Abstract
Background:
Griseofulvin is an antifungal drug and is available as oral dosage forms. Development of topical treatment could be advantageous for superficial fungal infections of skin. The film forming property of chitosan has made it interesting for transdermal/dermal drug delivery, but showed lack of stability. Soy phosphatidylcholine (PC), besides playing penetration enhancer and solubilizer roles, could enhance the stability and mechanical properties of the film. Films prepared from a mixture of chitosan and PC can achieve the benefits of both materials.Objectives:
The aim of this study was to prepare and characterize films formed from blends of chitosan and soy PC for topical delivery of griseofulvin.Materials and Methods:
Topical films composed of chitosan and soy PC were prepared by means of casting and solvent evaporation technique. The properties of the films were characterized regarding mechanical properties, swelling, ability to transmit vapor, drug release, thermal behavior and antifungal efficacy against Microsporum gypseum and Epidermophyton floccosum.Results:
Presence of soy PC improved mechanical properties, lowered swelling ratio and increased stability of the films. Solubilizing activity of phospholipid resulted in higher flux of drug release from formulation containing higher amount of soy PC. Antifungal efficacy of formulations was confirmed against two species of dermatophytes in vitro.Conclusions:
This topical composite film had the potential for griseofulvin delivery to superficial fungal infections.Keywords
1. Background
Superficial fungal infection is one of the most common infectious diseases. The main infections are mainly caused by dermatophytes belonging to genera Epidermophyton, Microsporum and Trichophyton. Several antifungal drugs are reported as an active agent against dermatophytes. Griseofulvin is one of these drugs and is available as oral dosage forms. Since dermatophytes mostly infect superficial layers of skin, topical treatment provides an alternative route that may bypass undesirable effects of oral administration and increase patient compliance (1).
Chitosan; β (1,4) 2-amino-2-D-glucose is a natural biodegradable and biocompatible polymer produced by alkaline N-deacetylation of chitin. Chitosan has been extensively evaluated as a drug delivery system in different forms of particles, gels and films. The film forming property of chitosan has made it interesting for transdermal/dermal drug delivery (2). Due to the hydrophilic nature of chitosan, films composed of chitosan alone showed lack of stability, chemical cross linking with agents such as glutaraldehyde could overcome this, but they have been found to be toxic and the films are often non-biodegradable (3). Blending chitosan with other materials to produce stable films by physical cross-linking achieved via electrostatic and/or hydrogen bonding interaction has been investigated widely (3-10).
Phosphatidylcholine (PC) of both human and vegetable origin shows a fatty acid composition, which is dominated by unsaturated fatty acids; oleic acid and linoleic acid. Soy PC, which is readily available and mostly used in cosmeceuticals, could fluidize the lipid bilayers of the horny layer and enhance the penetration of active agents. PC is also known as a solubilizer (11). It could enhance the stability and mechanical properties of the film by applying more flexibility to the film. Films prepared from a mixture of chitosan and PC can achieve the benefits of both materials (3).
2. Objectives
The aim of this study was to prepare and characterize films formed from blends of chitosan and soy PC for topical delivery of griseofulvin.
3. Materials and Methods
3.1. Materials
Griseofulvin supplied by Hengo, China, Chitosan (Viscosity 200-600 cp, Deacetylation degree 92%) purchased from Primex, Iceland and soy phosphatidylcholine (phospholipon 85G®) supplied by Lipoid (Germany) were used for the preparation of formulations. Sabouraud dextrose agar medium supplemented with 0.05% cycloheximide and 0.005% chloramphenicol (SCC) purchased from Merck (Germany). All other materials and reagents were of the highest grade commercially available. Microsporum gypseum and Epidermophyton floccosum were obtained from Iran Zamin laboratory, Ahvaz, Iran.
3.2. Preparation of the Films
The films were prepared using casting and solvent evaporation. Chitosan solution (Cs) (2% w/v) was prepared by dispersing required amount of chitosan in acetic acid solution (1% v/v). Soy PC solution (1% and 2% w/v) in ethanol and propylene glycol (5:1 v/v) containing certain amount of griseofulvin at final concentration of 0.2% w/v was mixed with the same volume of chitosan solution, vortexed and sonicated. Twenty-four milliliters of the mixture was placed in a glass Petri dish (9 cm diameter) and dried at 45°C for 48 hours and then peeled off (10, 12). These formulations were named PC1Cs2 and PC2Cs2, respectively. A film formulation without soy PC (NPC/Cs2) also prepared as control.
