Abstract
Background:
Anatomy embraces various fields and is regarded as the foundation of medical sciences due to its broad clinical applications, particularly in surgery. However, teaching this subject, particularly in practical units, poses challenges, and insufficient anatomical knowledge and errors in anatomy-related decisions by physicians can lead to avoidable deaths and medical errors. This study aimed to design and develop an electronic moulage (EM) for teaching upper limb anatomy, specifically focusing on the muscles and brachial plexus (BP).Methods:
This was a quasi-experimental study with a pre-test-post-test design. Electronic moulage has been implemented in a manner that allows independent structures within a compartment to be taught without the presence of a teacher or anatomical atlases and enables learners to comprehend the anatomical features and relationships of structures in both combined and isolated modes, even without prior knowledge of anatomical theory specific to that compartment. The EM incorporates a skeletal compartment system and a BP component, utilizing audio and visual systems to deliver a comprehensive educational experience. The effectiveness of the EM was evaluated by experts in anatomy and medical science students, comparing it to other teaching methods. Four groups of undergraduate medical radiology students with no prior instruction in upper limb anatomy participated in the study. Each group was assigned to a different training method, including lecture-based education, digital-based education with a simulator, cadaver-based education, and EM-based education.Results:
After assessing the results using educational and motivational indicators, it was determined that the EM demonstrated innovative potential and could enhance motivation and the quality of anatomy education. Comparing the post-test scores of the EM-based education group to the other groups revealed significantly higher scores in the EM-based group. This finding indicates that the EM has the potential to improve the effectiveness and efficiency of anatomy education, addressing the limitations of other teaching methods.Conclusions:
The developed EM presents a promising solution for enhancing anatomy education, particularly in the context of upper limb anatomy. The innovative features of EM and its ability to improve motivation and learning outcomes make it a valuable tool for teaching this subject matter.Keywords
Moulage Simulator Brachial Plexus Upper Limb Anatomy Education
1. Background
Anatomy encompasses various fields, including human, animal, and plant anatomy, and is regarded as the foundation of medical sciences due to its broad clinical applications, particularly in surgery. However, teaching this subject, particularly in practical units, poses challenges and is often less effective with traditional teaching methods due to time constraints and the vast amount of material to cover (1). Furthermore, certain countries, such as Iran, encounter difficulties in accessing cadavers for dissection due to religious beliefs, cultural attitudes toward body donation, and the associated costs of acquiring, preserving, and maintaining cadavers. Numerous studies have highlighted the inadequate knowledge of surgeons regarding anatomical structures and relationships as a significant factor contributing to perioperative deaths (2).
Ellis discovered a sevenfold increase in complaints about general practitioners’ insufficient anatomical knowledge reported to the Medical Defense Union in the UK between 1995 and 2000, with 32% of these complaints relating to inadequate understanding of anatomical structures and underlying injuries (2). Cahill et al. further revealed that 80 000 preventable deaths occur in the United States annually due to physicians’ insufficient anatomical knowledge and related errors (3). According to the Global Burden of Disease study, which examines global mortality and morbidity causes, an estimated 9.6 million deaths in 2019 were potentially avoidable. These deaths encompassed communicable diseases, non-communicable diseases, injuries, and other causes that could have been prevented through effective interventions, such as vaccination, sanitation, and improved healthcare accessibility (4).
Insufficient anatomical knowledge and errors in anatomy-related decisions by physicians can lead to avoidable deaths and medical errors. A global study involving over 1,000 medical students from 30 countries highlighted the widespread limited anatomical knowledge among medical students, as they performed poorly on anatomical knowledge tests (5, 6). In a study conducted by Kowalczyk and Majewski the relationship between surgical errors and anatomical variations was examined. The aforementioned study revealed that 6.8% of surgical errors occurred in laparoscopic colorectal surgery. Additionally, it was reported that 20% of patients undergoing inguinal hernia surgery experienced numbness in the inguinal, hypogastric, and genital regions due to damage to the ilioinguinal and iliohypogastric nerves (7). To address these challenges, technological advancements in medical education and innovative teaching methods have emerged to enhance the effectiveness of anatomy education. Current teaching methods for anatomy include theoretical education, cadaver dissection, examination of specimens during surgical procedures (surgical anatomy), the use of moulages (e.g., mannequins and molds), surface anatomy, and radiological anatomy. Modern educational tools, such as ultrasound and arthroscopy simulations, have also been integrated into anatomy education (8).
During medieval times, the teaching of anatomy involved dissecting corpses. However, in the 17th century, Naples introduced the first wax moulages, which were replicas of body parts used for medical education. Artists such as Jules Bartha further refined this technique in the 19th century, with St. Louis Hospital in Paris utilizing thousands of wax moulages for educational purposes (9, 10). Over time, colored wax was employed to create moulages depicting organs. In the late 19th and early 20th centuries, rubber and latex polymers were incorporated, and presently, advanced technologies, such as three-dimensional (3D) printing and medical imaging, are utilized to produce highly detailed and flexible moulages (11). Research indicates that the use of 3D moulages in anatomy education leads to improved efficiency, enhanced quality, and increased engagement.
