Abstract
Background:
The Artemisia genus is well-known for its medicinal properties, particularly in Iranian traditional medicine. Artemisia biennis, a species within this genus, is widely distributed across Iranian rangelands.Objectives:
This study aimed to evaluate the cytotoxic activity and chemical composition of essential oils (EO) isolated from the aerial parts of A. biennis in Iran at different growth stages.Methods:
The aerial parts of A. biennis from northeast Iran were collected during June (early vegetative stage), July (pre-flowering stage), August (full-flowering stage), and October (late vegetative stage). The essential oils (EOs) of the A. biennis species were extracted by hydro-distillation using a Clevenger-type apparatus and analyzed using gas chromatography–mass spectrometry (GC/MS). The cytotoxic activity of the EOs against normal fibroblasts, MCF-7, and HT-29 cell lines was evaluated using the MTT assay.Results:
Data from GC/MS analysis revealed that (Z)-nerolidol (22.62 - 54.4%), (E)-β-farnesene (6.89-16.38%), and (Z)-tonghaosu (12.33 - 18.61%) were the most abundant chemical constituents. The essential oil (EO) of A. biennis was characterized by a high content of oxygenated sesquiterpenes, with (Z)-nerolidol being the main constituent identified. Based on the MTT assay, the EO of the plant species collected in June exhibited the most potent cytotoxicity against MCF-7 (IC50 = 2.84 ± 0.15 µg/mL) and HT-29 cell lines (IC50 = 2.41 ± 0.2 µg/mL).Conclusions:
The growth stage of A. biennis affects EO yields, the composition of extracted secondary metabolites, and cytotoxic activity. A. biennis EOs can be considered potential sources of cytotoxic phytochemicals.Keywords
Artemisia biennis Growth Stages Nerolidol Tonghaosu Farnesene Cytotoxic Activity
1. Background
The Artemisia genus (family Asteraceae) contains more than 498 species worldwide, primarily distributed across Europe, Asia, and North America (1, 2). In Iran, this genus includes 34 species that grow wild in dry or semi-dry habitats (1, 2). The phytochemicals reported from Artemisia include flavonoids, coumarins, sterols, polyacetylenes, monoterpenes, sesquiterpenes, and sesquiterpene lactones (1-4). Several Artemisia species have been documented to possess antiviral, anti-inflammatory, anti-helminthic, antihepatotoxic, anti-malarial, antiseptic, cytotoxic, and antispasmodic activities (5-7). Therefore, identifying the secondary metabolites in these species could open new avenues for understanding the biological effects and nutritional properties of this herb.
Artemisia biennis, known as "Dermaneye dosaaleh" in Persian, is distributed across various regions of Iran (8). Previous studies in Iran have reported the presence of α-pinene (10.2%), 1,8-cineole (10.1%), Artemisia ketone (11.4%), and camphor (24.6%) as the main components of A. biennis essential oil (EO) (9). Lopes-Lutz et al. identified nearly 37 compounds in A. biennis EO from Canada, with the highest percentages attributed to (E)-β-farnesene (40%), (Z)-β-ocimene (34.7%), and (Z)-en-yn-dicycloether (11%) (10). Due to its high activity against dermatophytes, Cryptococcus neoformans, Fonsecaea pedrosoi, and Aspergillus niger, A. biennis EO could be a promising candidate for skin and hair care formulations (10). Additionally, the EO of A. biennis has demonstrated antibacterial activity against Staphylococcusaureus, Lactobacillus plantarum, and Escherichia coli (11). The EO from the aerial parts of A. biennis also showed significant analgesic effects in male rats, potentially involving the modulation of glutamatergic mechanisms through opioid systems (12).
Mohammadi et al. demonstrated that the vegetative state could quantitatively and qualitatively affect the EO of A. absinthium (13). The cytotoxic effects of dichloromethane extract fractions from A. biennis against MCF-7 and human prostate carcinoma (PC3) cancer cell lines have been previously reported, suggesting this plant as a potential source of cytotoxic phytochemicals (7). Therefore, a phytochemical study on the aerial parts of A. biennis, a plant growing in Iran, seems reasonable to investigate its ecological importance, nutritional value for animal feed, and to complete the study on the secondary metabolites of its EO.
2. Objectives
While A. biennis essential oils (EOs) have been studied for their biological properties, research specifically focusing on the effect of different growth stages on the chemical composition of the EO and its toxicity performance has not been previously conducted. Therefore, the primary aim of this study was to identify the chemical composition of EOs isolated from the aerial parts of A. biennis during the early vegetative stage, pre-flowering stage, full-flowering stage, and late vegetative stage from the mountainous areas of Iran for the first time. Additionally, this research aimed to evaluate the cytotoxicity of the obtained A. biennis EOs at different growth stages against various cancerous cell lines.
3. Methods
3.1. Materials
The cell culture media and supplements, including Dulbecco’s modified Eagle’s medium (DMEM-F12), fetal bovine serum (FBS), 3-(4,5-dimethylthiazol-2-yl)-2,5-diphenyl tetrazolium bromide (MTT), and penicillin-streptomycin, were obtained from Bonyakhteh (Iran). The human colon adenocarcinoma (HT-29), breast adenocarcinoma (MCF-7), and normal fibroblast cell lines were obtained from the Pasteur Institute (Tehran, Iran).
3.2. Preparation of Essential Oils
The aerial parts of A. biennis were collected from the mountainous areas of Jaghargh (36°18′43″N 59°19′21″E), Mashhad, Iran, in June (early vegetative stage), July (pre-flowering stage), August (full-flowering stage), and October (late vegetative stage) of 2021. The samples were identified and an herbarium specimen (No. 13605) was deposited in the herbarium of the School of Pharmacy at Mashhad University of Medical Sciences (Mashhad, Iran).
The aerial parts of the plant were washed with deionized water, dried at room temperature, and then finely powdered using an electric mill. The essential oil was extracted from the A. biennis powder through hydro-distillation using an all-glass Clevenger-type apparatus (14). Thirty grams of plant material were soaked in 300 mL of distilled water, placed in a glass flask, and heated for 3 hours at 100°C. Anhydrous sodium sulfate was used to remove any remaining water after extraction. The EO was stored in an airtight glass container at 4°C, and the EO yields were calculated as a percentage of the dry weight (% w/w). The extraction was performed three times, and the mixed EOs from each growth stage were then analyzed by GC/MS.