3.3. Characterization of the Films
3.3.1. Film Thickness
The thickness of both films was measured at five different spots using micrometer and mean values were calculated.
3.3.2. Mechanical Properties
Film strips (20 × 20 mm) were fixed between the two clamps of a texture analyzer (WDW-5, China) with a 50 N load cell. The strips were pulled at the rate of 5 mm/min; tensile strength (TS) and elongation at break (EB) were calculated as follows:


3.3.3. Water Vapor Transmission Rate (WVTR)
Three cylindrical glass containers of the same type and size were used. The containers were filled up with 5 g of CaCl2 and the opening was covered with the films. The area available for vapor permeation was 0.257 cm2. All the containers were maintained at 60°C for one day to reach constant weights. Then containers put in wet desiccators (75% relative humidity) and weighted at the days 1, 2, 3, 4 and 5. Changes in the weight of the containers were plotted against time. The slope of the weight vs. time plot was divided by the effective film area to obtain WVTR (g/m2.day) (10, 14).
3.3.4. Swelling and Erosion
A 20 × 20 mm dry film was weighted carefully and immersed in 50 mL of phosphate buffer (pH = 7.4) at 37°C. At predetermined intervals (15, 30, 45, 60, 75, 90, 105, 120, 150, 180, 210, 240 min and 24 hours), the films were removed, the excess water was dried with filter paper and weighed.
Swelling ratio (Q) was calculated using Equation 3:

W2 is the weight of film at each time point and W1 is the weight of initial dry film (3).
Then the samples were desiccated in an oven at 60°C for 24 hours and the weights of dried films (W3) were recorded. Erosion (E) was calculated using Equation 4 (15):

3.3.5. Content Uniformity
1 × 1 cm film was dissolved in 10 mL phosphate buffer (pH = 7.4), methanol (3:1 v/v) and then filtered through membrane filter 0.45 µm. The concentration of griseofulvin was measured at 295 nm by UV spectrophotometer. The test was repeated five times (1, 16).
3.4. In vitro Drug Release
Drug release studies were performed in vitro using Jacketed Franz cells with a receiver volume of 28 mL at 37°C. Phosphate buffer (pH = 7.4): methanol (3:1 v/v) was used as the receiver medium. A suitable size of the films was mounted in the Franz cell. Two milliliters of the receiver medium was withdrawn at 0.5, 1, 2, 3, 4, 5, 6, 7 and 8 hours and replaced with the same volume of blank receiver medium solution. Aliquots of the collected samples were analyzed for their griseofulvin content at 295 nm by UV spectrophotometer. The derived concentration values were corrected using Equation 5:

Where Mt(n) is the current cumulative mass of drug transported at time t, n is the number (times) of sampling, Cn is the current concentration in the receiver medium, ΣCm is the total of previously measured concentrations, Vr is the volume of receiver medium and Vs corresponds to the volume of sample removed for analysis (17).
The steady-state flux (Jss) of formulations was determined by the slope of the linear portion of plots of the amount of drug in the receiving chamber versus time, divided by exposed surface area of the film and lag time was estimated from the x-intercept of the linear portion of the graph (18).
3.5. Differential Scanning Calorimetry (DSC)
DSC thermograms were performed on drug alone, blank and drug loaded NPC/Cs2, PC1Cs2 and PC2Cs2. The procedure involved heating an accurately weighed sample in an aluminum pan at scanning rate of 10°C/min, over temperature range of 0°C to 300°C.
3.6. In vitro Antifungal Effect
Microsporum gypseum and Epidermophyton floccosum were cultivated for seven days at 28°C on SCC medium. Inoculums were prepared by pouring 9 mL of sterile normal saline containing 0.05% tween 80 on the agar plate surface, followed by a gentle scraping and then the suspensions were transferred into sterile tubes. A suspension equal to the 0.5 McFarland (106 colony forming units (CFU)/mL) was prepared by dilution in a sterile saline solution and diluted more to 104 CFU/mL. One-hundred microliters of this suspension (104 CFU/mL) was spread on the plates and allowed to dry at room temperature for 15 minutes. 7 mm discs of film formulations, blank film and paper disc containing 0.2% griseofulvin were put on the surface of the plates. After incubation for nine days at 28°C, inhibition zone diameters formed around the discs were measured (19).