2. Objectives
With this background in mind, the objective of this study was to design an electronic moulage (EM) that offers additional information compared to traditional wax moulages (12). The EM serves as an educational tool capable of teaching complex organs and body systems, employing advanced electronic and robotic design methods. The present study also focused on developing a prototype EM specifically designed to demonstrate the relationship between the brachial plexus (BP) and upper limb muscles, incorporating sound and light systems. This EM provides an analytical and practical learning experience enriched with features not present in traditional wax moulages.
3. Methods
3.1. Electronic Moulage Design and Performance
This was a quasi-experimental study with a pre-test-post-test design. The first brachial and lumbar network moulage for student education was created by this research team in 2018 and was used in anatomy practical classes for medical students, especially radiology students. After that, in 2019, a brachial network moulage was created next to the axillary artery. Positive feedback was received from students after training, and understanding the nervous system became easier for them. Finally, this team decided to create an electronic brachial network moulage alongside the muscles to facilitate learning for students.
As mentioned earlier, EM has addressed the limitations of traditional moulages in the field of anatomy education and has been implemented in a manner that allows independent structures within a compartment to be taught without the presence of a teacher or anatomical atlases. This is achieved through the integration of auditory, visual, and mechanical systems in the EM, enabling learners to comprehend the anatomical features and relationships of structures in both combined and isolated modes, even without prior knowledge of anatomical theory specific to that compartment. In contrast, traditional moulages always require the presence of a teacher and anatomical atlases, in addition to a prior understanding of anatomical theory. Moreover, traditional moulages often overlook the anatomical performance of structures and their natural surroundings.
Another advantage of EM is its ability to enhance motivation in anatomy learning. By incorporating auditory and visual capabilities into the structures, it eliminates fatigue during the learning process and transforms it into an interactive, exploratory, and engaging experience. The design of EM utilized the Delphi method developed by Stokes-Parish et al., which is a comprehensive program for creating electronic educational moulages. This approach focuses on performance and adaptability, and the design process consists of three stages: Overall idea, construction, and performance (13).
The designed and constructed EM for this purpose encompasses three valuable functions:
• Muscle Naming: It enables the expression of muscle names.
• Nerve-Muscle Connection: When the nerve keys are pressed, it visually demonstrates the connection between the nerves of the BP and the muscles in the brachial area by illuminating them.
• Illuminated Parts: The EM includes parts with the names of muscles (in red) and nerves (in yellow) written on them, which can be illuminated. This feature allows users to familiarize themselves with the structure and text of muscle and nerve names. When activated, the corresponding pages, the name of the nerve, and the associated functional muscle illuminate simultaneously, enabling users to observe the connection between the two sections.
These three unique characteristics are not found in other moulages. Additionally, the simulation of the BP in this moulage sets it apart from existing educational moulages. It consists of two parts: The BP on one side and the skeletal system of the right hand, along with four brachial muscles, on the other side. These components are electronically interconnected.
The moulage operates in three performance modes:
• Muscle Illumination: Pressing the terminal nerve keys causes the corresponding muscles to illuminate.
• Nerve Illumination: Pressing any key on the nerves illuminates the name of that nerve and the related muscles on the side box.
• Muscle Key Illumination: Pressing the keys attached to the muscles illuminates the names of the corresponding muscles on the side box (14, 15).
3.2. Different Components of Electronic Moulage
As previously mentioned, the electronic model consists of two distinct parts: The neural BP of the arm compartment on one side and the skeletal system of the right hand, including the arm muscles, on the other side (Figure 1).
Two parts of EM (the BP and associated muscles of the upper limb in EM).