3.3. Gas Chromatography–mass Spectrometry (GC/MS) Analysis
Gas chromatography–mass spectrometry analysis was performed using an Agilent Technologies 7890B GC System/5977A MSD (USA) equipped with a fused silica capillary HP-5 column (30 m × 0.25 mm, 0.25 μm film thickness). Helium was used as the carrier gas at a flow rate of 1 mL per minute. The process was conducted under the following conditions: Injection volume of 1 µL with a split ratio of 1:20, injector temperature set at 280°C, and the oven temperature programmed to increase from 30°C to 280°C at a rate of 3°C per minute. Mass spectra were obtained in electron impact (EI+) mode at 70 eV, with the ion source temperature set at 230°C. Mass spectra were recorded over the 50 - 500 a.m.u. range (15). Retention indices were calculated relative to the retention times of n-alkanes (C8-C24). The EO components were identified by comparing their Kovats indices with those reported in the literature and by referencing internal libraries (Wiley, NIST, and Mass Finder 2.1 GC/MS libraries) (16).
3.4. Cytotoxicity Test
3.4.1. Cell Cultures
The HT-29, MCF-7, and normal fibroblast cell lines were maintained in DMEM-F12 medium supplemented with 10% v/v fetal bovine serum, 100 U/mL penicillin, and 100 μg/mL streptomycin at 37°C in a humidified atmosphere containing 5% CO2. The cells were sub-cultured two to three times per week (17, 18).
3.4.2. Cytotoxicity Evaluation Using MTT Assay
The MTT assay was used to investigate the toxicity of the EOs, following a standard method previously reported (17, 18). A cell suspension with a concentration of 5 × 103 cells/mL was plated in each well of 96-well plates, and 100 μL of DMEM-F12 culture medium containing 10% FBS was added to each well. The plates were incubated for 24 hours at 37°C, with 90% humidity and 5% CO2. Various concentrations of the EOs, prepared through serial dilution (100, 50, 25, 12.5, and 6.25 µg/mL), were then added to the wells and incubated for an additional 24 hours. A 1% dimethyl sulfoxide (DMSO) solution was used as a positive control. The medium was then replaced with 100 μL of MTT solution (0.5 mg/mL), followed by a further 4-hour incubation period. After removing the solution, DMSO was added to dissolve the formazan product. The color intensity, which is proportional to the number of living cells, was measured using an ELISA plate reader (BioTek, ELx800™, USA) at a wavelength of 570 nm. Each assay was performed in triplicate, and the mean and standard error of the mean (SEM) were calculated. The IC50 values were determined using Prism software.
3.5. Statistical Analysis
Each experiment was performed three times, and the results were presented as means ± SEM. A one-way analysis of variance (ANOVA) was used to compare the differences between means. A probability value of P < 0.05 was considered statistically significant.
4. Results and Discussion
4.1. Phytochemical Analysis
The aerial parts of the Artemisia genus have been used in Iranian traditional medicine for therapeutic purposes, primarily because most essential oil components accumulate in the aerial parts of the plant (5, 8, 12). Therefore, this study focused on investigating the EOs of A. biennis aerial parts at different growth stages. The average EO yields ranged from 0.29 ± 0.08% in October to 0.5 ± 0.021% in August (Table 1), following the sequence: Late vegetative stage (0.29 ± 0.08%) < early vegetative stage (0.36 ± 0.02%) < pre-flowering stage (0.4 ± 0.014%) < full-flowering stage (0.5 ± 0.021%). The highest EO yield was observed in August during the full-flowering stage of A. biennis, consistent with findings from Verdian-Rizi et al. (19) regarding Artemisia annua EO yields. Similar results were also reported by Mallavarapu et al. (20), Sellami et al. (21), Özgüven et al. (22), and Rohloff et al. (23) at the full emergence of flower heads in Artemisia pallens Wall. ex Besser, Origanum majorana L., Thymus vulgaris L., and peppermint, respectively. The decrease in EO yield during the vegetative phase of the plant is likely due to the partial inactivation of enzymes necessary for the biosynthesis of specific volatile compounds (21). Therefore, the timing of plant harvesting plays a crucial role in achieving maximum EO yield. Various factors such as the season of harvest, soil pH, plant parts used, drying conditions, chemotype, genotype, subspecies, extraction methods, and plant collection location can impact the final EO yield (5). Previous studies have documented EO yields of A. biennis species in the range of 0.3% (10) to 0.42% (9). The EO yields calculated in the current study exceeded those of other A. biennis ecotypes.