3.7. Statistical Analysis
All experiments were performed in triplicate and expressed as mean ± SD unless otherwise stated. T-test or One-way ANOVA statistical test and multiple comparison Tukey test were used to assess the differences among the various groups. P < 0.05 was considered statistically significant.
4. Results
4.1. Characterization of the Films
Films formed from chitosan and soy PC were easily removed from the Petri dishes and showed acceptable color and appearance. NPC/Cs2 was somehow brittle and it was difficult to peel it off intact. Besides, crystals of drug were visible on the surface of the film.
4.1.1. Film Thickness and Mechanical Properties
The thickness, TS and EB of the films are shown in Table 1. The presence of soy PC increased film thickness (P < 0.05). There was no statistical significant differences between mechanical properties of NPC/Cs2 and PC2Cs2 (P > 0.05), but PC1Cs2 showed higher TS and EB than the two other formulations (P < 0.05).
Formulation | Thickness, mm (n = 5) | TS, N/mm2 (n = 3) | EB, % (n = 3) |
---|---|---|---|
NPC/Cs2 | 0.085 ± 0.004 | 20.193 ± 1.448 | 20.193 ± 1.448 |
PC1Cs2 | 0.184 ± 0.015 | 41.370 ± 2.238 | 41.370 ± 2.238 |
PC2Cs2 | 0.242 ± 0.013 | 27.890 ± 5.125 | 51.213 ± 5.541 |
4.1.2. Water Vapor Transmission Rate (WVTR)
WVTR from PC1Cs2 and PC2Cs2 were 2516.21 ± 383.88 and 2671.85 ± 227.99 (g/m2.day), respectively. These values did not show any significant difference (P > 0.05).
4.1.3. Swelling Ratio (Q) and Erosion
The swelling ratio of films in pH 7.4 phosphate buffer is shown in Figure 1. All films swelled rapidly in 15 minutes. ANOVA analysis showed that at points 15, 30 and 45 minutes, the greater SPC ratio added into the films, the lower the Q was (P < 0.05), but there was no significant difference between NPC/Cs2 and PC1Cs2 at time 1 hour and later.
Swelling Ratio of the Films in Phosphate Buffer (pH = 7.4) at 37°C.
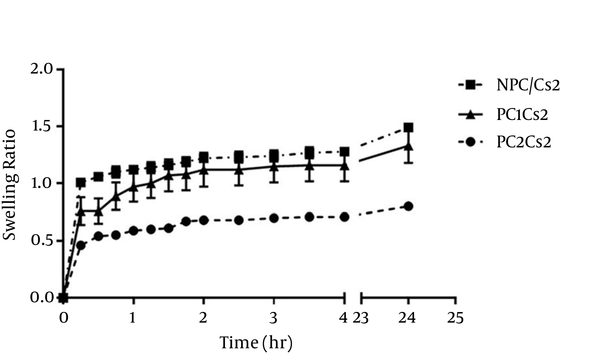
SPC containing films kept up the original shape and weight after 24 hours, but the erosion percent of NPC/Cs2 was 36.63% ± 0.65.
4.1.4. Content Uniformity
Film formulations containing SPC were found to be of uniform drug content. Table 2 shows the ratio of content of griseofulvin per 1 cm2 film to theoretical amount of loaded drug (drug content) and variation in distribution of griseofulvin in different film regions relative to the average (RSD%). This test was not performed for NPC/Cs2 because of visibility of drug crystals on the surface of the film showing lack of incorporation of the drug in the film.
Formulation | Drug Content ,% | RSD ,% |
---|---|---|
PC1Cs2 | 97.76 ± 3.68 | 3.77 |
PC2Cs2 | 95.66 ± 6.77 | 7.08 |
4.2. In vitro Drug Release
The release profiles of griseofulvin from film formulations are shown in Figure 2. Jss of PC1Cs2 and PC2Cs2 were 0.030 ± 0.004 and 0.041 ± 0.003 mg/cm2.h, respectively. A lag time of about 90 minutes was obtained for PC2Cs2, whereas griseofulvin released from PC1Cs2 without any lag time.