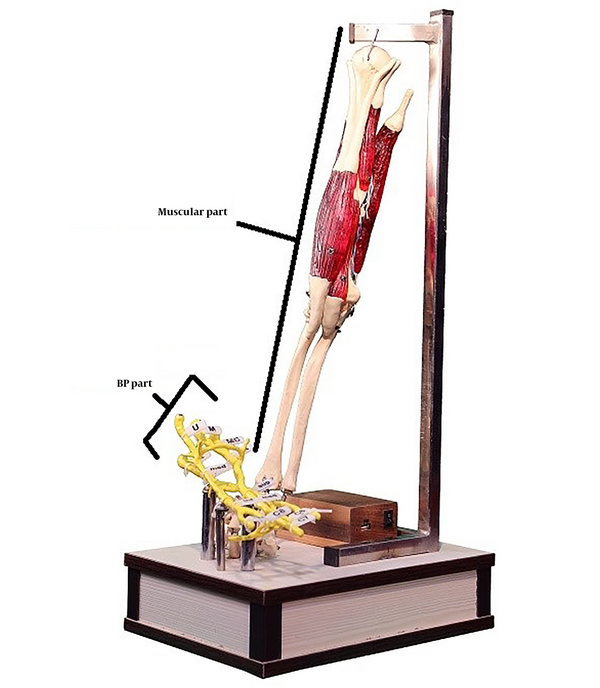
3.2.1. Brachial Plexus Component
The BP in the EM is a simulation moulage designed to resemble a larger-sized hand. It features a wired skeleton constructed from twisted wire covered with a twin dough material, which forms the structure of the BP. The twin dough used is durable and firm. To enhance realism, the BP is coated with yellow oil paint. The size of the BP is larger than that of a real moulage, making it easier for observation and learning purposes. At the end of the BP nerves, there are four small keys referred to as chassis. These keys are situated on the cutaneous, radial, median, and ulnar nerves. Each key is connected to two wires that are twisted around the wired skeleton of the BP and located within the twin dough structure. In other words, they are integrated into the BP’s structure and emerge from its center. These wires are then concealed and transferred to steel support bases, rendering them invisible, with only a small portion of the wires visible. The BP is mounted on three steel tube bases (Figure 2) (16).
The BP part of the EM. Roots (T1, C8, C7, C6, and C5), trunks (superior: Sup, medial: Med, and inferior: Inf), cords (medial: Med, posterior: Pos, and lateral: Lat), and branches (musculocutaneous: MC, radial: Rad, median: M, and ulnar: U).
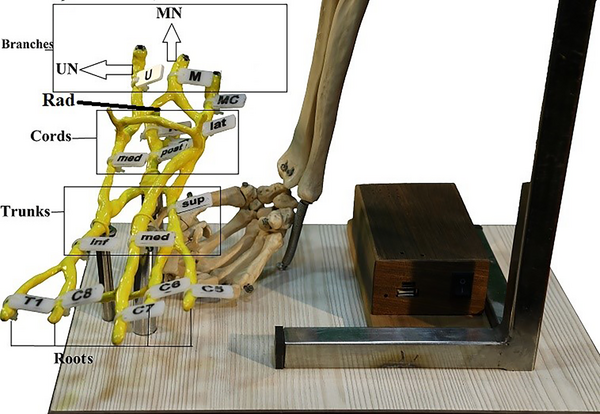
3.2.2. Muscle Component
On the opposite side of the electronic model, there is a polyvinyl chloride (PVC) skeletal system representing the right hand. This bone is connected to a steel base from above via a hook and from below with a spring, ensuring its upright position on the wooden box or main seat of the electronic model. The base, made of sturdy steel, features a hook-like shape to suspend the bone. It is vertically mounted on the wooden box using two welded screws underneath. The hand bone is accompanied by four arm muscles: Triceps, biceps, brachialis, and coracobrachialis. These muscles, made of epoxy resin, are colored red and capable of transmitting light. Epoxy resin is a highly resilient and durable material. Each muscle contains light-emitting diodes (LEDs), with multiple LEDs embedded within each muscle. The muscles have tendon endings at their beginning and end points, crafted from the same twin dough used for sculpting. The muscles and tendons are firmly connected to one another and to the bone using a strong twin glue, forming the skeletal structure of the arm muscles.
The LED wires and muscle keys are interconnected and ultimately terminate at the top of the bone with a plug (Figures 3 and 4) (17).
The muscular part of the EM. The muscles of the biceps, triceps, brachialis, and coracobrachialis can be observed next to the radius and ulna bones.
