org/10.1111/j.1600-6143.2007.01900.x.
-
17.
Hales BF. Comparison of the mutagenicity and teratogenicity of cyclophosphamide and its active metabolites, 4-hydroxycyclophosphamide, phosphoramide mustard, and acrolein. Cancer Res. 1982;42(8):3016-21. [PubMed ID: 7046914].
-
18.
Patel JM. Stimulation of cyclophosphamide-induced pulmonary microsomal lipid peroxidation by oxygen. Toxicology. 1987;45(1):79-91. [PubMed ID: 3603576].
-
19.
Travis LB, Curtis RE, Glimelius B, Holowaty EJ, Van Leeuwen FE, Lynch CF, et al. Bladder and kidney cancer following cyclophosphamide therapy for non-Hodgkin's lymphoma. J Natl Cancer Inst. 1995;87(7):524-30. [PubMed ID: 7707439].
-
20.
Abraham P, Isaac B. The effects of oral glutamine on cyclophosphamide-induced nephrotoxicity in rats. Hum Exp Toxicol. 2011;30(7):616-23. [PubMed ID: 20621952]. https://doi.org/10.1177/0960327110376552.
-
21.
Lawson M, Vasilaras A, De Vries A, Mactaggart P, Nicol D. Urological implications of cyclophosphamide and ifosfamide. Scand J Urol Nephrol. 2008;42(4):309-17. [PubMed ID: 18781541]. https://doi.org/10.1080/00365590701570953.
-
22.
Kalantari H, Jalali M, Jalali A, Mahdavinia M, Salimi A, Juhasz B, et al. Protective effect of Cassia fistula fruit extract against bromobenzene-induced liver injury in mice. Hum Exp Toxicol. 2011;30(8):1039-44. [PubMed ID: 20930029]. https://doi.org/10.1177/0960327110386256.
-
23.
Salimi A, Motaharitabar E, Goudarzi M, Rezaie A, Kalantari H. Toxicity evaluation of microemulsion (nano size) of sour cherry kernel extract for the oral bioavailability enhancement. Jundishapur J Nat Pharm Prod. 2014;9(1):16-23. [PubMed ID: 24644434].
-
24.
Brand-Williams W, Cuvelier ME, Berset CLWT. Use of a free radical method to evaluate antioxidant activity. Food Sci Technol. 1995;28(1):25-30.
-
25.
Mensor LL, Menezes FS, Leitao GG, Reis AS, dos Santos TC, Coube CS, et al. Screening of Brazilian plant extracts for antioxidant activity by the use of DPPH free radical method. Phytother Res. 2001;15(2):127-30. [PubMed ID: 11268111].
-
26.
El-Naggar SA, Alm-Eldeen AA, Germoush MO, El-Boray KF, Elgebaly HA. Ameliorative effect of propolis against cyclophosphamide-induced toxicity in mice. Pharm Biol. 2015;53(2):235-41. [PubMed ID: 25289525]. https://doi.org/10.3109/13880209.2014.914230.
-
27.
Bradford MM. A rapid and sensitive method for the quantitation of microgram quantities of protein utilizing the principle of protein-dye binding. Anal Biochem. 1976;72(1-2):248-54.
-
28.
Ellman GL. Tissue sulfhydryl groups. Arch Biochem Biophys. 1959;82(1):70-7. [PubMed ID: 13650640].
-
29.
Buege JA, Aust SD. Microsomal lipid peroxidation. Methods Enzymol. 1978;52:302-10. [PubMed ID: 672633].
-
30.
Kalantari H, Jalali M, Jalali A, Salimi A, Alhalvachi F, Varga B, et al. Protective effect of Cassia fistula fruit extract on bromobenzene-induced nephrotoxicity in mice. Hum Exp Toxicol. 2011;30(10):1710-5. [PubMed ID: 21247991]. https://doi.org/10.1177/0960327110396532.
-
31.
Doull C. Casarett And Doull's Toxicology, the basic science of poisons. New York: McGraw-Hill; 2008.
-
32.
Choudhury D, Ahmed Z. Drug-associated renal dysfunction and injury. Nat Clin Pract Nephrol. 2006;2(2):80-91. [PubMed ID: 16932399]. https://doi.org/10.1038/ncpneph0076.
