Abstract
Background:
Blood-brain barrier (BBB), as well-known protection for the brain, plays an active role in normal homeostasis. It might be changed by a range of inflammatory mediators to have a role in sickness behaviors.Objectives:
Regarding the anti-inflammatory effects of thymoquinone (TQ), its protection against BBB permeability, as a possible mechanism for protective effects against sickness behaviors elicited by lipopolysaccharide (LPS), was evaluated in rats.Methods:
The animals were grouped as follows and treated (n = 10 in each): (1) control (saline); (2) LPS 1 mg/kg, was injected two hours before behavioral tests for two weeks; (3-5) 2, 5, and 10 mg/kg TQ, respectively was injected 30 min before LPS injection. Open-field (OF), elevated plus-maze (EPM) and Forced Swimming test (FST) were done. Finally, the animals were anesthetized to evaluate for BBB permeability using Evans blue (EB) dye method.Results:
Compared with control, LPS decreased the peripheral distance and crossing and also total crossing and distance in OF, (P < 0.01 - P < 0.001). The central crossing and distance and central time in all three treatment groups were more than LPS (P < 0.05 - P < 0.001). LPS also reduced the entries and the time spent in the open arm while increased the time spent in the closed arm in EPM (P < 0.05 - P < 0.001). The effects of LPS were reversed by TQ (P < 0.05 - P < 0.001). In FST, the immobility time and active time were increased and decreased by LPS compared with control (P < 0.001), respectively. In all three TQ-treated groups, the active and climbing times were more while the immobility time was fewer than the LPS (P < 0.05 - P < 0.001). The animals of the LPS group showed more EB dye content in their brain tissue than the control group (P < 0.05 - P < 0.001). TQ significantly reduced EB dye content of the brain tissues (P < 0.05 - P < 0.001).Conclusions:
According to this study, protection against BBB permeability as a possible mechanism for the protective effects of TQ against sickness behaviors induced by LPS might be suggested.Keywords
Blood-Brain Barrier Thymoquinone Sickness Behavior Lipopolysaccharide
1. Background
The blood-brain barrier (BBB) plays a protective role for the brain by limiting the entry of neurotoxic blood-derived products into the brain (1). This layer that consists of specialized endothelial cells and surrounding pericytes and astrocytes is so sensitive to inflammatory insults, which can result in numerous neurocognitive syndromes (2). Several factors can destroy the BBB of which inflammation, that appears immediately following the insult, is the most common cause (3). Inflammation in the brain is one of the most causative factors in neurological diseases like depression (4), Alzheimer (5), multiple sclerosis (6) and Parkinson (7). It also seems to be responsible for behavioral responses that are known collectively as sickness behavior such as impairment of cognitive abilities, fatigue, and malaise, decreased locomotor, body weight and appetite (8). Inflammatory markers, including interleukin (IL)-1β, tumor necrosis factor (TNF-α), and IL-6, which communicate with the central nervous system (CNS) by vagal afferents and crossing the BBB, damage synapses and neurons, and ultimately lead to cognitive dysfunction (9). Lipopolysaccharide (LPS), is a piece of Gram-negative bacteria and is well-known as a potent inducer of inflammation. It is suggested to be able to increase BBB permeability by releasing cytokines (e.g., TNF-α, IL-6, IL-1β), prostaglandin E2, and nitric oxide (NO) (10, 11).
Anti-inflammatory agents are suggested to be useful to protect the BBB. Thymoquinone (TQ) a well-known component of Nigella sativa (NS) has been frequently reported to have anti-inflammatory effects (12-14). Other therapeutic effects of TQ consist of neuroprotective, antioxidant, anticonvulsant, and anti-tumor activity (15, 16).
2. Objectives
In this study, protection against BBB permeability, as a possible mechanism for protective effects of TQ against sickness behaviors induced by LPS, was investigated in rats.
3. Methods
3.1. Animals and Treatments
Male Wistar rats were obtained from a local animal center at Mashhad University of Medical Sciences for the experiments. They had a 70 - 80 days old and 240 ± 10 g weight. They were housed under standard status (temperature 22 ± 2°C, humidity of 54 ± 2%, and 12 h light/dark cycle). Food and water were freely accessible. Fifty of the rats were divided into five groups and treated (n = 10 in each group): (1) The animals in the control group received saline; (2) the rats in LPS group were injected by LPS (1mg/kg/day; i.p.); (3-5) the animals in these three groups received different doses of TQ (2 mg/kg in LPS-TQ 2, 5 mg/kg in LPS-TQ 5, and 10 mg/kg in LPS-TQ 10 group) and were then injected by LPS. The treatments were done for one week and were continued during behavioral tests. TQ was administered 30 min before LPS. LPS was injected 120 min before Behavioral tests. Both LPS and TQ were obtained from Sigma-Aldrich Company (Sigma Chemical Co). The drugs were dissolved in sterile saline prior to injection.
3.2. Behavioral Procedures
One week after the injection, an activity of the animals was evaluated in open-field (OF) by placing the rats into the center of a clear Plexiglas (100 × 100cm) for 5 min. A dim light was provided in a room and open-field was done. Activity was quantitated by a digital camera and the parameters such as the number of crossing in the central and peripheral zones, the traveled distance in central and peripheral zones, the time spent in central and peripheral zones, the total crossing number and traveled distance were recorded (16).
Elevated plus-maze (EPM) was a device designed with two closed and two open arms. The device was set 100 cm above the floor. Each rat was placed in the center of the arms and allowed to move inside the arms. Finally, the time spent and the number of entries into the open and closed arms were recorded. Forced Swimming test (FST) was performed for all groups as previously reported (16). In summary, each rat was compelled to swim in a water-filled tank. The total duration of immobility, as well as active and climbing times were recorded.
3.3. Blood-Brain Barrier Permeability
After completing the tests, the animals were anesthetized with urethane (1.6 g/kg) (17), then 20 mg/kg Evans blue dye was injected intravenously. After circulating for 20 minutes, in order to separate the brain, they were slaughtered. After launder with saline and drying, the tissues (cortex, cerebellum, and brain stem) were separately weighed and immersed in 5 mL formamide for 72 hours (18). The samples were homogenized with 2 ml trichloroacetic acid (TCA) 50% and then centrifuged (1500 rpm, 10 min). The absorbance of each tissue was measured at 632 nm (19).