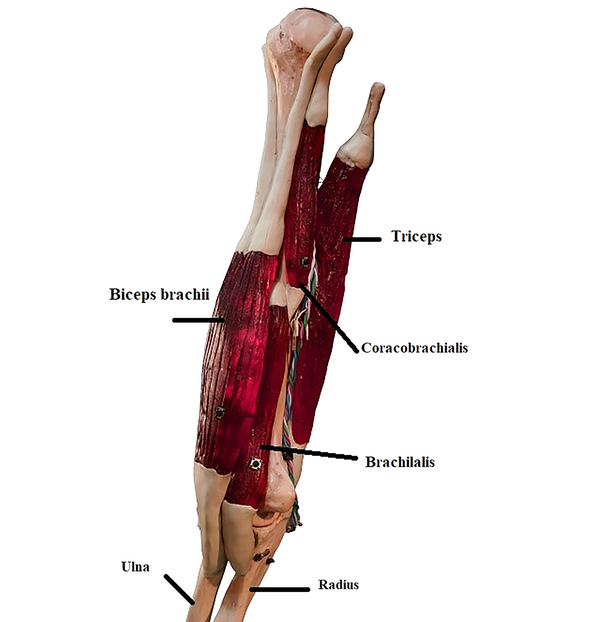
The diagram of the all parts of EM: (1) The main wooden box and the model’s main seat, (2) The BP part of the EM, (3) The muscular part of the EM, (4) The lateral plex box, (5) The small wooden box, (6) The steel base of the bony parts of the EM, (7) The steel base of the BP, (8) The hook, (9) The screw, (10) The mini USB charger port, (11) The USB charger port, (12) The on/off button, (13) The button over the musculocutaneous nerve, (14) The button over the radial nerve, (15) The button over the median nerve, (16) The button over the ulnar nerve, (17) The brachialis muscle, (18) The biceps muscle, (19) The triceps muscle, (20) The coracobrachialis muscle, (21) The wire, (22 and 23) The power plugs, (24) The wire, (25) The red rectangle containing the name of the triceps muscle, (26) The red rectangle containing the name of the biceps muscle, (27) The red rectangle containing the name of the brachialis muscle, (28) The red rectangle containing the name of the coracobrachialis muscle, (29) The yellow rectangle containing the name of the musculocutaneous nerve, (30) The yellow rectangle containing the name of the radial nerve, (31) The yellow rectangle containing the name of the median nerve, (32) The yellow rectangle containing the name of the ulnar nerve, (33) The button over the brachialis muscle, (34) The button over the biceps muscle, (35) The button over the triceps muscle, (36) The button over the coracobrachialis muscle, (37) The battery, (38) The charger board, (39) The sound player board, (40) The amplifier board.
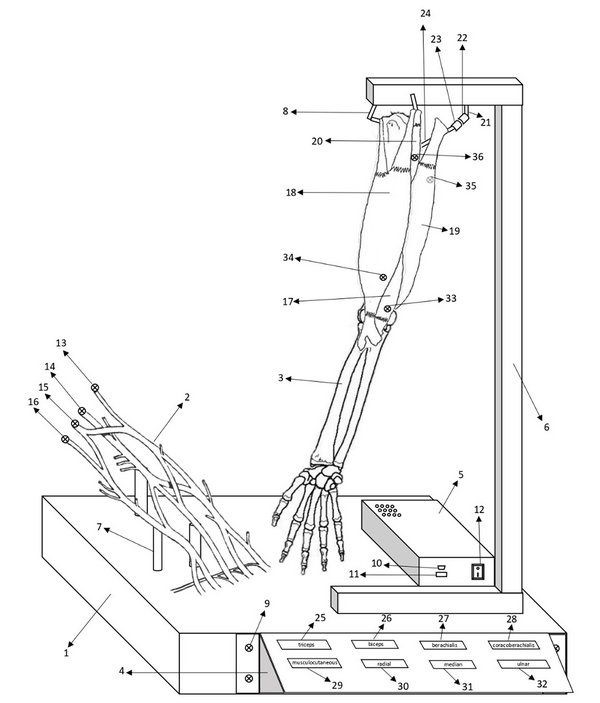
3.3. Evaluation of Performance, Importance, and Role of Electronic Moulage
3.3.1. Evaluation by Experts in Anatomy Sciences
After designing the electronic model, its performance and significance were evaluated by sharing a video file describing its structure and operation with experts in the field of anatomy sciences across several medical universities. The experts were provided with Appendix 1 in the Supplementary File, which contained indicators for assessment. Their opinions and ratings were collected and analyzed. The indicators in Appendix 1 in the Supplementary File included the following aspects: Practicality of the design for teaching the BP, potential for future upgrades to include other anatomical departments, ability to generate motivation, facilitation of learning the BP and its associated muscles, alignment with credible anatomy scientific sources, overall potential, and level of innovation compared to internal and external samples. A total of 30 anatomy experts from various medical sciences universities participated in the evaluation. They were surveyed and asked to provide scores ranging from 1 to 4 for each indicator. A higher score indicated a higher level of achievement in that particular indicator.
3.3.2. Evaluation by Students
3.3.2.1. Student Grouping
In this study, we utilized four independent, random, and homogeneous groups of undergraduate medical radiology students (80 students; 20/group) with an average age of 19.4 ± 1.6 years. The students in each group were admitted to the Bachelor of Radiology program in the 2019 - 2020 academic year. All groups were in their first semester of radiology and had not received any instruction on upper limb anatomy.
The grouping was done as follows (20 students/group):
(1) Students under training based on the lecture method (lecture-based education)
(2) Students under training based on the use of a digital method (KalbodNama anatomy training simulator digital-based education)
(3) Students under training with cadaver (cadaver-based education)
(4) Students under training with EM (EM-based education)
The purpose of this grouping was to compare the effect of EM on the training of the anterior and posterior compartments of the arm, in addition to on BP, before and after using EM and comparing to digital, lecture, and cadaver teaching methods.