Release Profiles of Griseofulvin From Film Formulations
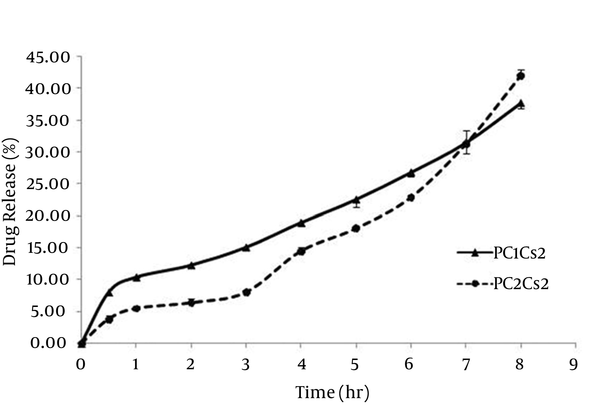
4.3. Differential Scanning Calorimetry (DSC)
Figure 3 depicts the DSC curve of griseofulvin and shows a single melting endothermic peak, melting point at 220°C. Chitosan free film shows a broad peak around 70°C, which can be contributed to non-bound water evaporation. This peak moved to higher temperature by increasing the amount of soy PC in blank films supposedly because of hydrophobicity. Another peak around 150 - 170°C was observed that may be the result of evaporation of bounded water. DSC scans of NPC/Cs2 and PC1Cs2 showed a peak nearly the same as the peak of the drug, but PC2Cs2 showed no such characteristic (2).
DSC thermograms of a) griseofulvin, b) NPC/Cs2, c) PC1Cs2, d) PC2Cs2, e) Blank NPC/Cs2, f) Blank PC1Cs2 and g) Blank PC2Cs2
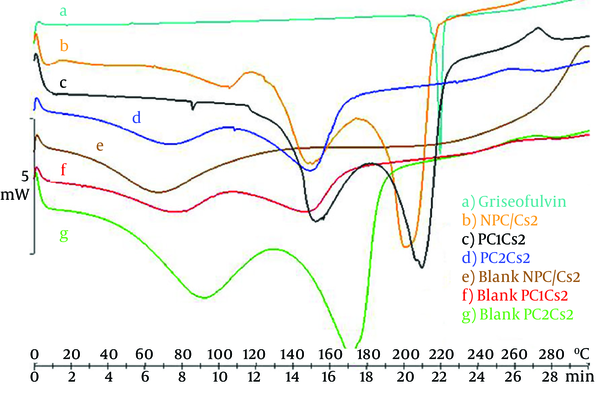
4.4. In vitro Antifungal Effect
The capabilities of film formulations, blank films and solution form of griseofulvin in inhibiting the growth of M. gypseum and E. floccosum on SCC medium are shown in Table 3. The inhibition zone of the formulations against M. gypseum in the decreasing order is: griseofulvin solution > PC2Cs2 > PC1Cs2 > Blank PC2Cs2 > Blank PC1Cs2 (P < 0.05). In case of E. floccosum, the same results were obtained except there was no significant difference between blank formulations (P > 0.05).
Inhibition Zones (mm) of Film Formulations, Blank Films and Solution Form of Griseofulvin against M. gypseum and E. floccosum (n = 4) a,b,c
PC1Cs2 | PC2Cs2 | Blank PC1Cs2 | Blank PC2Cs2 | Griseofulvin Solution | |
---|---|---|---|---|---|
M. gypseum | 14.50 ± 0.58 | 16.75 ± 0.50 | 7.00 ± 0.00 | 9.50 ± 0.58 | 20.25 ± 0.50 |
E. floccosum | 14.50 ± 0.58 | 16.75 ± 0.50 | 8.25 ± 0.50 | 8.75 ± 0.96 | 20.50 ± 1.00 |
5. Discussion
Griseofulvin is a hydrophobic antifungal drug and is currently used orally. Systemic griseofulvin may cause headache, fatigue, nausea and vomiting, diarrhea and even angioedema. Targeting the drug at the site of infection may reduce the duration of treatment and increase patient compliance (20, 21). The film-forming property of chitosan finds many applications including food packaging, wound dressings, sutures, artificial skin, tissue engineering and drug delivery systems, especially in topical formulations. Adequate mechanical strength and flexibility are generally required for these formulations (22, 23). In the past, chemical cross-linking was mostly used to prepare chitosan-based films. However, chemically cross-linked films are not suitable for biomedical applications because of toxicity and being non-biodegradable (3). Blend of chitosan and soy phosphatidylcholine was used in this study to prepare a film formulation for delivery of griseofulvin topically.