Chemical Composition of Artemisia biennis Essential Oils in Various Growth Stages of Harvest and Their Oil Yield
No. | Name | June | July | August | October | Lopes-Lutz et al. (10) | Nematollahi et al. (9) |
---|---|---|---|---|---|---|---|
1 | Hexanal | 0.1 | |||||
2 | Santolina triene | 4.9 | |||||
3 | α-Thujene | t a | |||||
4 | Benzaldehyde | 0.2 | |||||
5 | Sabinene | 0.1 | 0.8 | ||||
6 | α-Pinene | 0.37 | 0.31 | 0.52 | 0.29 | 0.2 | 10.2 |
7 | Camphene | 2.9 | |||||
8 | p-Cymene | 0.14 | 0.8 | ||||
9 | O-Cymene | 0.18 | |||||
10 | 1,8-Cineole | 0.85 | 0.29 | 10.1 | |||
11 | Linalool | 0.19 | 0.27 | 0.18 | |||
12 | β-Pinene | 0.2 | t a | 1 | |||
13 | (E)-α- necrodol | 3.42 | |||||
14 | Yomogi alcohol | 0.13 | 0.13 | ||||
15 | Limonene | 1.69 | 0.2 | ||||
16 | Limona ketone | 0.3 | 0.13 | 0.41 | |||
17 | β-Phellandrene | t a | |||||
18 | Caryophyllene oxide | 0.39 | 0.2 | ||||
19 | Cyclocopacamphenol | 0.85 | |||||
20 | Myrtenol | 0.9 | |||||
21 | Dihydrocarveol | 0.83 | |||||
22 | Photonerol | 6.37 | 3.94 | 6.8 | |||
23 | Terpinene-4-ol | 0.76 | 0.46 | 0.73 | 1.3 | 2.6 | |
24 | Trans-sabinyl acetate | 0.7 | |||||
25 | (E)-β-Farnesene | 14.13 | 6.89 | 11.49 | 16.38 | 40 | 1.8 |
26 | (Z)-α- Farnesene | 1.18 | |||||
27 | Germacrene D | 0.4 | 5.3 | ||||
28 | Cabreuva oxide A | 0.2 | |||||
29 | β-selinene | 1.8 | |||||
30 | α-selinene | 2.9 | |||||
31 | (E)-β-Ionone | 2.26 | 1.41 | 1.34 | 2.4 | ||
32 | α- Calacorene | 1.19 | |||||
33 | β- Maaliene | 1.49 | 0.99 | 0.58 | |||
34 | δ-cadinene | 0.92 | 1.02 | 0.48 | |||
35 | Alloaromadendrene | 0.81 | |||||
36 | (Z)-Nerolidol | 41.69 | 41.72 | 54.4 | 22.62 | ||
37 | (E)-Nerolidol | 0.45 | 0.94 | ||||
38 | β-Humulene | 0.37 | |||||
39 | (Z)-β-Ocimene | 34.7 | |||||
40 | (E)-β-Ocimene | 0.7 | |||||
41 | cis-Sabinene hydrate | 0.2 | 1 | ||||
42 | trans-Sabinene hydrate | 0.4 | 0.6 | ||||
43 | Artemisia alcohol | 0.1 | 1.4 | ||||
44 | Artemisia ketone | 11.4 | |||||
45 | α-campholenal | 0.6 | |||||
46 | Trans-pinocarveol | 3.8 | |||||
47 | Camphor | 24.6 | |||||
48 | Pinocarvone | 3.2 | |||||
49 | Borneol | 2.6 | |||||
50 | Allo-ocimene | 0.8 | |||||
51 | (Z)-Myroxide | 0.1 | |||||
52 | cis-Verbenyl acetate | 0.2 | |||||
53 | Farnesyl acetate | 0.71 | 0.6 | ||||
54 | (Z), (E)-Farnesyl acetate | 0.5 | |||||
55 | α-himachalene | 0.68 | |||||
56 | β-Costol | 0.73 | |||||
57 | γ-costol | 0.76 | |||||
58 | τ-Cadinol | 0.81 | 1.13 | 1.13 | |||
59 | Aromandendrene | 4.68 | 0.21 | ||||
60 | α-Bisabolol | 0.57 | 1.96 | 1.01 | |||
61 | β- bisabolene | 0.91 | |||||
62 | (Z), (Z)- Farnesol | 0.38 | 9.77 | ||||
63 | (E), (E)-Farnesol | 0.9 | |||||
64 | Valerenol | 0.56 | |||||
65 | β-Cyperone | 0.34 | 0.49 | 0.59 | |||
66 | Viridiflorol | 6.05 | |||||
67 | α-Terpineol | 2.12 | 1.28 | 0.55 | 1.51 | 0.2 | |
68 | α-Terpinene | 0.1 | |||||
69 | γ-Terpinene | 0.33 | 0.8 | 0.5 | |||
70 | (Z)-Caryophyllene | 0.33 | |||||
71 | (E)-Caryophyllene | 0.41 | 1.24 | 0.6 | 1.6 | ||
72 | γ-Gurjunene | 6.52 | |||||
73 | Hexahydrofarnesyl acetone | 0.35 | 1.11 | 0.76 | |||
74 | α- Santalol | 1.70 | 0.3 | ||||
75 | Intermedeol | 6 | |||||
76 | (Z)-tonghaosu | 12.33 | 18.61 | 16.38 | 17.06 | 10 | |
77 | (E)- tonghaosu | 1 | |||||
78 | Tibetin spiroether | 0.37 | 0.41 | 0.55 | 0.68 | ||
79 | Gerany-p-cymene | 0.4 | 0.88 | 1.01 | 0.81 | ||
80 | Phytol | 0.18 | 0.46 | 0.59 | |||
81 | Ascaridole | 1.5 | |||||
82 | Valencene | 0.81 | |||||
83 | Lyratyl acetate | 0.54 | |||||
84 | Monoterpene hydrocarbon | 2.59 | 0.45 | 0.52 | 0.47 | ||
85 | Oxygenated monoterpenes | 11.24 | 6.93 | 3.97 | 11.55 | ||
86 | Sesquiterpene hydrocarbon | 23.08 | 11.89 | 11.49 | 26.34 | ||
87 | Oxygenated sesquiterpenes | 48.71 | 56.99 | 63.44 | 41.15 | ||
88 | diterpene hydrocarbon | 0.4 | 0.88 | 1.01 | 0.81 | ||
89 | Oxygenated diterpene | 0.18 | 0.46 | 0.59 | |||
90 | Spiro Ethers | 12.7 | 19.02 | 16.93 | 17.74 | ||
91 | Unknown | 1.1 | 3.38 | 2.64 | 1.35 | ||
92 | Oil yield (%w/w) | 0.36 ± 0.02 | 0.4 ± 0.014 | 0.5 ± 0.021 | 0.29 ± 0.08 | 0.3 | 0.42 |
The GC/MS chromatograms of A. biennis EO are presented in Figure 1, with the corresponding results detailed in Table 1. The chemical constituents across four different collection periods (based on month) were identified, accounting for at least 96% of the total composition. The results showed that 35, 30, 11, and 32 total compounds were identified in June, July, August, and October, respectively. The chemical components of the analyzed oils were classified into monoterpene hydrocarbons, oxygenated monoterpenes, sesquiterpene hydrocarbons, oxygenated sesquiterpenes, diterpene hydrocarbons, oxygenated diterpenes, and spiroethers. Qualitative and quantitative variations in the chemical profile of the EOs were observed across different harvesting months. Overall, the chemical composition was dominated by oxygenated sesquiterpenes, with oxygenated diterpenes being a minor fraction.