3.4. Statistical Analysis
The data were presented as mean ± standard error of the mean (SEM). One-way ANOVA and Tuckey post hoc comparison tests were carried out. The P < 0.05 was considered statistically significant.
4. Results
4.1. Open-Field
According to the results, no significant difference was observed in the central crossing number between LPS and control groups (Figure 1A). The crossing number in the central zone by the animals of LPS-TQ 2 and LPS-TQ 5 groups was significantly more than the LPS group (P < 0.05-P < 0.001; Figure 1A); however, no significant difference was observed between LPS-TQ 10 and LPS groups (Figure 1A). The results also showed that the animals of LPS-TQ 5 group had a higher crossing number compared to the control group (P < 0.001). Central zone traveled distance was not significant between the LPS and control groups; however, this parameter in all three TQ-treated groups was more than LPS group (P < 0.05 - P < 0.001; Figure 1B). The animals of LPS-TQ 5 group had a longer central distance compared to the control group (P < 0.001). As shown in Figure 1C, the animals of the LPS group also spent a significant (P < 0.05) lower time in the central segment than the control group. However, treatment with TQ reversed this LPS-induced change and there was no significant difference between the LPS-TQ (2, 5, 10) and LPS groups.
A, Crossing; B, Distance; and C, Time in the central zone in the open field test. Data are shown as the mean ± standard error of the mean (SEM) (number of animals in each group is 10). *, P < 0.05; ***, P < 0.001 compared to the control group; +, P < 0.05; and +++, P < 0.001 compared to the LPS group.
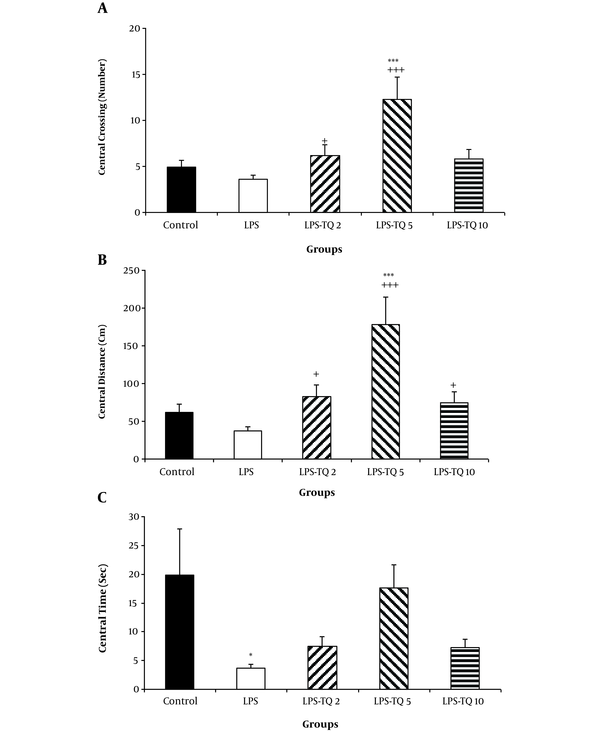
The results also revealed that the crossing number in the peripheral segment by the animals of the LPS group was fewer than that of the control group (P < 0.001; Figure 2A). According to Figure 2A, the peripheral crossing number by the rats of LPS-TQ 5 group was significantly more than the LPS group (P < 0.001; Figure 2A); however, no significant difference was observed between LPS-TQ 2, LPS-TQ 10 and LPS groups (Figure 2A). The peripheral zone distance in LPS and LPS-TQ2 groups was lower than the control group (P < 0.001and P < 0.05, respectively; Figure 2B). Peripheral zone distance in the LPS-TQ 5 group was more than the LPS group (P < 0.05; Figure 2B). However, no significant difference was observed in time spent in the peripheral zone between the LPS-TQ 2, LPS-TQ 10, and LPS groups (Figure 2B). No significant difference was seen between the groups when the peripheral zone time was compared between control and LPS groups as well as when the TQ-treated group was compared with the LPS group (Figure 2C).
A, Crossing; B, Distance; and C, Time in the peripheral zone in the open field test. Data are shown as the mean ± standard error of the mean (SEM) (number of animals in each group is 10). *, P < 0.05; ***, P < 0.001 compared to control group; +, P < 0.05; and +++, P < 0.001 compared to the LPS group.
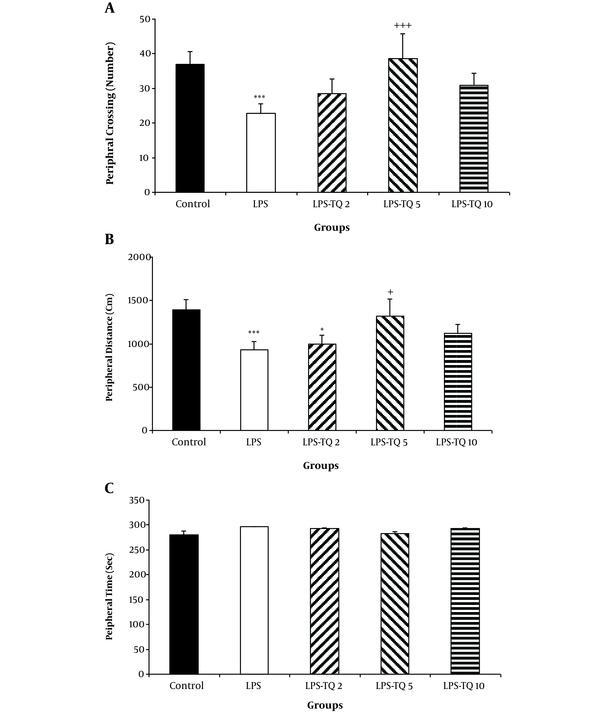
Additionally, the total crossing in the LPS group was lower than the control group (P < 0.01). The total crossing number in LPS-TQ 5 group was significantly more than the LPS group (P < 0.001, Figure 3A). No significant difference was seen between groups of LPS-TQ 2, LPS-TQ 10, and LPS. As shown in Figure 3B, the total distance in the LPS group was shorter than the control (P < 0.01) and LPS-TQ 5 (P < 0.05; Figure 3B) groups. But, there was no significant difference between LPS-TQ 2, LPS-TQ 10, and LPS groups. In addition, total distance in the LPS-TQ 2 group was lower than that in the control group (Figure 3B).