3.3.2.2. Pre-test/Post-test Evaluation
Before the start of the teaching session, we administered a pre-test to all three groups, consisting of six standard questions related to the anatomy of the arm compartment and BP. The questions were homogeneous, and we asked them during a standard time of 6 minutes under the same conditions for three groups. After teaching the same topic, we asked the same six questions in a post-test (see Appendix 2 in the Supplementary File). We then compared the pre-and post-test results between the groups.
All participants were assessed before (pre-test) and immediately after the intervention (post-test) using the Questionnaire of Anatomy’s Knowledge.
The inclusion criteria for the present study were the interest in participating in the research and completing a course in the anatomy of BP Muscles. The exclusion criteria for participants included refusal to proceed with the research, failure to attend an educational session, and failure to complete the research instruments in the second phase of data collection. Informed consent was obtained from all participants. The subjects were assured that their personal information would be kept confidential and only general statistics and data would be published. This project was approved by the Ethics Committee of EDC of Torbat Heydarieh University of Medical Sciences, Torbat Heydarieh, Iran.
3.3.2.3. Survey Evaluation of Students
At the end of the teaching, a survey form was provided to the students, which included 11 questions related to motivational goals, performance, and the importance of the EM method (Appendix 3 in the Supplementary File). The opinions and ratings were collected and analyzed, with a score range of 1 - 5 (a higher score indicates a higher level of the indicator).
3.4. Statical Analysis
To determine the internal consistency of the questionnaire, Cronbach’s alpha coefficient was utilized, with an acceptable threshold of 0.85 considered for reliability. Validity was assessed using the Pearson correlation coefficient, with a minimum threshold of 0.4 considered acceptable for correlation. To compare the evaluations between anatomy science experts and students, the mean ± standard deviation was employed. Furthermore, to analyze the results of the pre-/post-test evaluations, the normality of the data was assessed using the Kolmogorov-Smirnov test (P > 0.05). The mean results were then compared between the groups using the two independent samples t-test in SPSS version 16 software, with a significance level of 0.05 (P < 0.05).
4. Results
4.1. Evaluation of Performance, Importance, and Role of Electronic Moulage
4.1.1. Evaluation by Experts in Anatomy Sciences
After analyzing the survey results, it was determined that anatomy science experts, on average, rated the performance and importance of this EM at 3.8 out of 4. The questionnaire exhibited good internal consistency with a Cronbach’s alpha coefficient of 0.92, indicating high reliability. The validity of the questionnaire was supported by a correlation coefficient of 0.65. Among the various indicators assessed, the practicality of the design for teaching received the lowest score of 3.8 (Table 1).
Results Related to the Evaluation of Performance Indicators and the Importance of Electronic Moulage from the Point of View of Experts in the Field of Anatomical Sciences (n = 30)
Evaluation Parameters of the EM/Scores | Mean ± SD |
---|---|
The practicality of the design in teaching the BP. | 3.8 ± 0.54 |
The ability to upgrade to more complete models (adding other compartments of the upper limb). | 4 ± 0 |
Creating motivation and interest in learning anatomy. | 4 ± 0 |
Helping to facilitate learning of the arm’s nervous system and its related muscles | 4 ± 0 |
The conformity of the electronic model with credible scientific anatomy resources | 4 ± 0 |
Level of innovation (in terms of having internal or external models) | 3.9 ± 0.24 |
Total mean | 3.8 ± 0.32 |
4.1.2. Evaluation by Students
Upon analyzing the pre-/post-test evaluations of radiology students in groups, it was observed that Cronbach’s alpha coefficient was 0.87, indicating good internal consistency. The correlation coefficient was observed to be 0.55, indicating a moderate level of validity. In the lecture-based education group, the mean pre-test score was 1.8 ± 0.2, and the post-test score was 2.5 ± 0.2. However, this difference was not statistically significant (P = 0.23). Similarly, in the digital-based education group, the mean pre-test score was 2.1 ± 0.3, and the post-test score was 3.1 ± 0.3, which was also not statistically significant (P = 0.086). Conversely, the cadaver-based education group showed a significant increase in the post-test score, compared to the pre-test score, with a mean pre-test score of 0.4 ± 4.2 and a mean post-test score of 0.3 ± 2.0 (P = 0.021). However, the post-test scores of the digital and lecture-based education groups did not show significant increases, compared to their respective pre-test scores.
In the EM-based education group, the mean pre-test score was 2.0 ± 0.3, and the post-test score was 5.4 ± 0.3, which was statistically significant (P = 0.003). Furthermore, comparing the mean post-test scores of the EM-based education group to the digital-based education group (P = 0.016) and the lecture-based education group (P = 0.009) revealed that the EM-based education group had significantly higher scores (Table 2). After evaluating the survey results of the EM-based education group, which exhibited a Cronbach’s alpha coefficient of 0.88 and a correlation coefficient of 0.60, it was determined that the students gave a mean score of 4.75 out of 5 for the performance and importance of this EM. Among the defined indicators, the teaching design index received the lowest score of 3.8 (Table 3).