The GC/MS chromatogram of the Artemisia biennis essential oil (EO) under various growth stages during June, July, August, and October
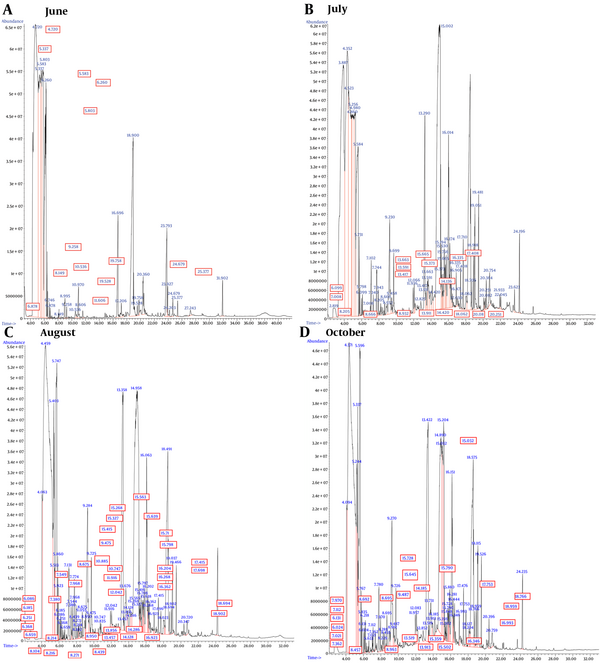
The chemical diversity of the EO constituents decreased as the growth stages progressed towards the full-flowering stage, while the yield of volatile oil extraction increased inversely. Additionally, during the first three stages of plant growth, the number of distinctive and exclusive compounds—those detected in only one growth stage—also decreased. However, in the late vegetative stage, all three trends were reversed, resulting in an increase in the chemical diversity of the EO constituents, a decrease in the yield of volatile oil extraction, and an increase in the number of distinctive and exclusive components.
In all EO samples, oxygenated monoterpenes and oxygenated sesquiterpenes were present in higher concentrations than their hydrocarbon counterparts. The highest level of monoterpenes was recorded in June, contrasting with the lowest amount observed in August. Conversely, the highest level of sesquiterpenes was recorded in August, while the lowest amount was observed in October. The content of oxygenated monoterpenes and sesquiterpenes in October and August (11.55% and 63.44%, respectively) was higher than in any other collection months (Table 1). It's important to note that oxygenated terpenoids, known for their contributions to fragrance and therapeutic properties, are considered essential markers for assessing the quality of EOs (24).
Throughout the four different harvesting times, the major constituents of A. biennis EO were (Z)-nerolidol, (E)-β-farnesene, and (Z)-tonghaosu (Figure 2). However, there were variations in the percentages of these constituents at each month. The percentage of (Z)-nerolidol showed only a slight increase from June to July (41.69% and 41.72%, respectively). In August, the proportion of (Z)-nerolidol significantly increased to 54.4%, before dropping to its lowest level in October (22.62%). The level of (E)-β-farnesene was recorded at 14.13% during the early vegetative stage, experienced a distinct decrease in July (6.89%), and then increased again during the full-flowering and late vegetative phases (11.49% and 16.38%, respectively). (Z)-tonghaosu exhibited its lowest value during the early vegetative stage (12.33%), followed by a rapid increase during the pre-flowering stage (18.61%), reaching its highest proportion compared to other growth stages.
Major chemical compounds identified in the essential oil (EO) of Artemisia biennis
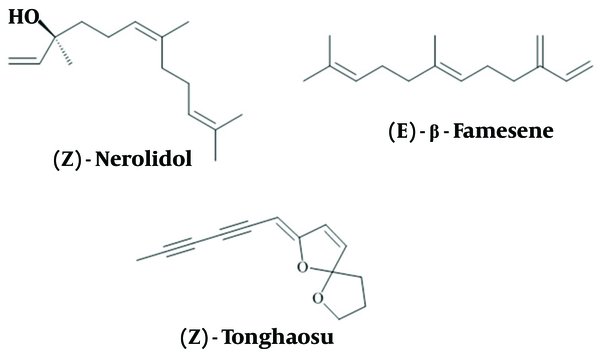
Previous research by Lopes-Lutz et al. (10) identified (E)-β-farnesene and (Z)-β-ocimene as the major components in A. biennis EO. Another study by Nematollahi et al. (9) found camphor (24.6%), Artemisia ketone (11.4%), and α-pinene (10.2%) as the main components of A. biennis EOs. The diversity in extracted secondary metabolites can be attributed to various environmental factors such as the collection season, plant age, environmental conditions, and genetic factors (25, 26).
Investigating the effects of four different harvest times allowed for the first-time study of EO changes in A. biennis in Iran. This study revealed a distinctive and exclusive array of compounds in A. biennis EO compared to previous research (9, 10). The majority of exclusive compounds in A. biennis EO were attributed to the June and October harvests. Seven compounds were unique to June, including dihydrocarveol (0.83%), cabreuva oxide A (0.20%), alloaromadendrene (0.81%), β-humulene (0.37%), α-himachalene (0.68%), valerenol (0.56%), and lyratyl acetate (0.54%). Similarly, seven distinctive compounds were identified in the October harvest: O-cymene (0.18%), β-costol (0.73%), (E, E)-farnesol (0.9%), (Z)-caryophyllene (0.33%), γ-gurjunene (6.52%), ascaridole (1.50%), and valencene (0.81%).
The EO harvested in July contained six distinctive compounds, accounting for 10.94% of the total composition. These included cyclocopacamphenol (0.85%), (Z)-α-farnesene (1.18%), α-calacorene (1.19%), γ-costol (0.76%), β-bisabolene (0.91%), and viridiflorol (6.05%). During the full-flowering stage, which had the highest EO yield, two exclusive components with relatively high proportions were identified: E-α-necrodol (3.42%) and intermedeol (6.00%).
Nerolidol, which was found in the highest proportion in the EOs of the current study, is a sesquiterpene alcohol characterized by a floral odor and is a prominent component extracted from various medicinal plants. The wide-ranging pharmacological activities of nerolidol have attracted significant research interest, including its reported efficacy in anticancer and antitumor effects (27, 28), anti-inflammatory properties (29, 30), antiulcer activity (31), antimalarial activity (32), antifungal and antibacterial activity (33-36), anti-biofilm properties (37), antioxidant benefits (38), anti-parasitic effects (39, 40), and its role as a skin-penetration enhancer (41).