The thickness of the films was significantly affected by the percent of PC in the films. PC2Cs2 had more thickness versus PC1Cs2 and PC1Cs2 was thicker than NPC/Cs2. However, TS and EB of PC1Cs2 were higher than the two other formulations. Ionic and hydrogen bonding interactions of chitosan and PC may be explained by the high tensile strength and elongation of PC1Cs2 compared to NPC/Cs2 and since chitosan provides film forming property in these formulations, the higher strength and elasticity of PC1Cs2 compared to PC2Cs2 could be because of higher ratio of chitosan to PC in this formulation. Presence of drug crystals may be the reason for brittleness and lower TS and EB of NPC/Cs2 as well (3, 10, 24).
Water vapor transmission rate is defined as the ease of moisture for penetrating and passing through the hydrophilic portion of film (25). Existence of hydroxyl and amino groups in chitosan generates polarity and it could be the reason for high WVTR of film formulations (26). Queen et al. suggested that a level of 2000 - 2500 g/m2.day is sufficient to give adequate moisture and preventing dehydration, which is close to our results (27).
Possession of polymer bearing amine (-NH2) and hydroxyl groups (-OH) increases chitosan affinity to water and hydrogen bond formation with hydrophilic solvents (10). However, incorporation of Soy PC, which is hydrophobic decreased the availability of hydroxyl and amino groups and caused a reduction in swelling ratio particularly when it was added at higher concentrations (28). At the end of the experiment, NPC/Cs2 showed 36.63% erosion, while PC1Cs2 and PC2Cs2 retained their integrity.
Both film formulations were found to be of uniform drug content as seen in the results given in Table 2.
The release pattern of PC1Cs2 and PC2Cs2 showed 37.61% and 41.89% drug release within eight hours of testing period. In the first six hours, PC1Cs2 showed higher drug release compared to PC2Cs2, at 7th hour the amount of drug release was the same for both formulations and at 8th hour, PC2Cs2 released more griseofulvin.
The drug release from complex systems usually consists of different mechanisms, i.e. entry of the release medium into the matrix, swelling, dissolution of the drug in the medium, drug diffusion through the hydrated matrix and film erosion. This matter could explain that multi-phase release profile was obtained for PC2Cs2 (3, 29).
A lag time of about 90 minutes was obtained for PC2Cs2, but PC1Cs2 released drug immediately without any lag time. It can be because of more hydrophilicity of PC1Cs2, fast initial swelling process of the film and presence of unassociated drug in this formulation, which is also observed in DSC diagrams (Figure 3) (3).
Jss of drug release from PC2Cs2 was significantly (P < 0.05) higher than that of PC1Cs2, which can be contributed to solubilizing activity of amphiphilic molecules of phospholipid (30) and so that the lag time in the drug release from PC2Cs2 can be compensated at 8th hour and led to higher drug release compared to PC1Cs2.
Disappearance of the drug peak in DSC thermogram of PC2Cs2 could be related to the incorporation of griseofulvin in film structure (2).
When the formulations were subjected to agar plate diffusion, it was observed that the disc containing griseofulvin showed larger inhibition zone that could be because of direct availability of the drug (31). The zones of inhibition for PC1Cs2 were less compared to PC2Cs2; this could be attributed to the higher flux of griseofulvin from PC2Cs2. The presence of higher amount of soy PC in PC2Cs2 could be considered the reason of this fact as well. The blank PC2Cs2 also showed greater inhibition zone against M. gypseum compared to blank PC1Cs2, which could be due to solubilizing activity of phospholipid (30). Extremely low anti-fungal effect was also seen for blank films that are consistent with other studies showing that chitosan has antifungal activity against several fungi species (32).
There is a need for further in vivo studies examining the efficacy of formulations on superficial fungal infection.
Film forming property of chitosan made it interesting for drug delivery. Different studies have been performed to improve stability and physicochemical characteristics of chitosan film by blending it with other materials. Grant et al. used combination of chitosan and egg phosphatidylcholine to prepare composite films for localized drug delivery of paclitaxel and concluded that egg PC produces chitosan-based films with minimal swelling and a high degree of stability as a result of ionic and hydrogen bonding interactions between these two biomaterials (3).
Ahmed et al. evaluated potential drug delivery of chitosan films containing terbinafine HCl for topical treatment of fungal infections and found that incorporation of Glycerol triacetate as a plasticizer into medicated chitosan films, resulted in higher response in the inhibition zone sizes (2).