A, Total crossing; and B, Distance in the open field test. Data are shown as the mean ± standard error of the mean (SEM) (number of animals in each group is 10). *, P < 0.05; **, P < 0.01; and ***, P < 0.001 compared to control group; +, P < 0.05; and +++, P < 0.001 compared to the LPS group.
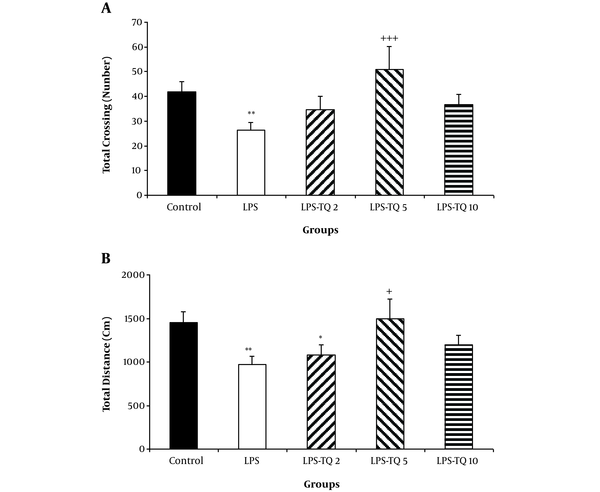
4.2. Elevated Plus-Maze
The open arm entries in the LPS group were lower than the control group (P < 0.01; Figure 4A). In all three TQ-treated groups, the open arm entries were more than the LPS group (P < 0.05 - P < 0.001; Figure 4A). The open arm entries in the LPS-TQ 10 group were more than the control group (P < 0.05; Figure 4A). The animals of the LPS-treated group also spent a long time in the open arm than the control ones (P < 0.05; Figure 4B). Treatment by all three doses of TQ prolonged the time spent in open arms (P < 0.01 - P < 0.001; Figure 4B). The open arm time in the LPS-TQ 10 group was more than the control group (P < 0.01; Figure 4B).
A, Open arm entries; and B, Time in the elevated plus-maze test. Data are shown as the mean ± standard error of the mean (SEM) (number of animals in each group is 10). *, P < 0.05; **, P < 0.01 compared to the control group; +, P < 0.05; ++, P < 0.01; and +++, P < 0.001 compared to the LPS group.
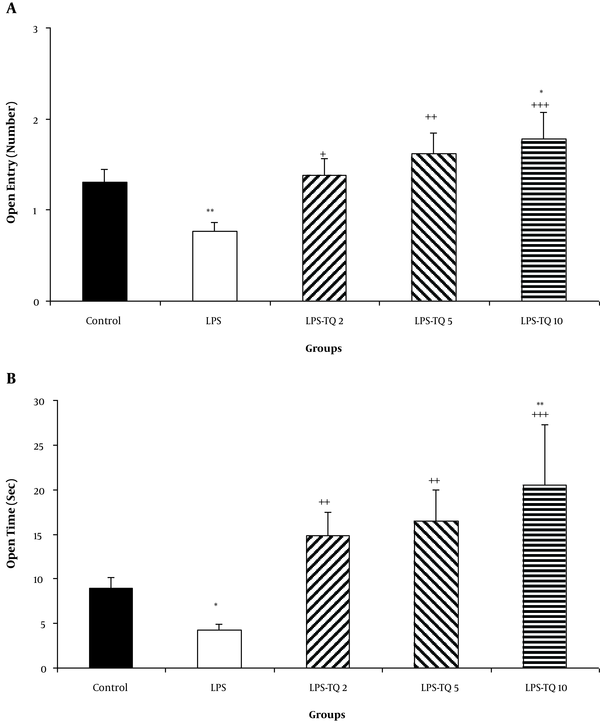
The results also showed that the animals of LPS, LPS-TQ 2 and LPS-TQ 5 groups were entered more frequently to the closed arm than the control ones (P < 0.001, P < 0.05, and P < 0.05 respectively; Figure 5A). No significant difference was observed when the entries to the closed arm were compared between LPS-TQ 5 and LPS groups, while this parameter in LPS-TQ 2 and LPS-TQ 10 groups was more than the LPS group (P < 0.05 - P < 0.001; Figure 5A). The time spent in the closed arm was no significantly different between the two control and LPS groups (Figure 5B). Compared to the LPS group, the LPS-TQ 5 group spent a lower time in the closed arm (P < 0.05; Figure 5B); however, there was no difference between the LPS-TQ 2, LPS-TQ 10, and LPS groups.
A, Closed arm entries; and B, time in the elevated plus-maze test. Data are shown as the mean ± standard error of the mean (SEM) (number of animals in each group is 10). *, P < 0.05; ***, P < 0.001 compared to the control group; +, P < 0.05; and +++, P < 0.001 compared to the LPS group.
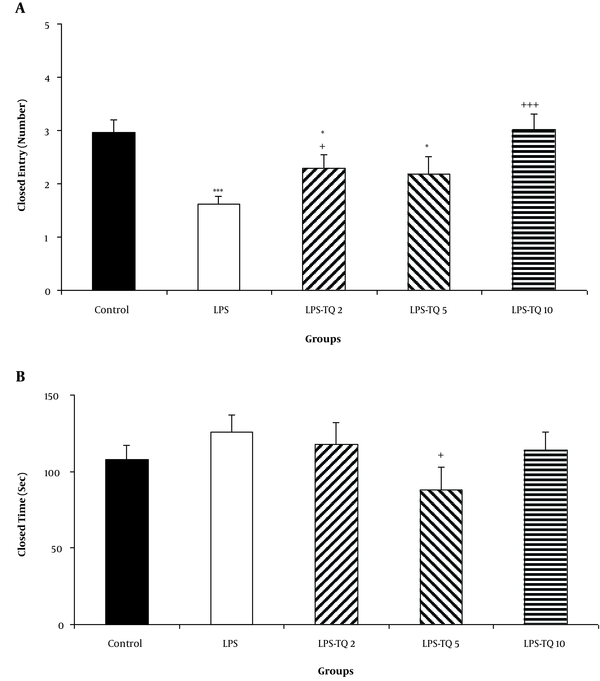
4.3. Forced Swimming Test
The results showed that LPS injection increased the immobility times in the LPS group compared to the control group (P < 0.001; Figure 6A). This parameter in all three TQ-treated groups was lower than the LPS group (P < 0.05 - P < 0.001; Figure 6A). Immobility times also in LPS-TQ 2, LPS-TQ 5, and LPS-TQ 10 groups were longer than that in the control group (P < 0.05 - P < 0.001; Figure 6A). Additionally, compared to the control group, LPS injection shortened the active times in the LPS group (P < 0.001; Figure 6B). However, this parameter in all three TQ-treated groups were more than the LPS group (P < 0.001; Figure 6B). The times also in LPS-TQ 5 and LPS-TQ 10 groups were shorter than that in the control group (P < 0.001; Figure 6B).