(E)-β-farnesene, a linear sesquiterpenoid, is another important component of the plant's essential oil. Farnesene is known for a wide range of biological effects, including antioxidant (42), antibacterial (43), and antifungal properties (44, 45). Additionally, farnesene has been employed as an intermediary in the synthesis of isophytol, the precursor of vitamin E (46).
4.2. Cytotoxicity Results
Colorectal cancer is the third most common cancer worldwide, ranking third among men and second among women. Additionally, breast cancer is the most common cancer in women in 157 out of 185 countries as of 2022. Notably, recent studies have shown that female breast cancer has surpassed lung cancer as the most commonly diagnosed cancer, with an estimated 2.3 million new cases (11.7%), followed by lung (11.4%) and colorectal (10.0%) cancers (47). Given this context, the cytotoxic effect of A. biennis EOs on breast and colon human cancer cell lines was investigated.
In a study conducted by Tayarani-Najaran et al. (48), the cytotoxic and apoptotic effects of the dichloromethane extract of A. biennis on the K562 and HL-60 cancer cell lines were examined. The A. biennis extract was found to induce apoptosis in human leukemia cells through a mitochondria- and caspase-dependent pathway.
The percentage of cell viability after 24 hours of exposure to different concentrations of the EOs (0, 6.25, 12.5, 25, 50, and 100 μg/mL) was evaluated using the MTT method, and the results were analyzed with Prism software (Figure 3). The cytotoxicity effects on cell lines were found to be dose-dependent. According to Table 2, the most significant reduction in cell viability across all concentrations was observed with the EO extracted in June, demonstrating IC50 values of 2.99 ± 0.23, 2.41 ± 0.2, and 2.84 ± 0.15 μg/mL against normal fibroblast, HT-29, and MCF-7 cells, respectively (*P < 0.05).
The growth inhibition activity of various concentrations of Artemisia biennis essential oils (EOs) on human normal fibroblast, HT29, and MCF-7 cancer cell lines. Values were mean ± SEM of at least three independent experiments (n = 3). * P < 0.05, ** P < 0.01, and *** P < 0.001 compared to the control group.
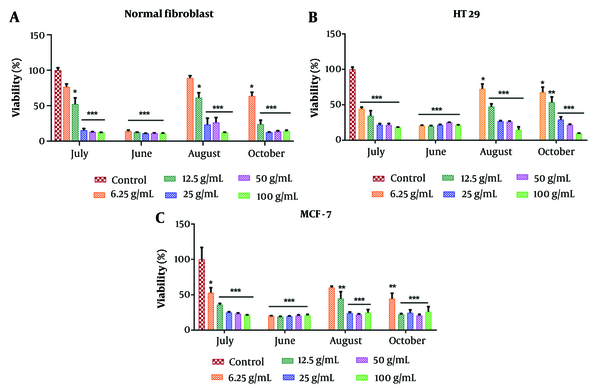
The Calculated IC50 (µg/mL) of Artemisia biennis Essential Oils Was Collected in Four Months on Normal Fibroblast, HT29 and MCF7 Cells. Data Are Presented as Mean ± SEM
Variables | June | July | August | October |
---|---|---|---|---|
Normal fibroblast | 2.99 ± 0.23 | 12.21 ± 0.38 | 14.96 ± 0.51 | 7.81 ± 0.36 |
HT-29 | 2.41 ± 0.2 | 5.64 ± 0.57 | 11.46 ± 0.32 | 14.12 ± 0.81 |
MCF-7 | 2.84 ± 0.15 | 7.66 ± 0.42 | 10.5 ± 0.41 | 5.5 ± 0.28 |
Generally, the compounds obtained from EOs in June and August had the highest and lowest proportions of monoterpenes, respectively. These phytochemicals have been extensively studied for their potential as anticancer agents in various tumor cell lines (49, 50), primarily by triggering apoptosis through intrinsic mechanisms, which result from oxidative stress induced by elevated ROS levels (51). In addition, monoterpenes have demonstrated cytostatic effects by blocking the cell cycle and inhibiting cell invasion and migration (51).
There are numerous reports on the cytotoxic effects of sesquiterpenes. For example, nerolidol—the main component of the EOs in the current study—suppresses the growth of bone cancer cells (MG-63) and induces apoptosis via PI3K/JNK regulation through cell cycle arrest (52). Many studies have investigated the anticancer potential of nerolidol (53, 54), particularly its effects on inhibiting cell growth and reducing cell proliferation. Lu et al. (55) suggested that nerolidol suppressed the growth of Glioblastoma multiforme cells and triggered cell death by modulating the activities of cell-cycle proteins through the p38 mitogen-activated protein kinase signaling pathways.
Additionally, (Z)-β-farnesene has shown significant anticancer potential, as reported by Afoulous et al. (56). Shaaban et al. (57) demonstrated that Matricaria chamomilla extracts could inhibit Caco-2 colon cancer cell migration, with tonghaosu identified as a key component responsible for this activity.
4.3. Conclusions
This study provided a quantitative and qualitative investigation of A. biennis EOs across different growth stages. Regardless of the growth stage, (Z)-nerolidol, (E)-β-farnesene, and (Z)-tonghaosu were identified as the major components of the EOs. The EO yields increased as the plant progressed to the full-flowering stage, with a subsequent decrease in the late vegetative stage. Based on the MTT assay results, the EO extracted during the early vegetative stage exhibited more potent cytotoxic activity against MCF-7 and HT-29 cells. The IC50 values for the normal human fibroblast and MCF-7 cell lines increased as the plant advanced to the full-flowering stage, but this trend reversed in the late vegetative stage. Interestingly, the content of oxygenated sesquiterpenes followed the same rise-and-fall pattern as the cytotoxic activity, while the trend for oxygenated monoterpenes exhibited the opposite pattern.
References
-
1.
Bora KS, Sharma A. The genus Artemisia: a comprehensive review. Pharm Biol. 2011;49(1):101-9. [PubMed ID: 20681755]. https://doi.org/10.3109/13880209.2010.497815.
-
2.