Groups | Pre-test, Mean ± SD | Post-test, Mean ± SD |
---|---|---|
Lecture-based education | 1.8 ± 0.2 | 2.5 ± 0.2 |
Digital-based education | 2.1 ± 0.3 | 3.1 ± 0.3 |
Cadaver-based education | 2.0 ± 0.3 | 4.2 ± 0.4 |
EM-based education | 1.9 ± 0.2 | 5.4 ± 0.3 |
Results Related to the Evaluation of Students’ Opinions (n = 40/20 in Each Group)
Evaluation Parameters of the EM/Scores | EM-Based Education Group, Mean ± SD |
---|---|
Using moulage improved the quality of learning for me | 4.90 ± 0.29 |
Moulage increased my learning speed | 4.73 ± 0.36 |
Working with moulage was easy for me | 4.73 ± 0.36 |
The moulage was close to the real model | 5.0 ± 00.00 |
I am happy that I was able to work independently with moulage | 4.90 ± 0.11 |
I am satisfied that the moulage has light and sound | 4.00 ± 0.39 |
I am satisfied that the moulage shows the connection between nerve and muscle | 4.90 ± 0.26 |
Using moulage along with teacher’s teaching improves the quality of education | 4.90 ± 0.16 |
It is better to add this moulage to the moulage salon | 4.72 ± 0.21 |
How difficult was working with the model from a student’s point of view? | 4.72 ± 0.27 |
To what extent do you know the quality of the construction of the various components of the model, in addition to the clarity and loudness of the sound and the quality of the light? | 4.81 ± 0.19 |
5. Discussion
In this study, an EM was developed to specifically showcase the BP and its associated muscles, and its technical evaluation was conducted. This moulage addresses the limitations of other methods used in anatomy education, such as dissection, cadaveric education, plastination, traditional wax moulages and resin moulage, computer-based learning (CBL), live anatomy education (surgical anatomy), imaging techniques (e.g., computed tomography [CT] and magnetic resonance imaging [MRI]), and lecture-based education. It can be utilized independently or in conjunction with other methods to enhance the teaching and comprehension of anatomical structures. The effectiveness of anatomy education is typically assessed through short-term learning outcomes or long-term retention of information using various evaluation methods (18).
One of the persistent challenges in anatomy education has been the need for tangible representations of structures combined with an understanding of their functionality. Additionally, learning about adjacent structures aids surgeons and other medical professionals in obtaining a comprehensive understanding of superficial and deep structures at surgical sites. The oldest approach to teaching anatomy involving hands-on exploration of structures is through dissection and cadaver education. This method fosters in-depth and clinically relevant learning of anatomical topics, preparing students for patient-oriented settings. Currently, dissection and cadaver education constitute a significant component of practical anatomy units in most medical universities worldwide, including Iran (17). Research by Patel and Moxham in 2006 revealed that 69% of anatomists prefer incorporating cadaver-based teaching alongside lecture-based instruction (19). Similar studies conducted in 2011 and 2014 in medical schools also demonstrated the preference of both teachers and students for anatomy education through cadaver dissection (2, 20). Although there are varying opinions regarding the role of cadavers in the education of different medical disciplines today, some argue that cadaver dissection is unnecessary for non-medical fields and exclusively required for medical students. Another perspective suggests that cadavers should only be utilized in anatomy education for students in advanced study programs and residencies (21, 22).
However, it is important to note that these viewpoints are influenced by certain factors, including religious beliefs, concerns regarding the inhalation of toxic substances such as formalin gas, the high costs associated with procuring, fixing, and maintaining cadavers, and time constraints in cadaver-based education. In the present study, the EM demonstrated a significant improvement in learning outcomes compared to traditional dissection and lecture-based methods. Student feedback also indicated increased interest in learning anatomy when using this tool compared to other methods. Chytas et al. conducted a study showing that although virtual cadaver dissection is a useful method for teaching anatomy to medical students, it is significantly less effective than real cadaver dissection in terms of long-term learning. This is due to the inability to physically interact with structures and the lack of visibility of their real-life relationships (23). In contrast, the electronic dissection employed in this study overcomes these limitations by allowing students to physically touch anatomical structures and providing traceable performance feedback.