A, Immobility; and B, Active times in the forced swimming test. Data are shown as the mean ± standard error of the mean (SEM) (number of animals in each group is 10). *, P < 0.05; **, P < 0.01; ***, P < 0.001 compared to the control group; +, P < 0.05; and +++, P < 0.001 compared to the LPS group.
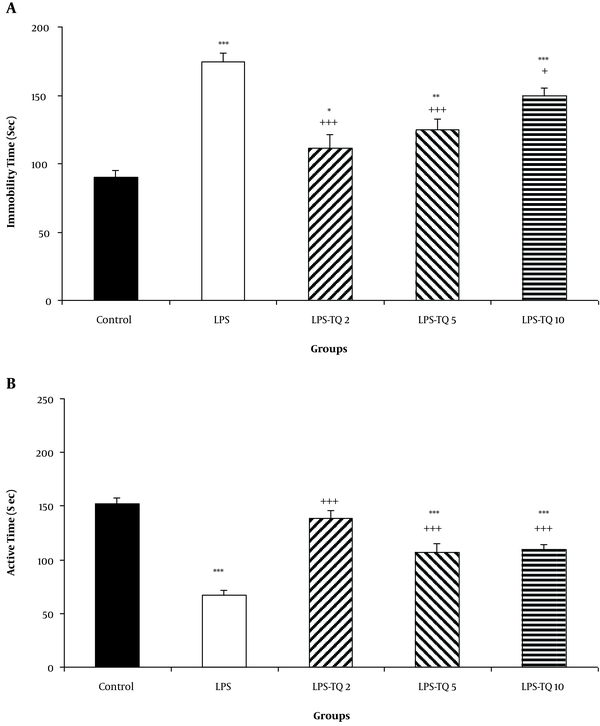
4.4. Blood-Brain Barrier Permeability
EB dye content in the brain tissues, including cortex, brain stem, and cerebellum of the LPS group was significantly more than the control group (P < 0.05 - P < 0.001; Figure 7A-C). Treatment by all three doses of the extract reduced EB dye content of the cortical tissues compared to the LPS group (P < 0.05 - P < 0.001; Figure 7A). The two highest doses of TQ also attenuated the EB content of the brain stem tissues (P < 0.05 - P < 0.001; Figure 7B). As Figure 7C shows there was no significant difference between the TQ-treated and LPS groups in the BBB permeability of cerebellum (Figure 7C).
EB dye content in the cortex (A), brain stem (B), and cerebellum(C). Data are shown as mean ± standard error of the mean (SEM) (number of animals in each group is 10). *, P < 0.05; ***, P < 0.001 compared to the control group; +, P < 0.05; ++, P < 0.01; and +++, P < 0.001 compared to the LPS group.
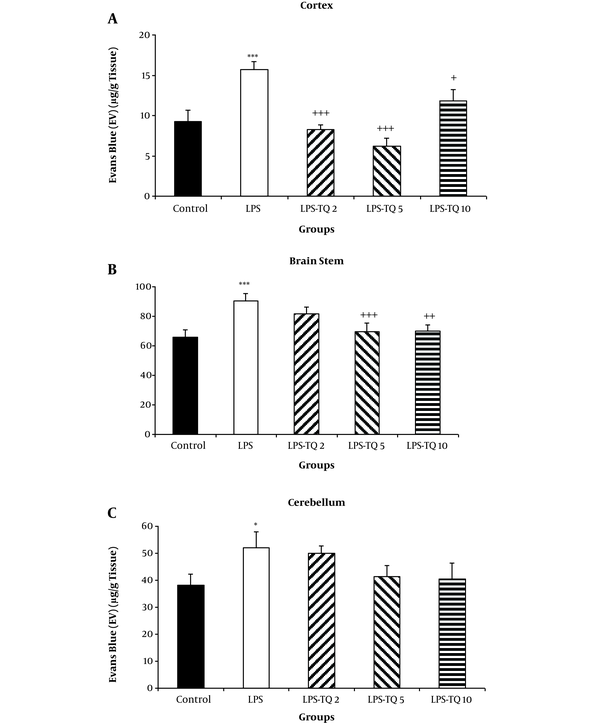
5. Discussion
In the current study, we showed that TQ was able to protect BBB and prevent the sickness behaviors induced by LPS in rats. Anatomical studies of BBB show that it is comprised of especially endothelial cells. These barriers are vital for normal physiological functions of the brain and spinal cord (1). Moreover, the BBB prevents free circulation of subtends between blood and CNS due to a high density of tight junction. This structure provides conditions for the proper functioning of the BBB (20). Studies show that some conditions affect this layer and change the permeability of the BBB (21), one of these conditions is inflammation (22). So inflammatory processes are known to disrupt BBB integrity (23, 24).
Inflammation is a physiological and primary response to various stimuli such as infection and tissue wounds. Inflammatory responses, on the one hand, stimulate the innate and acquired immune system and on the other hand, affect the epithelial duct as well as modify neuroendocrine status and behavioral changes, which lead to energy preservation such as increased sleep, lethargy, reduced appetite, and fever (25, 26). According to several studies, LPS, as a bacterial endotoxin and a powerful inducer of cytokine release, is frequently used to induce a common model for creating inflammation (23). Its effect is also known to cause neuronal inflammation (27). LPS leads to a release of NO, prostaglandins, and inflammatory cytokines such as IL-1, TNF-a, and IL-6 by activating macrophages through binding to toll-like receptor-4 (TLR4). In this condition, brain endothelial cells in the face of these materials lose their integrity (28-30). In this study, a higher EB dye content in the brain tissues of LPS-treated rats compared with the control ones confirms the destructive effect of LPS on BBB. It has been indicated that LPS induces BBB disruption through a cyclooxygenase (COX)-dependent pathway, possibly one that involves secretion of cytokines from the brain endothelial cells (31). In addition, experimental animal studies suggest that TNF-α alters the permeability of the BBB and due to a migration of leukocytes into the CNS by enhancing adhesion between leukocytes and BECs (32). On the other hand, TNF-α also induces NO generation, which later contributes to disruption of the BBB (33). It has been suggested that penetration of LPS to the BBB might be responsible for the events such as lethargy, fatigue, increased sleep, and reduced mobility and that collectively known as sickness behaviors (34).