Hatami T, Emami SA, Miraghaee SS, Mojarrab M. Total Phenolic Contents and Antioxidant Activities of Different Extracts and Fractions from the Aerial Parts of Artemisia biennis Willd. Iran J Pharm Res. 2014;13(2):551-9. [PubMed ID: 25237350]. [PubMed Central ID: PMC4157030].
-
3.
Tan RX, Tang HQ, Hu J, Shuai B. Lignans and sesquiterpene lactones from Artemisia sieversiana and Inula racemosa. Phytochemistry. 1998;49(1):157-61. [PubMed ID: 9745767]. https://doi.org/10.1016/s0031-9422(97)00889-3.
-
4.
Tayarani-Najaran Z, Akaberi M, Hassanzadeh B, Shirazi N, Asili J, Al-Najjar H, et al. Analysis of the Essential Oils of Five Artemisia Species and Evaluation of their Cytotoxic and Proapoptotic Effects. Mini Rev Med Chem. 2019;19(11):902-12. [PubMed ID: 30864526]. https://doi.org/10.2174/1389557519666190311155021.
-
5.
Abad MJ, Bedoya LM, Apaza L, Bermejo P. The artemisia L. Genus: a review of bioactive essential oils. Molecules. 2012;17(3):2542-66. [PubMed ID: 22388966]. [PubMed Central ID: PMC6268508]. https://doi.org/10.3390/molecules17032542.
-
6.
Tilaoui M, Ait Mouse H, Jaafari A, Zyad A. Comparative Phytochemical Analysis of Essential Oils from Different Biological Parts of Artemisia herba alba and Their Cytotoxic Effect on Cancer Cells. PLoS One. 2015;10(7). e0131799. [PubMed ID: 26196123]. [PubMed Central ID: PMC4510584]. https://doi.org/10.1371/journal.pone.0131799.
-
7.
Ramazani E, Tayarani-Najaran Z, Shokoohinia Y, Mojarrab M. Comparison of the cytotoxic effects of different fractions of Artemisia ciniformis and Artemisia biennis on B16/F10, PC3 and MCF7 Cells. Res Pharm Sci. 2020;15(3):273-80. [PubMed ID: 33088327]. [PubMed Central ID: PMC7540818]. https://doi.org/10.4103/1735-5362.288434.
-
8.
V. Mozaffarian. A Dictionary of Iranian Plant Names. Tehran: Farhang Mo'aser; 2009.
-
9.
Nematollahi F, Rustaiyan A, Larijani K, Nadimi M, Masoudi S. [Essential Oil Composition of Artemisia bienniszWilld and Pulicaria undulata(L.) C.A. Mey. Two Compositae Herbs Growing Wild in Iran]. J Essent Oil Res. 2006;18(3):339-41. FA. https://doi.org/10.1080/10412905.2006.9699106.
-
10.
Lopes-Lutz D, Alviano DS, Alviano CS, Kolodziejczyk PP. Screening of chemical composition, antimicrobial and antioxidant activities of Artemisia essential oils. Phytochemistry. 2008;69(8):1732-8. [PubMed ID: 18417176]. https://doi.org/10.1016/j.phytochem.2008.02.014.
-
11.
Hedayati Rad F, Sharifan A, Khodaiyan F, Shahabi Ghahfarrokhi I. Preparation and characterization of pullulan-soy protein concentrate blended film incorporated with Zataria multiflora and Artemisia biennis essential oils. Jundishapur J Nat Pharm Prod. 2017;12(4).
-
12.
Zarei M, Ahmadimoghaddam D, Mohammadi S. Artemisia biennis Willd.: Anti-Nociceptive effects and possible mechanisms of action. J Ethnopharmacol. 2021;268:113604. [PubMed ID: 33232780]. https://doi.org/10.1016/j.jep.2020.113604.
-
13.
Mohammadi A, Sani TA, Ameri AA, Imani M, Golmakani E, Kamali H. Seasonal variation in the chemical composition, antioxidant activity, and total phenolic content of Artemisia absinthium essential oils. Pharmacognosy Res. 2014;7(4):329-34. [PubMed ID: 26692746]. [PubMed Central ID: PMC4660511]. https://doi.org/10.4103/0974-8490.158441.
-
14.
Guenther E, Kulka K, Rogers JA. Essential Oils. Anal Chem J. 2002;31(4):679-87. https://doi.org/10.1021/ac60148a005.
-
15.
Daferera DJ, Ziogas BN, Polissiou MG. GC-MS analysis of essential oils from some Greek aromatic plants and their fungitoxicity on Penicillium digitatum. J Agric Food Chem. 2000;48(6):2576-81. [PubMed ID: 10888587]. https://doi.org/10.1021/jf990835x.
-
16.
Adams RP. Identification of Essential Oil Components By Gas Chromatography/Mass Spectrometry. Allured publishing corporation Carol Stream; 2007.
-
17.
Plumb JA. Cell sensitivity assays: the MTT assay. Methods Mol Med. 2004;88:165-9. [PubMed ID: 14634227]. https://doi.org/10.1385/1-59259-406-9:165.
-
18.
Plumb JA. Cell sensitivity assays : the MTT assay. Methods Mol Med. 1999;28:25-30. [PubMed ID: 21374024]. https://doi.org/10.1385/1-59259-687-8:25.
-
19.
Verdian-Rizi M. Variation in the essential oil composition of Artemisia annua L. of different growth stages cultivated in Iran. Afr J Plant Sci. 2008;2(2).
-
20.
Mallavarapu GR, Kulkarni RN, Baskaran K, Rao L, Ramesh S. Influence of plant growth stage on the essential oil content and composition in Davana (Artemisia pallens wall.). J Agric Food Chem. 1999;47(1):254-8. [PubMed ID: 10563881]. https://doi.org/10.1021/jf980624c.
-
21.
Sellami IH, Maamouri E, Chahed T, Wannes WA, Kchouk ME, Marzouk B. Effect of growth stage on the content and composition of the essential oil and phenolic fraction of sweet marjoram (Origanum majorana L.). Ind Crops Prod. 2009;30(3):395-402. https://doi.org/10.1016/j.indcrop.2009.07.010.
-
22.
Özgüven M, Tansi S. Drug yield and essential oil of Thymus vulgaris L. as in influenced by ecological and ontogenetical variation. Turk J Agric For. 1998;22(6):537-42.