Leung et al. put forth the argument that cadaver-based anatomy education should be limited to surgeons, although alternative methods should be employed for other fields (22). Cadaver-based education predominantly aligns with traditional medical education and has become less practical in modern system-based anatomy education approaches, which have been implemented in Iran in recent years. This shift is due to the systemic nature of anatomy education and the move away from region-based anatomy instruction (24, 25). Furthermore, the limitations of cadaver-based education include the degradation of cadaver quality over time, loss of structures during dissection, and ethical considerations associated with cadaver use (26). In contrast, EM utilizes artificial intelligence, electronics, robotics, and sustainable material engineering to create a tangible, safe, and sustainable moulage for anatomy learning. In the present study, this method was employed in a group setting without generating any odor or undergoing changes in color and texture, and it can be reused multiple times.
The latest moulages, designed by Lim et al., utilize 3D printing and computer-aided design (CAD) systems connected to laser devices, incorporating data from MRI, CT, and ultrasound images (27). These moulages exhibit precise dimensions and accurately depict the location of minute structures within each tissue and organ, aiding in the comprehension of anatomical accuracy and depth in conjunction with cadaver-based instruction. However, it should be noted that this method alone cannot offer comprehensive education, as theoretical knowledge from books and atlases is still necessary (28). On the other hand, electronic dissection has the potential to overcome these limitations in the future, potentially revolutionizing anatomy education and enhancing the understanding of its concepts. In this regard, EM serves as a promising prototype for smart moulages, encouraging companies to invest in this field and create a new educational platform.
5.1. Conclusions
Anatomy education is faced with limitations when using various methods, prompting the exploration of interactive and complementary approaches to enhance the quality and effectiveness of learning and concept comprehension. However, challenges arise due to cost and time constraints in implementing these methods. Considering the significant impact of insufficient anatomy education, particularly in surgical fields, leading to medical errors, there is a pressing need to reduce these errors and save thousands of lives annually. In this regard, the utilization of advanced technology in designing smart (electronic) moulages holds great promise. The electronic dissection developed in this study serves as a prototype that can be further enhanced and has the potential to greatly improve anatomy education.
References
-
1.
Saeid N. [Effect of blended Learning on Students' Self-Determination and Academic Eagerness. New Educ Approach. 2019;14(2):67-86. Persian. https://doi.org/10.22108/nea.2020.117216.1372.
-
2.
Ellis H. Medico-legal Litigation and its Links with Surgical Anatomy. Surgery (Oxford). 2002;20(8):i-ii. https://doi.org/10.1383/surg.20.8.0.14518.
-
3.
Cahill DR, Leonard RJ, Marks SJ. Standards in health care and medical education. Clin Anat. 2000;13(2):150. [PubMed ID: 10679860]. https://doi.org/10.1002/(SICI)1098-2353(2000)13:2<150::AID-CA12>3.0.CO;2-V.
-
4.
G. B. D. Diseases Injuries Collaborators. Global burden of 369 diseases and injuries in 204 countries and territories, 1990-2019: a systematic analysis for the Global Burden of Disease Study 2019. Lancet. 2020;396(10258):1204-22. [PubMed ID: 33069326]. [PubMed Central ID: PMC7567026]. https://doi.org/10.1016/S0140-6736(20)30925-9.
-
5.
Kuo KH, Leo JM. Optical Versus Virtual Microscope for Medical Education: A Systematic Review. Anat Sci Educ. 2019;12(6):678-85. [PubMed ID: 30414261]. https://doi.org/10.1002/ase.1844.
-
6.
McBride JM, Drake RL. National survey on anatomical sciences in medical education. Anat Sci Educ. 2018;11(1):7-14. https://doi.org/10.1002/ase.1760.
-
7.
Kowalczyk KA, Majewski A. Analysis of surgical errors associated with anatomical variations clinically relevant in general surgery. Review of the literature. Transl Res Anat. 2021;23:100107. https://doi.org/10.1016/j.tria.2020.100107.
-
8.
Khot Z, Quinlan K, Norman GR, Wainman B. The relative effectiveness of computer-based and traditional resources for education in anatomy. Anat Sci Educ. 2013;6(4):211-5. [PubMed ID: 23509000]. https://doi.org/10.1002/ase.1355.
-
9.
Arraez-Aybar LA, Sanchez-Montesinos I, Mirapeix RM, Mompeo-Corredera B, Sanudo-Tejero JR. Relevance of human anatomy in daily clinical practice. Ann Anat. 2010;192(6):341-8. [PubMed ID: 20591641]. https://doi.org/10.1016/j.aanat.2010.05.002.
-
10.
Wilson AB, Ross C, Petty M, Williams JM, Thorp LE. Bridging the transfer gap: laboratory exercise combines clinical exposure and anatomy review. Med Educ. 2009;43(8):790-8. [PubMed ID: 19659493]. https://doi.org/10.1111/j.1365-2923.2009.03409.x.
-
11.
McMenamin PG. Body painting as a tool in clinical anatomy teaching. Anat Sci Educ. 2008;1(4):139-44. [PubMed ID: 19177400]. https://doi.org/10.1002/ase.32.
-
12.