In this study, TQ increased central crossing, central traveled distance, peripheral crossing, peripheral traveled distance, total crossing and total traveled distance in OF test. Considering all of these findings, accompanying protective effects of TQ against BBB permeability with the beneficial effects on sickness behaviors in LPS-induced model of rats might be suggested. Other studies also reported that TQ exerts its antioxidant and anti-inflammatory effects through inhibition of cytokines release (TNF-α, IL-1β). Studies show that TQ has also been able to suppress oxidative stress-induced neuropathy accompanying by a reduced level in nuclear factor kappa B activity in the brain and spinal cord (35, 36). In the present study, we did not compare the effects of TQ on BBB in different regions, including cortex, cerebellum, and brain stem, and the aim of this study was not such a comparison. However, at a glance, it seems that EB content in the cerebellum and brain stem tissues of the control group was more than that of cortex. We did not compare the effects of LPS on BBB between different parts of the brain. The effects of TQ have not also been previously evaluated but considering the previous studies, it seems that BBB is tighter in the cortex than the brain stem and the cerebellum (37-39); however, it needs to be more investigated in the future.
In this study, performances of the LPS-treated rats in EPM and OP tests also confirmed accompanying sickness behaviors with increasing of BBB permeability. Administration of LPS decreased the time spent in and the number of entries into the open arms of EPM and the time spent in a central area of apparatus, which might be considered a sign of anxiety-like behaviors in LPS-treated rats (16, 40). LPS also decreased the entries into the closed arm in EPM. The animals of the LPS group also had a lower crossing and a shorter traveled distance in the peripheral zone of apparatus. LPS also decreased the total traveled distance and total crossing number in the OF test. All of these findings confirm sickness behaviors induced by LPS injection (16, 40).
Medicinal plants have indicated considerable therapeutic effects possibly because of having several compounds with strong antioxidant and anti-inflammatory attributes (41). Owing to more adverse effects of synthetic antidepressants and on the other hand, expanding safe and effective agents from traditional herbs, researchers incline to use medicinal plants, and they found that a large number of antioxidants showed some beneficial effects on human health, as well as many of them such as NS, showed antidepressant-like effects (42). NS commonly known as the black seed is used as a natural treatment for some diseases. Researches have shown that the most effective treatment of NS is especially related to its constituent TQ (43, 44). It has been previously reported that TQ exerts an antidepressant-like effect by inhibiting the uptake of norepinephrine, serotonin, and dopamine (45). Perveen et al. (cited in Aquib) reported that TQ increased concentrations of 5 hydroxytryptamine (5-HT) and 5-hydroxy indole acetic acid (5-HIAA) in rats’ brains (46). Meanwhile, TQ is suggested to apply the antidepressant-like effect through antioxidant effects and protection against lipid peroxidation (47). Moreover, various studies have proved anti-inflammatory effects of TQ (46, 48). In this study, all doses of TQ decreased immobility times, while increased active time in FST, which confirms its anti-depression-like effects in an LPS-induced model in rats. As was mentioned above, BBB impairment has an important role in behavioral impairments due to neuroinflammation. Therefore, besides other mechanism(s) which were explained, protection against BBB rupture was examined as a mechanism for the beneficial effects of TQ in the brain. The results showed that EB dye contents of the brains of TQ-treated rats were lower than that of LPS-treated ones. EPM is a well-known experiment to assess anti-anxiety compounds. The compounds with anti-anxiety effects remarkably increased the entries into, and time spent in the open arms (49, 50). In this study, all doses of TQ increased the open arm entries and the time spent in the open arms of EPM, which might be considered its anti-anxiety effects. Supporting, TQ has previously shown to have anti-anxiety-like activity possibly through modulation of NO and gamma-aminobutyric acid (GABA) (51). The FST is broadly utilized for screening substances for potential antidepressant effects (52, 53). In this study, in addition to increasing BBB permeability, LPS injection increased immobility time while, decreased active time in the FST, which might be considered a depression-like behavior (54, 55) as well as a sign of sickness behavior induced by LPS in rats (16, 40). Accordingly, Hines et al. (56) also found that after LPS injection, the BBB breakdown occurred, which comforted the migration of macrophages into the brain; thus worsened neuroinflammatory and resulted in sickness behavior. Other studies also demonstrated that exposure to LPS reduced serotonin and noradrenaline levels in the brain and caused a change in emotion (57). Previously, it has been reported that LPS induces neuroinflammation by increasing BBB permeability, leading to neuronal damage, especially ineffective brain areas in emotion, including the limbic system (10). It has also been suggested that BBB disturbance may contribute to some neuropathology conditions such as multiple sclerosis, epilepsy and Alzheimer’s disease (58). On the other hand, inflammatory response in the brain has been found to create huge amounts of reactive oxygen species, which prompt oxidative stress and exacerbate the BBB damage (59, 60).
In conclusion, the results of this study showed that administration of LPS induced sickness behaviors in rats, which reflected impaired performances of the rats in FST, OF, and EPM. The sickness behaviors were accompanied by BBB impairments. TQ protected BBB disruption induced by LPS and also improved performances of the rats in behavioral tests. In the present study, considering the results of OF test and EPM in which TQ improved performances of the rats compared to the control group, it seems that TQ by itself has an anti-anxiety effect. Considering these results, besides other mechanism(s) which have been previously explained, protection against BBB permeability, as a possible mechanism for protective effects of TQ against sickness behaviors induced by LPS, is suggested; however, it needs to be more investigated.
Acknowledgements
References
-
1.
Varatharaj A, Galea I. The blood-brain barrier in systemic inflammation. Brain Behav Immun. 2017;60:1-12. [PubMed ID: 26995317]. https://doi.org/10.1016/j.bbi.2016.03.010.
-
2.
Abbott NJ, Ronnback L, Hansson E. Astrocyte-endothelial interactions at the blood-brain barrier. Nat Rev Neurosci. 2006;7(1):41-53. [PubMed ID: 16371949]. https://doi.org/10.1038/nrn1824.