-
23.
Rohloff J, Dragland S, Mordal R, Iversen TH. Effect of harvest time and drying method on biomass production, essential oil yield, and quality of peppermint (Mentha x piperita L.). J Agric Food Chem. 2005;53(10):4143-8. [PubMed ID: 15884852]. https://doi.org/10.1021/jf047998s.
-
24.
Elyemni M, Louaste B, Nechad I, Elkamli T, Bouia A, Taleb M, et al. Extraction of Essential Oils of Rosmarinus officinalis L. by Two Different Methods: Hydrodistillation and Microwave Assisted Hydrodistillation. ScientificWorldJournal. 2019;2019:3659432. [PubMed ID: 31057339]. [PubMed Central ID: PMC6463580]. https://doi.org/10.1155/2019/3659432.
-
25.
Yang L, Wen KS, Ruan X, Zhao YX, Wei F, Wang Q. Response of Plant Secondary Metabolites to Environmental Factors. Molecules. 2018;23(4). [PubMed ID: 29584636]. [PubMed Central ID: PMC6017249]. https://doi.org/10.3390/molecules23040762.
-
26.
Li Y, Kong D, Fu Y, Sussman MR, Wu H. The effect of developmental and environmental factors on secondary metabolites in medicinal plants. Plant Physiol Biochem. 2020;148:80-9. [PubMed ID: 31951944]. https://doi.org/10.1016/j.plaphy.2020.01.006.
-
27.
Chan CK, Supriady H, Goh BH, Kadir HA. Elephantopus scaber induces apoptosis through ROS-dependent mitochondrial signaling pathway in HCT116 human colorectal carcinoma cells. J Ethnopharmacol. 2015;168:291-304. [PubMed ID: 25861953]. https://doi.org/10.1016/j.jep.2015.03.072.
-
28.
Gautam N, Mantha AK, Mittal S. Essential oils and their constituents as anticancer agents: a mechanistic view. Biomed Res Int. 2014;2014:154106. [PubMed ID: 25003106]. [PubMed Central ID: PMC4070586]. https://doi.org/10.1155/2014/154106.
-
29.
Lima DK, Ballico LJ, Rocha Lapa F, Goncalves HP, de Souza LM, Iacomini M, et al. Evaluation of the antinociceptive, anti-inflammatory and gastric antiulcer activities of the essential oil from Piper aleyreanum C.DC in rodents. J Ethnopharmacol. 2012;142(1):274-82. [PubMed ID: 22588049]. https://doi.org/10.1016/j.jep.2012.05.016.
-
30.
Fonseca DV, Salgado PR, de Carvalho FL, Salvadori MG, Penha AR, Leite FC, et al. Nerolidol exhibits antinociceptive and anti-inflammatory activity: involvement of the GABAergic system and proinflammatory cytokines. Fundam Clin Pharmacol. 2016;30(1):14-22. [PubMed ID: 26791997]. https://doi.org/10.1111/fcp.12166.
-
31.
Klopell FC, Lemos M, Sousa JP, Comunello E, Maistro EL, Bastos JK, et al. Nerolidol, an antiulcer constituent from the essential oil of Baccharis dracunculifolia DC (Asteraceae). Z Naturforsch C J Biosci. 2007;62(7-8):537-42. [PubMed ID: 17913068]. https://doi.org/10.1515/znc-2007-7-812.
-
32.
Saito AY, Marin Rodriguez AA, Menchaca Vega DS, Sussmann RAC, Kimura EA, Katzin AM. Antimalarial activity of the terpene nerolidol. Int J Antimicrob Agents. 2016;48(6):641-6. [PubMed ID: 27742206]. https://doi.org/10.1016/j.ijantimicag.2016.08.017.
-
33.
Lee SJ, Han JI, Lee GS, Park MJ, Choi IG, Na KJ, et al. Antifungal effect of eugenol and nerolidol against Microsporum gypseum in a guinea pig model. Biol Pharm Bull. 2007;30(1):184-8. [PubMed ID: 17202684]. https://doi.org/10.1248/bpb.30.184.
-
34.
Fonseca Bezerra C, de Alencar Junior JG, de Lima Honorato R, Dos Santos ATL, Pereira da Silva JC, Silva TGD, et al. Antifungal Properties of Nerolidol-Containing Liposomes in Association with Fluconazole. Membranes (Basel). 2020;10(9). [PubMed ID: 32825411]. [PubMed Central ID: PMC7558210]. https://doi.org/10.3390/membranes10090194.
-
35.
Goncalves O, Pereira R, Goncalves F, Mendo S, Coimbra MA, Rocha SM. Evaluation of the mutagenicity of sesquiterpenic compounds and their influence on the susceptibility towards antibiotics of two clinically relevant bacterial strains. Mutat Res. 2011;723(1):18-25. [PubMed ID: 21453784]. https://doi.org/10.1016/j.mrgentox.2011.03.010.
-
36.
de Moura DF, Rocha TA, de Melo Barros D, da Silva MM, Dos Santos Santana M, Neta BM, et al. Evaluation of the antioxidant, antibacterial, and antibiofilm activity of the sesquiterpene nerolidol. Arch Microbiol. 2021;203(7):4303-11. [PubMed ID: 34110480]. https://doi.org/10.1007/s00203-021-02377-5.
-
37.
Curvelo JAR, Marques AM, Barreto ALS, Romanos MTV, Portela MB, Kaplan MAC, et al. A novel nerolidol-rich essential oil from Piper claussenianum modulates Candida albicans biofilm. J Med Microbiol. 2014;63(Pt 5):697-702. [PubMed ID: 24523158]. https://doi.org/10.1099/jmm.0.063834-0.
-
38.
Nogueira Neto JD, de Almeida AA, da Silva Oliveira J, Dos Santos PS, de Sousa DP, de Freitas RM. Antioxidant effects of nerolidol in mice hippocampus after open field test. Neurochem Res. 2013;38(9):1861-70. [PubMed ID: 23765368]. https://doi.org/10.1007/s11064-013-1092-2.
-
39.
Marques AM, Peixoto ACC, de Paula RC, Nascimento MFA, Soares LF, Velozo LSM, et al. Phytochemical Investigation of Anti-plasmodial Metabolites from Brazilian NativePiperSpecies. J Essent Oil Bear Plants. 2015;18(1):74-81. https://doi.org/10.1080/0972060x.2014.974075.