Bockers A, Mayer C, Bockers TM. Does learning in clinical context in anatomical sciences improve examination results, learning motivation, or learning orientation? Anat Sci Educ. 2014;7(1):3-11. [PubMed ID: 23733503]. https://doi.org/10.1002/ase.1375.
-
13.
Stokes-Parish J, Duvivier R, Jolly B. Expert opinions on the authenticity of moulage in simulation: a Delphi study. Adv Simul (Lond). 2019;4:16. [PubMed ID: 31333880]. [PubMed Central ID: PMC6615296]. https://doi.org/10.1186/s41077-019-0103-z.
-
14.
Smith-Stoner M. Using moulage to enhance educational instruction. Nurse Educ. 2011;36(1):21-4. [PubMed ID: 21135679]. https://doi.org/10.1097/NNE.0b013e3182001e98.
-
15.
Petersen CD, Rush SC, Gallo I, Dalere B, Staak BP, Moore L, et al. Optimization of Simulation and Moulage in Military-Related Medical Training. J Spec Oper Med. 2017;17(3):74-80. [PubMed ID: 28910473]. https://doi.org/10.55460/X6BB-TZ0C.
-
16.
Makkink AW, Slabber H. For the students, by the students: Student perceptions of low cost medical moulage in a resource-constrained environment. Afr J Emerg Med. 2019;9(4):207-11. [PubMed ID: 31890486]. [PubMed Central ID: PMC6933254]. https://doi.org/10.1016/j.afjem.2019.08.003.
-
17.
Davis CR, Bates AS, Ellis H, Roberts AM. Human anatomy: let the students tell us how to teach. Anat Sci Educ. 2014;7(4):262-72. [PubMed ID: 24249485]. https://doi.org/10.1002/ase.1424.
-
18.
Singh V, Kharb P. A paradigm shift from teaching to learning gross anatomy: meta-analysis of implications for instructional methods. J Anat Soc India. 2013;62(1):84-9. https://doi.org/10.1016/s0003-2778(13)80019-6.
-
19.
Patel KM, Moxham BJ. Attitudes of professional anatomists to curricular change. Clin Anat. 2006;19(2):132-41. [PubMed ID: 16302246]. https://doi.org/10.1002/ca.20249.
-
20.
Bockers A, Jerg-Bretzke L, Lamp C, Brinkmann A, Traue HC, Bockers TM. The gross anatomy course: an analysis of its importance. Anat Sci Educ. 2010;3(1):3-11. [PubMed ID: 20058224]. https://doi.org/10.1002/ase.124.
-
21.
Bergman EM, van der Vleuten CP, Scherpbier AJ. Why don't they know enough about anatomy? A narrative review. Med Teach. 2011;33(5):403-9. [PubMed ID: 21355704]. https://doi.org/10.3109/0142159X.2010.536276.
-
22.
Leung KK, Lu KS, Huang TS, Hsieh BS. Anatomy instruction in medical schools: connecting the past and the future. Adv Health Sci Educ Theory Pract. 2006;11(2):209-15. [PubMed ID: 16729246]. https://doi.org/10.1007/s10459-005-1256-1.
-
23.
Chytas D, Salmas M, Noussios G, Paraskevas G, Protogerou V, Demesticha T, et al. Do virtual dissection tables add benefit to cadaver-based anatomy education? An evaluation. Morphologie. 2023;107(356):1-5. [PubMed ID: 35135673]. https://doi.org/10.1016/j.morpho.2022.01.002.
-
24.
McLachlan JC, Regan De Bere S. How we teach anatomy without cadavers. The Clinical Teacher. 2004;1(2):49-52. https://doi.org/10.1111/j.1743-498X.2004.00038.x.
-
25.
Vaccarezza M, Papa V. 3D printing: a valuable resource in human anatomy education. Anat Sci Int. 2015;90(1):64-5. [PubMed ID: 25287879]. https://doi.org/10.1007/s12565-014-0257-7.
-
26.
Thomas RG, John NW, Delieu JM. Augmented reality for anatomical education. J Vis Commun Med. 2010;33(1):6-15. [PubMed ID: 20297908]. https://doi.org/10.3109/17453050903557359.
-
27.
Lim KH, Loo ZY, Goldie SJ, Adams JW, McMenamin PG. Use of 3D printed models in medical education: A randomized control trial comparing 3D prints versus cadaveric materials for learning external cardiac anatomy. Anat Sci Educ. 2016;9(3):213-21. [PubMed ID: 26468636]. https://doi.org/10.1002/ase.1573.
-
28.
Azer SA, Eizenberg N. Do we need dissection in an integrated problem-based learning medical course? Perceptions of first- and second-year students. Surg Radiol Anat. 2007;29(2):173-80. [PubMed ID: 17318286]. https://doi.org/10.1007/s00276-007-0180-x.