-
3.
Siegal T, Rubinstein R, Bokstein F, Schwartz A, Lossos A, Shalom E, et al. In vivo assessment of the window of barrier opening after osmotic blood-brain barrier disruption in humans. J Neurosurg. 2000;92(4):599-605. [PubMed ID: 10761648]. https://doi.org/10.3171/jns.2000.92.4.0599.
-
4.
Dantzer R, O'Connor JC, Lawson MA, Kelley KW. Inflammation-associated depression: from serotonin to kynurenine. Psychoneuroendocrinology. 2011;36(3):426-36. [PubMed ID: 21041030]. [PubMed Central ID: PMC3053088]. https://doi.org/10.1016/j.psyneuen.2010.09.012.
-
5.
Holmes C, Cunningham C, Zotova E, Woolford J, Dean C, Kerr S, et al. Systemic inflammation and disease progression in Alzheimer disease. Neurology. 2009;73(10):768-74. [PubMed ID: 19738171]. [PubMed Central ID: PMC2848584]. https://doi.org/10.1212/WNL.0b013e3181b6bb95.
-
6.
Frischer JM, Bramow S, Dal-Bianco A, Lucchinetti CF, Rauschka H, Schmidbauer M, et al. The relation between inflammation and neurodegeneration in multiple sclerosis brains. Brain. 2009;132(Pt 5):1175-89. [PubMed ID: 19339255]. [PubMed Central ID: PMC2677799]. https://doi.org/10.1093/brain/awp070.
-
7.
Asgharzadeh F, Rouzbahani R, Khazaei M. Chronic low-grade inflammation: Etiology and its effects. J Isfahan Med Sch. 2016;34:408-21.
-
8.
Dantzer R. Cytokine-induced sickness behaviour: a neuroimmune response to activation of innate immunity. Eur J Pharmacol. 2004;500(1-3):399-411. [PubMed ID: 15464048]. https://doi.org/10.1016/j.ejphar.2004.07.040.
-
9.
Luo A, Yan J, Tang X, Zhao Y, Zhou B, Li S. Postoperative cognitive dysfunction in the aged: the collision of neuroinflammaging with perioperative neuroinflammation. Inflammopharmacology. 2019;27(1):27-37. [PubMed ID: 30607668]. https://doi.org/10.1007/s10787-018-00559-0.
-
10.
Banks WA, Gray AM, Erickson MA, Salameh TS, Damodarasamy M, Sheibani N, et al. Lipopolysaccharide-induced blood-brain barrier disruption: roles of cyclooxygenase, oxidative stress, neuroinflammation, and elements of the neurovascular unit. J Neuroinflammation. 2015;12:223. [PubMed ID: 26608623]. [PubMed Central ID: PMC4660627]. https://doi.org/10.1186/s12974-015-0434-1.
-
11.
Tunkel AR, Rosser SW, Hansen EJ, Scheld WM. Blood-brain barrier alterations in bacterial meningitis: development of an in vitro model and observations on the effects of lipopolysaccharide. In Vitro Cell Dev Biol. 1991;27A(2):113-20. [PubMed ID: 1826902]. https://doi.org/10.1007/BF02630996.
-
12.
Hassan S, Ahmed WA, Galeb FM, El-Taweel MA. In vitro challenge using thymoquinone on (HepG2) cell line. Toxicol Lett. 2009;189. https://doi.org/10.1016/j.toxlet.2009.06.400.
-
13.
Shabana A, El-Menyar A, Asim M, Al-Azzeh H, Al Thani H. Cardiovascular benefits of black cumin (Nigella sativa). Cardiovasc Toxicol. 2013;13(1):9-21. [PubMed ID: 22911452]. https://doi.org/10.1007/s12012-012-9181-z.
-
14.
Sultan MT, Butt MS, Karim R, Ahmed W, Kaka U, Ahmad S, et al. Nigella sativa fixed and essential oil modulates glutathione redox enzymes in potassium bromate induced oxidative stress. BMC Complement Altern Med. 2015;15:330. [PubMed ID: 26385559]. [PubMed Central ID: PMC4575498]. https://doi.org/10.1186/s12906-015-0853-7.
-
15.
Asgharzadeh F, Bargi R, Beheshti F, Hosseini M, Farzadnia M, Khazaei M. Thymoquinone Prevents Myocardial and Perivascular Fibrosis Induced by Chronic Lipopolysaccharide Exposure in Male Rats: - Thymoquinone and Cardiac Fibrosis. J Pharmacopuncture. 2018;21(4):284-93. [PubMed ID: 30652055]. [PubMed Central ID: PMC6333195]. https://doi.org/10.3831/KPI.2018.21.032.
-
16.
Azizi-Malekabadi H, Hosseini M, Pourganji M, Zabihi H, Saeedjalali M, Anaeigoudari A. Deletion of ovarian hormones induces a sickness behavior in rats comparable to the effect of lipopolysaccharide. Neurol Res Int. 2015;2015:627642. [PubMed ID: 25705518]. [PubMed Central ID: PMC4325213]. https://doi.org/10.1155/2015/627642.
-
17.
Anaeigoudari A, Norouzi F, Abareshi A, Beheshti F, Aaghaei A, Shafei MN, et al. Protective effects of Nigella sativa on synaptic plasticity impairment induced by lipopolysaccharide. Vet Res Forum. 2018;9(1):27-33. [PubMed ID: 29719661]. [PubMed Central ID: PMC5913558].
-
18.
Hu N, Guo D, Wang H, Xie K, Wang C, Li Y, et al. Involvement of the blood-brain barrier opening in cognitive decline in aged rats following orthopedic surgery and high concentration of sevoflurane inhalation. Brain Res. 2014;1551:13-24. [PubMed ID: 24440777]. https://doi.org/10.1016/j.brainres.2014.01.015.
-
19.
Uyama O, Okamura N, Yanase M, Narita M, Kawabata K, Sugita M. Quantitative evaluation of vascular permeability in the gerbil brain after transient ischemia using Evans blue fluorescence. J Cereb Blood Flow Metab. 1988;8(2):282-4. [PubMed ID: 3343300]. https://doi.org/10.1038/jcbfm.1988.59.
-
20.
Zlokovic BV. The blood-brain barrier in health and chronic neurodegenerative disorders. Neuron. 2008;57(2):178-201. [PubMed ID: 18215617]. https://doi.org/10.1016/j.neuron.2008.01.003.