Dantas et al. prepared sodium alginate/chitosan based dressing films and provided improvement in burn healing together with low level laser therapy (33). Abruzzo et al. developed chitosan/gelatin films as a mucoadhesive system for buccal delivery of propranolol hydrochloride and adequate drug release and permeation as well as desirable film characteristic were achieved (34). Stefanescu et al. used blends of chitosan and cellulose and prepared a film with homogeneous structure (35). Kouchak et al. prepared blend films using chitosan and polyvinyl alcohol (PVA), containing nitrofurazone for burn wound dressing and concluded that addition of PVA at any concentration improved mechanical properties, reduced WVTR and increased swelling ratio of drug loaded chitosan films (10).
The aim of the present study was to formulate and characterize chitosan/soy PC film formulation for dermal delivery of griseofulvin. The effect of soy PC in modifying the physicochemical and mechanical properties of chitosan film containing 0.2% griseofulvin was evaluated. The tensile strength and elongation at break values of the films were improved by addition of soy PC to chitosan in weight ratio of 1 to 2. Presence of soy PC also led to lower swelling ratio and more stability of the films. The ability of phospholipids in ionic interactions and hydrogen bond formation with chitosan helped maintaining the structure of the film. Solubilizing activity of phospholipid led to higher Jss of drug release from PC2Cs2 than that of PC1Cs2, but PC1Cs2 released drug immediately with no lag time. PC2Cs2 showed more antifungal effect than PC1Cs2, which may be because of the higher flux of griseofulvin from PC2Cs2. These topical composite films had the potential for griseofulvin delivery to superficial fungal infections.
References
-
1.
Aggarwal N, Goindi S. Preparation and evaluation of antifungal efficacy of griseofulvin loaded deformable membrane vesicles in optimized guinea pig model of Microsporum canis--dermatophytosis. Int J Pharm. 2012;437(1-2):277-87. [PubMed ID: 22939964]. https://doi.org/10.1016/j.ijpharm.2012.08.015.
-
2.
Ahmed SM, Ibrahim MA, Sarhan HA, Amin MA. Formulation and Characterization of Biodgradable Chitosan Films for Topical Application of Terbinafine Hcl. Bull Pharm Sci Assiut Univ. 2007;30(2):111.
-
3.
Grant J, Blicker M, Piquette-Miller M, Allen C. Hybrid films from blends of chitosan and egg phosphatidylcholine for localized delivery of paclitaxel. J Pharm Sci. 2005;94(7):1512-27. [PubMed ID: 15920770]. https://doi.org/10.1002/jps.20379.
-
4.
Esmaeili A, Beni AA. A novel fixed-bed reactor design incorporating an electrospun PVA/chitosan nanofiber membrane. J Hazard Mater. 2014;280:788-96. [PubMed ID: 25240648]. https://doi.org/10.1016/j.jhazmat.2014.08.048.
-
5.
Sionkowska A, Planecka A, Lewandowska K, Michalska M. The influence of UV-irradiation on thermal and mechanical properties of chitosan and silk fibroin mixtures. J Photochem Photobiol B. 2014;140:301-5. [PubMed ID: 25218587]. https://doi.org/10.1016/j.jphotobiol.2014.08.017.
-
6.
Lai GJ, Shalumon KT, Chen SH, Chen JP. Composite chitosan/silk fibroin nanofibers for modulation of osteogenic differentiation and proliferation of human mesenchymal stem cells. Carbohydr Polym. 2014;111:288-97. [PubMed ID: 25037354]. https://doi.org/10.1016/j.carbpol.2014.04.094.
-
7.
Gholipour-Kanani A, Bahrami SH, Joghataie MT, Samadikuchaksaraei A, Ahmadi-Taftie H, Rabbani S, et al. Tissue engineered poly(caprolactone)-chitosan-poly(vinyl alcohol) nanofibrous scaffolds for burn and cutting wound healing. IET Nanobiotechnol. 2014;8(2):123-31. [PubMed ID: 25014084]. https://doi.org/10.1049/iet-nbt.2012.0050.
-
8.
Azizi S, Ahmad MB, Ibrahim NA, Hussein MZ, Namvar F. Cellulose nanocrystals/ZnO as a bifunctional reinforcing nanocomposite for poly(vinyl alcohol)/chitosan blend films: fabrication, characterization and properties. Int J Mol Sci. 2014;15(6):11040-53. [PubMed ID: 24945313]. https://doi.org/10.3390/ijms150611040.