-
40.
Anthony JP, Fyfe L, Smith H. Plant active components - a resource for antiparasitic agents? Trends Parasitol. 2005;21(10):462-8. [PubMed ID: 16099722]. https://doi.org/10.1016/j.pt.2005.08.004.
-
41.
Krishnaiah YS, Al-Saidan SM, Jayaram B. Effect of nerodilol, carvone and anethole on the in vitro transdermal delivery of selegiline hydrochloride. Pharmazie. 2006;61(1):46-53. [PubMed ID: 16454206].
-
42.
Fırat Z, Demirci F, Demirci B. Antioxidant Activity of Chamomile Essential Oil and Main Components. Nat Volatiles Essent Oils. 2018;5(1).
-
43.
Molina-Gallardo AR, Cruz-Martínez YR, Orozco-Martínez J, Valencia Quiroz I, Hernández-Delgado CT. The Roles of Farnesol and Farnesene in Curtailing Antibiotic Resistance. Biotechnology and Drug Development for Targeting Human Diseases. 2024. p. 52-69. https://doi.org/10.2174/9789815223163124090005.
-
44.
ÇElİK K, ToĞAr B, TÜRkez H, TaŞPinar N. In vitro cytotoxic, genotoxic, and oxidative effects of acyclic sesquiterpene farnesene. Turk J Biol. 2014;38:253-9. https://doi.org/10.3906/biy-1309-55.
-
45.
Satyal P, Shrestha S, Setzer WN. Composition and Bioactivities of an (E)-β-Farnesene Chemotype of Chamomile (Matricaria Chamomilla) Essential Oil from Nepal. Nat Prod Commun. 2015;10(8). https://doi.org/10.1177/1934578x1501000835.
-
46.
Liu Y, Wang Z, Cui Z, Qi Q, Hou J. Progress and perspectives for microbial production of farnesene. Bioresour Technol. 2022;347:126682. [PubMed ID: 35007732]. https://doi.org/10.1016/j.biortech.2022.126682.
-
47.
Bray F, Laversanne M, Sung H, Ferlay J, Siegel RL, Soerjomataram I, et al. Global cancer statistics 2022: GLOBOCAN estimates of incidence and mortality worldwide for 36 cancers in 185 countries. CA Cancer J Clin. 2024;74(3):229-63. [PubMed ID: 38572751]. https://doi.org/10.3322/caac.21834.
-
48.
Tayarani-Najaran Z, Makki FS, Alamolhodaei NS, Mojarrab M, Emami SA. Cytotoxic and apoptotic effects of different extracts of Artemisia biennis Willd. on K562 and HL-60 cell lines. Iran J Basic Med Sci. 2017;20(2):166-71. [PubMed ID: 28293393]. [PubMed Central ID: PMC5339657]. https://doi.org/10.22038/ijbms.2017.8242.
-
49.
Greay SJ, Hammer KA. Recent developments in the bioactivity of mono- and diterpenes: anticancer and antimicrobial activity. Phytochem Rev. 2011;14(1):1-6. https://doi.org/10.1007/s11101-011-9212-6.
-
50.
Machado TQ, da Fonseca ACC, Duarte ABS, Robbs BK, de Sousa DP. A Narrative Review of the Antitumor Activity of Monoterpenes from Essential Oils: An Update. Biomed Res Int. 2022;2022:6317201. [PubMed ID: 35655488]. [PubMed Central ID: PMC9155973]. https://doi.org/10.1155/2022/6317201.
-
51.
Silva BIM, Nascimento EA, Silva CJ, Silva TG, Aguiar JS. Anticancer activity of monoterpenes: a systematic review. Mol Biol Rep. 2021;48(7):5775-85. [PubMed ID: 34304392]. https://doi.org/10.1007/s11033-021-06578-5.
-
52.
Yu Y, Velu P, Ma Y, Vijayalakshmi A. Nerolidol induced apoptosis via PI3K/JNK regulation through cell cycle arrest in MG-63 osteosarcoma cells. Environ Toxicol. 2022;37(7):1750-8. [PubMed ID: 35357761]. https://doi.org/10.1002/tox.23522.
-
53.
Biazi BI, Zanetti TA, Baranoski A, Corveloni AC, Mantovani MS. Cis-Nerolidol Induces Endoplasmic Reticulum Stress and Cell Death in Human Hepatocellular Carcinoma Cells through Extensive CYP2C19 and CYP1A2 Oxidation. Basic Clin Pharmacol Toxicol. 2017;121(4):334-41. [PubMed ID: 28256105]. https://doi.org/10.1111/bcpt.12772.
-
54.
Glumac M, Cikes Culic V, Marinovic-Terzic I, Radan M. Mechanism of cis-Nerolidol-Induced Bladder Carcinoma Cell Death. Cancers (Basel). 2023;15(3). [PubMed ID: 36765938]. [PubMed Central ID: PMC9913136]. https://doi.org/10.3390/cancers15030981.
-
55.
Lu Z, Tang T, Huang J, Shi Y. Nerolidol inhibited U-251 human glioblastoma cell proliferation and triggered apoptosis via the upregulation of the p38 MAPK signaling pathway. Adv Clin Exp Med. 2024;33(6):633-40. [PubMed ID: 37747443]. https://doi.org/10.17219/acem/170184.
-
56.
Afoulous S, Ferhout H, Raoelison EG, Valentin A, Moukarzel B, Couderc F, et al. Chemical composition and anticancer, antiinflammatory, antioxidant and antimalarial activities of leaves essential oil of Cedrelopsis grevei. Food Chem Toxicol. 2013;56:352-62. [PubMed ID: 23459148]. https://doi.org/10.1016/j.fct.2013.02.008.
-
57.
Shaaban M, El-Hagrassi AM, Osman AF, Soltan MM. Bioactive compounds from Matricaria chamomilla: structure identification, in vitro antiproliferative, antimigratory, antiangiogenic, and antiadenoviral activities. Z Naturforsch C J Biosci. 2022;77(3-4):85-94. [PubMed ID: 34463438]. https://doi.org/10.1515/znc-2021-0083.