-
21.
Wardlaw JM, Sandercock PA, Dennis MS, Starr J. Is breakdown of the blood-brain barrier responsible for lacunar stroke, leukoaraiosis, and dementia? Stroke. 2003;34(3):806-12. [PubMed ID: 12624314]. https://doi.org/10.1161/01.STR.0000058480.77236.B3.
-
22.
Stolp HB, Dziegielewska KM. Review: Role of developmental inflammation and blood-brain barrier dysfunction in neurodevelopmental and neurodegenerative diseases. Neuropathol Appl Neurobiol. 2009;35(2):132-46. [PubMed ID: 19077110]. https://doi.org/10.1111/j.1365-2990.2008.01005.x.
-
23.
Minami T, Okazaki J, Kawabata A, Kuroda R, Okazaki Y. Penetration of cisplatin into mouse brain by lipopolysaccharide. Toxicology. 1998;130(2-3):107-13. [PubMed ID: 9865478]. https://doi.org/10.1016/s0300-483x(98)00103-6.
-
24.
Xaio H, Banks WA, Niehoff ML, Morley JE. Effect of LPS on the permeability of the blood–brain barrier to insulin. Brain Res. 2001;896(1-2):36-42. https://doi.org/10.1016/s0006-8993(00)03247-9.
-
25.
Suffredini AF, Fantuzzi G, Badolato R, Oppenheim JJ, O'Grady NP. New insights into the biology of the acute phase response. J Clin Immunol. 1999;19(4):203-14. [PubMed ID: 10471974]. https://doi.org/10.1023/a:1020563913045.
-
26.
Lucas SM, Rothwell NJ, Gibson RM. The role of inflammation in CNS injury and disease. Br J Pharmacol. 2006;147 Suppl 1:S232-40. [PubMed ID: 16402109]. [PubMed Central ID: PMC1760754]. https://doi.org/10.1038/sj.bjp.0706400.
-
27.
Qin L, Wu X, Block ML, Liu Y, Breese GR, Hong JS, et al. Systemic LPS causes chronic neuroinflammation and progressive neurodegeneration. Glia. 2007;55(5):453-62. [PubMed ID: 17203472]. [PubMed Central ID: PMC2871685]. https://doi.org/10.1002/glia.20467.
-
28.
Cao C, Matsumura K, Yamagata K, Watanabe Y. Involvement of cyclooxygenase-2 in LPS-induced fever and regulation of its mRNA by LPS in the rat brain. Am J Physiol. 1997;272(6 Pt 2):R1712-25. [PubMed ID: 9227582]. https://doi.org/10.1152/ajpregu.1997.272.6.R1712.
-
29.
Reyes TM, Fabry Z, Coe CL. Brain endothelial cell production of a neuroprotective cytokine, interleukin-6, in response to noxious stimuli. Brain Res. 1999;851(1-2):215-20. [PubMed ID: 10642846]. https://doi.org/10.1016/s0006-8993(99)02189-7.
-
30.
Gutierrez EG, Banks WA, Kastin AJ. Murine tumor necrosis factor alpha is transported from blood to brain in the mouse. J Neuroimmunol. 1993;47(2):169-76. [PubMed ID: 8370768]. https://doi.org/10.1016/0165-5728(93)90027-v.
-
31.
Engstrom L, Ruud J, Eskilsson A, Larsson A, Mackerlova L, Kugelberg U, et al. Lipopolysaccharide-induced fever depends on prostaglandin E2 production specifically in brain endothelial cells. Endocrinology. 2012;153(10):4849-61. [PubMed ID: 22872578]. https://doi.org/10.1210/en.2012-1375.
-
32.
Pober JS, Cotran RS. Cytokines and endothelial cell biology. Physiol Rev. 1990;70(2):427-51. [PubMed ID: 1690903]. https://doi.org/10.1152/physrev.1990.70.2.427.
-
33.
Mayhan WG. Role of nitric oxide in disruption of the blood-brain barrier during acute hypertension. Brain Res. 1995;686(1):99-103. [PubMed ID: 7583277]. https://doi.org/10.1016/0006-8993(95)00460-8.
-
34.
Shalev H, Serlin Y, Friedman A. Breaching the blood-brain barrier as a gate to psychiatric disorder. Cardiovasc Psychiatry Neurol. 2009;2009:278531. [PubMed ID: 19936105]. [PubMed Central ID: PMC2775634]. https://doi.org/10.1155/2009/278531.
-
35.
Hamdy NM, Taha RA. Effects of Nigella sativa oil and thymoquinone on oxidative stress and neuropathy in streptozotocin-induced diabetic rats. Pharmacology. 2009;84(3):127-34. [PubMed ID: 19684436]. https://doi.org/10.1159/000234466.
-
36.
Mohamed A, Afridi DM, Garani O, Tucci M. Thymoquinone inhibits the activation of NF-kappaB in the brain and spinal cord of experimental autoimmune encephalomyelitis. Biomed Sci Instrum. 2005;41:388-93. [PubMed ID: 15850137].
-
37.
Ikeda M, Bhattacharjee AK, Kondoh T, Nagashima T, Tamaki N. Synergistic effect of cold mannitol and Na(+)/Ca(2+) exchange blocker on blood-brain barrier opening. Biochem Biophys Res Commun. 2002;291(3):669-74. [PubMed ID: 11855842]. https://doi.org/10.1006/bbrc.2002.6495.
-
38.
Bhattacharjee AK, Nagashima T, Kondoh T, Tamaki N. Quantification of early blood-brain barrier disruption by in situ brain perfusion technique. Brain Res Brain Res Protoc. 2001;8(2):126-31. [PubMed ID: 11673094]. https://doi.org/10.1016/s1385-299x(01)00094-0.
-
39.
Skultetyova I, Tokarev D, Jezova D. Stress-induced increase in blood-brain barrier permeability in control and monosodium glutamate-treated rats. Brain Res Bull. 1998;45(2):175-8. [PubMed ID: 9443836]. https://doi.org/10.1016/s0361-9230(97)00335-3.
-
40.
Norouzi F, Abareshi A, Anaeigoudari A, Shafei MN, Gholamnezhad Z, Saeedjalali M, et al. The effects of Nigella sativa on sickness behavior induced by lipopolysaccharide in male Wistar rats. Avicenna J Phytomed. 2016;6(1):104-16. [PubMed ID: 27247927]. [PubMed Central ID: PMC4884223].