-
9.
Tanase CE, Spiridon I. PLA/chitosan/keratin composites for biomedical applications. Mater Sci Eng C Mater Biol Appl. 2014;40:242-7. [PubMed ID: 24857489]. https://doi.org/10.1016/j.msec.2014.03.054.
-
10.
Kouchak M, Ameri A, Naseri B, Kargar Boldaji S. Chitosan and polyvinyl alcohol composite films containing nitrofurazone: preparation and evaluation. Iran J Basic Med Sci. 2014;17(1):14-20. [PubMed ID: 24592302].
-
11.
Lautenschläger H. Liposomes. In: Barel AO, Paye M, Maibach HI, editors. Handbook of Cosmetic Science and Technology. Boca Raton: CRC Press Taylor & Francis Group; 2006. p. 155-63.
-
12.
Vargas M, Albors A, Chiralt A, González-Martínez C. Characterization of chitosan–oleic acid composite films. Food Hydrocoll. 2009;23(2):536-47. https://doi.org/10.1016/j.foodhyd.2008.02.009.
-
13.
Pereda M, Ponce AG, Marcovich NE, Ruseckaite RA, Martucci JF. Chitosan-gelatin composites and bi-layer films with potential antimicrobial activity. Food Hydrocoll. 2011;25(5):1372-81. https://doi.org/10.1016/j.foodhyd.2011.01.001.
-
14.
Ojagh SM, Rezaei M, Razavi SH, Hosseini SMH. Development and evaluation of a novel biodegradable film made from chitosan and cinnamon essential oil with low affinity toward water. Food Chem. 2010;122(1):161-6. https://doi.org/10.1016/j.foodchem.2010.02.033.
-
15.
Tang C, Guan YX, Yao SJ, Zhu ZQ. Preparation of ibuprofen-loaded chitosan films for oral mucosal drug delivery using supercritical solution impregnation. Int J Pharm. 2014;473(1-2):434-41. [PubMed ID: 25079432]. https://doi.org/10.1016/j.ijpharm.2014.07.039.
-
16.
Rasool BK, Aziz US, Sarheed O, Rasool AA. Design and evaluation of a bioadhesive film for transdermal delivery of propranolol hydrochloride. Acta Pharm. 2011;61(3):271-82. [PubMed ID: 21945906]. https://doi.org/10.2478/v10007-011-0025-3.
-
17.
Jaafari MR, Bavarsad N, Bazzaz BS, Samiei A, Soroush D, Ghorbani S, et al. Effect of topical liposomes containing paromomycin sulfate in the course of Leishmania major infection in susceptible BALB/c mice. Antimicrob Agents Chemother. 2009;53(6):2259-65. [PubMed ID: 19223613]. https://doi.org/10.1128/AAC.01319-08.
-
18.
Sinko PJ, Singh Y, editors. Martin's Physical Pharmacy and Pharmaceutical Sciences. 6th ed. Lippincott Williams and Wilkins; 2006. p. 228-48.
-
19.
Gajra B, Pandya SS, Singh S, Rabari HA. Mucoadhesive hydrogel films of econazole nitrate: formulation and optimization using factorial design. J Drug Deliv. 2014;2014:305863. [PubMed ID: 25006462]. https://doi.org/10.1155/2014/305863.
-
20.
Aggarwal N, Goindi S, Mehta SD. Preparation and evaluation of dermal delivery system of griseofulvin containing vitamin E-TPGS as penetration enhancer. AAPS PharmSciTech. 2012;13(1):67-74. [PubMed ID: 22130790]. https://doi.org/10.1208/s12249-011-9722-y.
-
21.
Kassem MA, Esmat S, Bendas ER, El-Komy MH. Efficacy of topical griseofulvin in treatment of tinea corporis. Mycoses. 2006;49(3):232-5. [PubMed ID: 16681816]. https://doi.org/10.1111/j.1439-0507.2006.01221.x.
-
22.
Kołodziejska I, Kaczorowski K, Piotrowska B, Sadowska M. Modification of the properties of gelatin from skins of Baltic cod (Gadus morhua) with transglutaminase. Food Chem. 2004;86(2):203-9. https://doi.org/10.1016/j.foodchem.2003.08.036.