-
33.
Joy J, Nair CK. Amelioration of cisplatin induced nephrotoxicity in Swiss albino mice by Rubia cordifolia extract. J Cancer Res Ther. 2008;4(3):111-5. [PubMed ID: 18923202].
-
34.
Singh NP, Ganguli A, Prakash A. Drug-induced kidney diseases. J Assoc Physicians India. 2003;51:970-9. [PubMed ID: 14719587].
-
35.
Lopes VM. Cyclophospharnide nephrotoxicity in man. Lancer. 1967;1:1060.
-
36.
Singh M, Kumar N, Shuaib M, Garg VK, Sharma A. A review on renal protective agents for cyclophosphamide induced nephrotoxicity. World J Pharm Pharmaceut Sci. 2014;3:737-47.
-
37.
Ohno Y, Ormstad K. Formation, toxicity and inactivation of acrolein during biotransformation of cyclophosphamide as studied in freshly isolated cells from rat liver and kidney. Arch Toxicol. 1985;57(2):99-103. [PubMed ID: 4026579].
-
38.
Lavin P, Koss LG. Effects of a single dose of cyclophosphamide on various organs in the rat. IV. Electron microscopic study of the renal tubules. Am J Pathol. 1971;62(2):169-80. [PubMed ID: 5540656].
-
39.
Arumugam N, Sivakumar V, Thanislass J, Devaraj H. Effects of acrolein on rat liver antioxidant defense system. Indian J Exp Biol. 1997;35(12):1373-4. [PubMed ID: 9567773].
-
40.
Slater CA, Liang MH, McCune JW, Christman GM, Laufer MR. Preserving ovarian function in patients receiving cyclophosphamide. Lupus. 1999;8(1):3-10. [PubMed ID: 10025593].
-
41.
Sohn JH, Han KL, Choo JH, Hwang JK. Macelignan protects HepG2 cells against tert-butylhydroperoxide-induced oxidative damage. Biofactors. 2007;29(1):1-10. [PubMed ID: 17611289].
-
42.
Wu Y, Li L, Wen T, Li YQ. Protective effects of echinacoside on carbon tetrachloride-induced hepatotoxicity in rats. Toxicology. 2007;232(1-2):50-6. [PubMed ID: 17222497]. https://doi.org/10.1016/j.tox.2006.12.013.
-
43.
Germano MP, De Pasquale R, D'Angelo V, Catania S, Silvari V, Costa C. Evaluation of extracts and isolated fraction from Capparis spinosa L. buds as an antioxidant source. J Agric Food Chem. 2002;50(5):1168-71. [PubMed ID: 11853498].
-
44.
Ayhanci A, Gunes S, Sahinturk V, Appak S, Uyar R, Cengiz M, et al. Seleno L-methionine acts on cyclophosphamide-induced kidney toxicity. Biol Trace Elem Res. 2010;136(2):171-9. [PubMed ID: 19826776]. https://doi.org/10.1007/s12011-009-8535-2.
-
45.
Premkumar K, Pachiappan A, Abraham SK, Santhiya ST, Gopinath PM, Ramesh A. Effect of Spirulina fusiformis on cyclophosphamide and mitomycin-C induced genotoxicity and oxidative stress in mice. Fitoterapia. 2001;72(8):906-11. [PubMed ID: 11731115].
-
46.
Chamorro-Cevallos G, Garduno-Siciliano L, Barron BL, Madrigal-Bujaidar E, Cruz-Vega DE, Pages N. Chemoprotective effect of Spirulina (Arthrospira) against cyclophosphamide-induced mutagenicity in mice. Food Chem Toxicol. 2008;46(2):567-74. [PubMed ID: 17928122]. https://doi.org/10.1016/j.fct.2007.08.039.
-
47.
Sinanoglu O, Yener AN, Ekici S, Midi A, Aksungar FB. The protective effects of spirulina in cyclophosphamide induced nephrotoxicity and urotoxicity in rats. Urology. 2012;80(6):1392 e1-6. [PubMed ID: 22951000]. https://doi.org/10.1016/j.urology.2012.06.053.