-
41.
Farzaneh V, Carvalho IS. A review of the health benefit potentials of herbal plant infusions and their mechanism of actions. Ind Crops Prod. 2015;65:247-58. https://doi.org/10.1016/j.indcrop.2014.10.057.
-
42.
Kanter M, Coskun O, Uysal H. The antioxidative and antihistaminic effect of Nigella sativa and its major constituent, thymoquinone on ethanol-induced gastric mucosal damage. Arch Toxicol. 2006;80(4):217-24. [PubMed ID: 16240107]. https://doi.org/10.1007/s00204-005-0037-1.
-
43.
Ali BH, Blunden G. Pharmacological and toxicological properties of Nigella sativa. Phytother Res. 2003;17(4):299-305. [PubMed ID: 12722128]. https://doi.org/10.1002/ptr.1309.
-
44.
Badary OA, Taha RA, Gamal el-Din AM, Abdel-Wahab MH. Thymoquinone is a potent superoxide anion scavenger. Drug Chem Toxicol. 2003;26(2):87-98. [PubMed ID: 12816394]. https://doi.org/10.1081/dct-120020404.
-
45.
Elkhayat ES, Alorainy MS, El-Ashmawy IM, Fat'hi S. Potential Antidepressant Constituents of Nigella sativa Seeds. Pharmacogn Mag. 2016;12(Suppl 1):S27-31. [PubMed ID: 27041854]. [PubMed Central ID: PMC4791995]. https://doi.org/10.4103/0973-1296.176118.
-
46.
Aquib M, Najmi AK, Akhtar M. Antidepressant Effect of Thymoquinone in Animal Models of Depression. Drug Res (Stuttg). 2015;65(9):490-4. [PubMed ID: 25207705]. https://doi.org/10.1055/s-0034-1389920.
-
47.
Houghton PJ, Zarka R, de las Heras B, Hoult JR. Fixed oil of Nigella sativa and derived thymoquinone inhibit eicosanoid generation in leukocytes and membrane lipid peroxidation. Planta Med. 1995;61(1):33-6. [PubMed ID: 7700988]. https://doi.org/10.1055/s-2006-957994.
-
48.
Ghannadi A, Hajhashemi V, Jafarabadi H. An investigation of the analgesic and anti-inflammatory effects of Nigella sativa seed polyphenols. J Med Food. 2005;8(4):488-93. [PubMed ID: 16379560]. https://doi.org/10.1089/jmf.2005.8.488.
-
49.
Pellow S, Chopin P, File SE, Briley M. Validation of open:closed arm entries in an elevated plus-maze as a measure of anxiety in the rat. J Neurosci Methods. 1985;14(3):149-67. [PubMed ID: 2864480]. https://doi.org/10.1016/0165-0270(85)90031-7.
-
50.
Denenberg VH. Open-field bheavior in the rat: what does it mean? Ann N Y Acad Sci. 1969;159(3):852-9. [PubMed ID: 5260302]. https://doi.org/10.1111/j.1749-6632.1969.tb12983.x.
-
51.
Gilhotra N, Dhingra D. Thymoquinone produced antianxiety-like effects in mice through modulation of GABA and NO levels. Pharmacol Rep. 2011;63(3):660-9. [PubMed ID: 21857076]. https://doi.org/10.1016/s1734-1140(11)70577-1.
-
52.
Ramos A, Mormede P. Stress and emotionality: a multidimensional and genetic approach. Neurosci Biobehav Rev. 1998;22(1):33-57. [PubMed ID: 9491939]. https://doi.org/10.1016/s0149-7634(97)00001-8.
-
53.
Porsolt RD, Le Pichon M, Jalfre M. Depression: a new animal model sensitive to antidepressant treatments. Nature. 1977;266(5604):730-2. [PubMed ID: 559941]. https://doi.org/10.1038/266730a0.
-
54.
Neamati A, Chaman F, Hosseini M, Boskabady MH. The effects of Valeriana officinalis L. hydro-alcoholic extract on depression like behavior in ovalbumin sensitized rats. J Pharm Bioallied Sci. 2014;6(2):97-103. [PubMed ID: 24741277]. [PubMed Central ID: PMC3983753]. https://doi.org/10.4103/0975-7406.129174.
-
55.
Hosseini M, Zakeri S, Khoshdast S, Yousefian FT, Rastegar M, Vafaee F, et al. The effects of Nigella sativa hydro-alcoholic extract and thymoquinone on lipopolysaccharide - induced depression like behavior in rats. J Pharm Bioallied Sci. 2012;4(3):219-25. [PubMed ID: 22923964]. [PubMed Central ID: PMC3425171]. https://doi.org/10.4103/0975-7406.99052.
-
56.
Hines DJ, Choi HB, Hines RM, Phillips AG, MacVicar BA. Prevention of LPS-induced microglia activation, cytokine production and sickness behavior with TLR4 receptor interfering peptides. PLoS One. 2013;8(3). e60388. [PubMed ID: 23555964]. [PubMed Central ID: PMC3610686]. https://doi.org/10.1371/journal.pone.0060388.
-
57.
Depino AM. Early prenatal exposure to LPS results in anxiety- and depression-related behaviors in adulthood. Neuroscience. 2015;299:56-65. [PubMed ID: 25943476]. https://doi.org/10.1016/j.neuroscience.2015.04.065.
-
58.
Tomkins O, Friedman O, Ivens S, Reiffurth C, Major S, Dreier JP, et al. Blood-brain barrier disruption results in delayed functional and structural alterations in the rat neocortex. Neurobiol Dis. 2007;25(2):367-77. [PubMed ID: 17188501]. https://doi.org/10.1016/j.nbd.2006.10.006.
-
59.
Candelario-Jalil E, Mhadu NH, Al-Dalain SM, Martinez G, Leon OS. Time course of oxidative damage in different brain regions following transient cerebral ischemia in gerbils. Neurosci Res. 2001;41(3):233-41. [PubMed ID: 11672836]. https://doi.org/10.1016/s0168-0102(01)00282-6.
-
60.
Bromont C, Marie C, Bralet J. Increased lipid peroxidation in vulnerable brain regions after transient forebrain ischemia in rats. Stroke. 1989;20(7):918-24. [PubMed ID: 2749850]. https://doi.org/10.1161/01.str.20.7.918.