-
23.
Senel S, Ikinci G, Kas S, Yousefi-Rad A, Sargon MF, Hincal AA. Chitosan films and hydrogels of chlorhexidine gluconate for oral mucosal delivery. Int J Pharm. 2000;193(2):197-203. [PubMed ID: 10606782].
-
24.
Jridi M, Hajji S, Ayed HB, Lassoued I, Mbarek A, Kammoun M, et al. Physical, structural, antioxidant and antimicrobial properties of gelatin-chitosan composite edible films. Int J Biol Macromol. 2014;67:373-9. [PubMed ID: 24709012]. https://doi.org/10.1016/j.ijbiomac.2014.03.054.
-
25.
Caner C, Vergano PJ, Wiles JL. Chitosan Film Mechanical and Permeation Properties as Affected by Acid, Plasticizer, and Storage. J Food Sci. 2006;63(6):1049-53. https://doi.org/10.1111/j.1365-2621.1998.tb15852.x.
-
26.
Costa Idos S, Abranches RP, Garcia MT, Pierre MB. Chitosan-based mucoadhesive films containing 5-aminolevulinic acid for buccal cancer's treatment. J Photochem Photobiol B. 2014;140:266-75. [PubMed ID: 25190225]. https://doi.org/10.1016/j.jphotobiol.2014.08.005.
-
27.
Queen D, Evans JH, Gaylor JDS, Courtney JM, Reid WH. An in vitro assessment of wound dressing conformability. Biomater. 1987;8(5):372-6. https://doi.org/10.1016/0142-9612(87)90008-1.
-
28.
Binsi PK, Ravishankar CN, Srinivasa Gopal TK. Development and characterization of an edible composite film based on chitosan and virgin coconut oil with improved moisture sorption properties. J Food Sci. 2013;78(4):E526-34. [PubMed ID: 23464980]. https://doi.org/10.1111/1750-3841.12084.
-
29.
Hermans K, Van den Plas D, Kerimova S, Carleer R, Adriaensens P, Weyenberg W, et al. Development and characterization of mucoadhesive chitosan films for ophthalmic delivery of cyclosporine A. Int J Pharm. 2014;472(1-2):10-9. [PubMed ID: 24929014]. https://doi.org/10.1016/j.ijpharm.2014.06.017.
-
30.
Budai L, Kaszas N, Grof P, Lenti K, Maghami K, Antal I, et al. Liposomes for topical use: a physico-chemical comparison of vesicles prepared from egg or soy lecithin. Sci Pharm. 2013;81(4):1151-66. [PubMed ID: 24482779]. https://doi.org/10.3797/scipharm.1305-11.
-
31.
Chhonker YS, Prasad YD, Chandasana H, Vishvkarma A, Mitra K, Shukla PK, et al. Amphotericin-B entrapped lecithin/chitosan nanoparticles for prolonged ocular application. Int J Biol Macromol. 2015;72:1451-8. [PubMed ID: 25453292]. https://doi.org/10.1016/j.ijbiomac.2014.10.014.
-
32.
Sajomsang W, Gonil P, Saesoo S, Ovatlarnporn C. Antifungal property of quaternized chitosan and its derivatives. Int J Biol Macromol. 2012;50(1):263-9. [PubMed ID: 22100980]. https://doi.org/10.1016/j.ijbiomac.2011.11.004.
-
33.
Dantas MD, Cavalcante DR, Araujo FE, Barretto SR, Aciole GT, Pinheiro AL, et al. Improvement of dermal burn healing by combining sodium alginate/chitosan-based films and low level laser therapy. J Photochem Photobiol B. 2011;105(1):51-9. [PubMed ID: 21803596]. https://doi.org/10.1016/j.jphotobiol.2011.06.009.
-
34.
Abruzzo A, Bigucci F, Cerchiara T, Cruciani F, Vitali B, Luppi B. Mucoadhesive chitosan/gelatin films for buccal delivery of propranolol hydrochloride. Carbohydr Polym. 2012;87(1):581-8. https://doi.org/10.1016/j.carbpol.2011.08.024.
-
35.
Stefanescu C, Daly WH, Negulescu II. Biocomposite films prepared from ionic liquid solutions of chitosan and cellulose. Carbohydr Polym. 2012;87(1):435-43. https://doi.org/10.1016/j.carbpol.2011.08.003.